ABSTRACT
Triptolide (TPL) is a diterpenoid isolated from the traditional Chinese medicine Tripterygium wilfordii. It has powerful antitumor, immunosuppressive and anti-inflammatory properties. Recent studies have shown that TPL can induce apoptosis of hematological tumor cells, inhibit their proliferation and survival, promote autophagy and ferroptosis, and enhance the efficacy of traditional chemotherapy and targeted therapies. Various molecules and signaling pathways, such as NF-κB, BCR-ABL, and Caspase, are involved in inducing apoptosis of leukemia cells. To solve the water solubility and toxic side effects of TPL, low-dose TPL (IC20) combined with chemotherapy drugs and various TPL derivatives have entered preclinical studies. This review discusses advances in molecular mechanism, the development and utilization of structural analogues of TPL in hematologic tumors in the past two decades, and clinical applications.
Introduction
Triptolide (TPL, PG 490, LDTT-2, C20H24O6, molecular weight 360.4) is a diterpenoid tricyclic oxide from the Chinese herbal medicine Tripterygium wilfordii, which was first described in 1972 [Citation1]. Previous studies have shown that TPL has a wide range of anti-inflammatory and immunosuppressive activities and has been used to treat autoimmune diseases [Citation2–4]. Many studies have shown that TPL has antitumor effects [Citation5–9]. However, most of these studies concentrated on solid tumors, and relatively few studies on hematological malignancies. This review summarizes the research progress of TPL in hematological malignancies and the molecular mechanisms involved.
1 The effect of TPL as a single drug
1.1 TPL induces apoptosis in hematological tumor cells
Apoptosis is the main antitumor mechanism of TPL. Multiple signaling pathways and mechanisms are involved in mediating the proapoptotic effect of TPL (). XIAP (X-linked inhibitor of apoptosis protein) is a natural intracellular caspase inhibitor and is highly expressed in a variety of leukemia cell lines and acute myelocytic leukemia (AML) blasts [Citation10, Citation11]. TPL can induce caspase-dependent apoptosis in several AML cell lines and AML blasts by reducing the expression of XIAP and MCL-1 [Citation12], and promoting cytochrome C release to upregulate caspase-dependent apoptotic pathways [Citation13, Citation14]. The p53 signaling pathway is also involved in this process [Citation15]. MDM2 is an intracellular negative regulator of p53. TPL induces AML cell apoptosis by reducing MDM2 expression to disrupt MDM2-p53 homeostasis [Citation16], thereby promoting p53 activation and upregulating the expression of the downstream death receptor DR5 [Citation17]. AML cells are relatively resistant to tumor necrosis factor α-related apoptosis-inducing ligand (TRAIL), which can be overcome by TPL by downregulating XIAP and activating the P53 signaling pathway [Citation18, Citation19]. Therefore, TPL combined with TRAIL may achieve a better effect in treating AML.
Figure 1. Single drug effects of TPL. Note MDM2: murine double minute 2; DR5: death receptor 5; XIAP: X-linked inhibitor of apoptosis protein; ROS: reactive oxygen species; JAK: Janus Kinase; STAT3/STAT5: signal transducers and activators of transduction; MAPK: mitogen-activated protein kinase; ROCK1: Rho-associated protein kinase1; MLC: Myosin light chain protein; NF-κB: nuclear factor kappa-B; PENT: Phosphatase and tensin homolog; Nrf2: NF-E2-related factor 2; LC3 (MAP1LC3): microtubule- associated proteins 1A and 1B. ↘: activation/up-regulation; −: suppression/down-regulation.
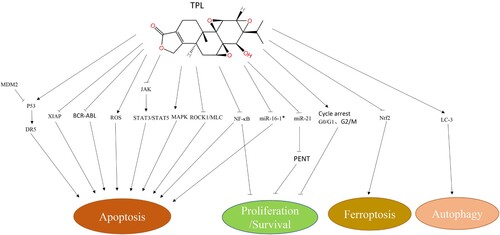
ROCK is a serine/threonine protein kinase composed of ROCK1 and ROCK2. Caspase-3 can cause the cleavage and activation of ROCK1 and lead to the phosphorylation of MLC and MYPT [Citation20, Citation21]. TPL can selectively induce leukemia cell apoptosis through the ROCK1/MLC pathway [Citation22, Citation23]. In mouse leukemia xenotransplantation models, TPL can inhibit tumor growth through ROCK1 activation and MLC phosphorylation [Citation24]. In addition, TPL can also inhibit the proliferation of AML cell lines by inhibiting the NF-κB pathway and activating the MAPK pathway to upregulate caspase-dependent apoptosis [Citation25]. In myeloproliferative disorder (MPD) cells, TPL can induce tumor cell apoptosis by inhibiting JAK transcription [Citation26]. In lymphocytic leukemia cell lines, TPL promotes apoptosis by inhibiting the NF-κB pathway and downregulating the expression of microRNA-16-1* [Citation27].
TPL has excellent antitumor effects in specific types of hematological tumors. In chronic myelogenous leukemia (CML) cell lines, TPL can inhibit the proliferation of CML cells and reduce the expression level of BCR-ABL [Citation28]. TPL can overcome imatinib resistance in T315I mutant cells by inhibiting BCR-ABL transcription and inducing apoptosis [Citation29]. In t (8; 21) AML, the development of the disease requires the formation of the AML1-ETO fusion gene and C-KIT mutation. TPL can inhibit these two processes and suppress the proliferation of tumor cells by inhibiting the JAK-STAT pathway and promoting apoptosis [Citation30].
In multiple myeloma (MM), TPL can inhibit the growth of bone marrow-derived mesenchymal stem cells (BMMSCs) from MM patients by decreasing IL-6, IL-1β and SCF mRNA expression [Citation56,Citation58,Citation59], and improve the bone marrow hematopoietic microenvironment by inhibiting the proliferation of MM cells [Citation59]. TPL inhibits Mcl-1 mRNA transcription and promotes its protein degradation through the ubiquitin-proteasome pathway [Citation87], and induces MM cell apoptosis by upregulating the expression of RIZ1 [Citation88] and protein tyrosine phosphatase 1 (SHP-1), and down-regulating antiapoptotic factors through the STAT3 pathway [Citation89]. In addition, TPL induces apoptosis in MM cells by blocking the aggregation of trimethylation of histone H3 lysine 4 (H3K4me3) in the promoter region of c-Myc and VEGFA [Citation90], and inhibiting the transcription factor NF-κB [Citation91].
In Burkitt lymphoma (BL), where the loss of SIRT3 is associated with high aggressiveness [Citation70], TPL promotes apoptosis by upregulating SIRT3 expression and activating the SIRT3/GSK-3β/Bax pathway. In addition, TPL inhibits tumor growth in mouse BL xenografts in a dose-dependent manner [Citation71]. In B lymphoma cells, TPL inhibits tumor cell growth by reducing the expression of human telomerase reverse transcriptase (hTERT) through the down-regulation of the expression levels of EBV-positive B lymphoma cell-specific protein 1 (SP1) and c-Myc transcription factor [Citation92]. TPL inhibits the proliferation of Raji cells, a B-cell non-Hodgkin's lymphoma (B-NHL) cell line, by blocking the SDF-1/CXCR4 axis [Citation93]. Low-dose TPL promotes apoptosis in B lymphoma cells by increasing phosphorylated histone H2AX levels, DNA fragmentation, and activation of caspase-3 [Citation94]. In T cell lymphoblastic lymphoma (T-LBL), TPL exerts an anti-tumor effect by increasing the expression of Caspase-3 and Bax, downregulating the expression of Bcl-2 [Citation95], and inhibiting invasion and EMT of Jurkat cells through the regulation of PI3 K/AKT/mTOR pathway [Citation95].
1.2 TPL inhibits the proliferation of hematological tumor cells by inducing cell cycle arrest
TPL can inhibit tumor cell proliferation through cell cycle arrest (). RPB1, the largest subunit of RNAPII, maintains genome integrity and promotes DNA repair through its interaction with Williams syndrome transcription factor (WSTF) (RPB1-WSTF) [Citation45]. TPL can induce apoptosis by causing DNA damage through the downregulation of WSTF-RPB1. In vitro and in vivo experiments showed that TPL inhibits the proliferation of AML cell lines and causes cell cycle arrest in G1 phase [Citation87]. Compared with solid tumors and other types of leukemia, AML cell lines are more sensitive to low-dose TPL-induced cell cycle arrest [Citation96]. Raman spectroscopy data show that TPL can inhibit the proliferation of leukemic T lymphocytes by arresting them in the G1 and S phases [Citation97].
In multiple myeloma (MM), RPMI8226 cells treated with TPL showed a significant increase in the proportion of cells in the G0/G1 phase compared with the control, suggesting that TPL treatment can induce G0/G1 phase cell cycle arrest in these cells [Citation31]. In contrast, in MM cell line U266, TPL arrests the cells in the G2/M phase [Citation32, Citation98]. TPL-induced cell cycle arrest is achieved by inhibiting the methylation of histone proteins in both cell types.
TPL can also induce cell cycle arrest in murine leukemia cells. TPL can also impair the survival ability of K562/A02 cells and overcome their resistance to daunorubicin (DOX) by inhibiting the expression of miR-21 and thus reducing the level of PENT [Citation32].
1.3 TPL promotes autophagy in hematological tumor cells
Studies have shown that autophagy plays an important role in tumorigenesis and treatment sensitivity [Citation33, Citation34], and there is an interaction between apoptosis and autophagy [Citation35–38]. It was shown that TPL induces both apoptosis and autophagy in WEHI-3 murine leukemia cells, evident by the characteristic changes in apoptotic proteins, the expression level of the autophagy marker LC-3, and the increased expression of autophagy molecules related to apoptosis, Atg5, Atg7, and Atg12 [Citation39]. The results indicate that the inhibitory effect of TPL on WEHI-3 may involve the interaction between apoptosis and autophagy, as shown in .
1.4 TPL promotes ferroptosis in hematological tumor cells
Recent studies have shown that TPL promotes ferroptosis in leukemic cells [Citation99]. TPL-induced ferroptosis in DOX-resistant leukemic cells is achieved by inhibiting the expression of Nrf2, resulting in increased ROS level, lipid oxidation, and decreased glutathione peroxidase 4 (GPX4). Notably, low-dose TPL treatment could resensitize DOX-resistant leukemic cells [Citation99], which is consistent with the inhibitory effect of TPL on Nrf2 target gene expression in lung cancer cells [Citation100].
2 Combined effects of TPL and chemotherapy drugs
2.1 TPL in combination with traditional chemotherapy drugs
DOX and idarubicin (IDA), both of which are anthracyclines, have a broad killing effect on various solid and hematologic tumors [Citation40, Citation41]. Cytosine arabinoside (Ara-c) is the most commonly used chemotherapy drug in the treatment of AML [Citation42, Citation43]. These three drugs are widely used in the treatment of hematologic tumors, and the ‘7 + 3’ regimen consisting of DOX/IDA and Ara-c has become the primary chemotherapy for AML [Citation44].
The combination of TPL with chemotherapeutic agents exerts antitumor effects through multiple mechanisms of action (). IDA/DOX combined with TPL can induce apoptosis of leukemia cells by downregulating HIF-1a and Nrf2 [Citation45, Citation46]. HIF-1a and Nrf2 are transcription factors. HIF-1a plays an important role in the adaptive response of tumor cells to a hypoxic environment, and it is also significant for the survival of leukemia stem cells [Citation47]. Nrf2 is an antioxidant transcription factor [Citation48–50]. The inhibition of HIF-1a expression can directly promote tumor cell apoptosis [Citation51], while Nrf2 reduction can also induce tumor cell apoptosis caused by the accumulation of ROS [Citation52]. TPL combined with chemotherapy drugs can induce leukemia cell apoptosis through DNA damage [Citation101].
Figure 2. Combination therapies. Note Dex: dexamethasone; PI3k: phosphatidylinositol-3-kinase; PI3k: phosphatidylinositol-3-kinase; YS110: humanized anti-CD26 monoclonal antibody; BTZ: bortezomib; Apg-1: HSPA4L, a member of the HSP110 family; VEN(ABT-199):Venetoclax; Bcl-2: B-cell lymphoma-2; Nutlin-3a: MDM2 inhibitor; TRAIL:TNF related apoptosis inducing ligand; IDA: Idarubicin; ADM(DOX): adriamycin(doxorubicin); IM:imatinib; HIF-1a: hypoxia inducible factor-1 alpha; Nrf2: NF-E2-related factor 2; ROS: reactive oxygen species; Ara-c: cytarabine; GO: gemtuzumab ozogamicin; FLT3: Fms-like tyrosine kinase; STAT5: signal transducers and activators of transduction 5. ↘: activation/up-regulation; −: suppression/down-regulation.
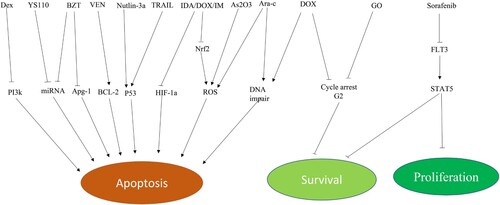
The cotreatment of TPL and DOX/Ara-c in NALM-6 cells can effectively inhibit DNA damage checkpoints 1 and 2, leading to intense DNA damage and tumor cell apoptosis [Citation53]. TPL can inhibit the expansion and survival of leukemia cells. DOX is a DNA-damaging drug that induces G2 phase arrest [Citation54, Citation55]. In the study by Bing Z. Carter et al., the treatment of OCI-AML3 cells with DOX or gemtuzumab ozogamicin (GO) alone produced significant G2 phase arrest after 48 h. However, when TPL and DOX/GO were combined, this cell arrest was abolished, leading to cell death [Citation12]. This indicates that TPL can induce cell death by breaking the DOX/GO-induced G2 phase arrest.
2.2 TPL in combination with targeted therapy
Targeted therapy is an important means of tumor treatment that has made rapid progress in recent years and is widely used in hematological malignancies [Citation57]. This effect can be improved when combined with TPL () [Citation13, Citation14, Citation60]. Venetoclax is a BCL-2 inhibitor approved by the FDA for treating chronic lymphocytic leukemia (CLL) and AML [Citation61]. The combination of TPL and Venetoclax can exert anti-leukemic effects by regulating the expression of Bcl-2 family proteins and activating the endogenous apoptosis pathway [Citation13]. Sorafenib is a multitargeted tyrosine kinase inhibitor that is mainly used for the treatment of AML with FLT3-ITD mutations [Citation62]. However, it is difficult to achieve durable remission, and long-term use is associated with drug resistance [Citation63, Citation64]. Low-dose TPL (IC20) combined with sorafenib can enhance the apoptosis rate of FLT3-ITD AML cells by reducing the expression of FLT3, STAT5, and P-STAT5, and the two drugs show a good synergistic effect [Citation65]. HSP-90 is overexpressed in AML cells and closely related to the proliferation of leukemia cells [Citation66, Citation67]. Inhibition of HSP-90 expression can degrade the BCR-ABL fusion protein and induce apoptosis in Ph+ Acute lymphoblastic leukemia (ALL) cells [Citation68]. BIIB021 is a selective inhibitor of HSP-90 that is used for the treatment of a wide range of tumors [Citation69]. In vitro experiments showed that TPL combined with BIIB021 could disrupt the dynamic balance between P53 and MDM2 in MOLT4 T-ALL cells, resulting in excessive activation of P53 and upregulation of the expression of Bak and Bim to induce tumor cell apoptosis [Citation16]. When combined with tyrosine kinase inhibitor imatinib, TPL can induce apoptosis in CML and acute promyelocytic leukemia (APL) cells by inhibiting the expression of HIF-1a and Nrf2 [Citation48]. TPL cooperates with Nutlin-3a to induce mitochondria-mediated apoptosis in vitro and in vivo. In addition, there is a synergistic effect in some cases of p53 deletion. Nutlin-3a upregulates the expression of p53 downstream targets PUMA and p21, while TPL reduces the mRNA levels of XIAP and Mcl-1. These two drugs have synergistic effects when used together [Citation102].
The synergistic effect of TPL and bortezomib (BTZ) on MM cells was achieved by reducing the expression of Apg-1 and HSF1 and increasing the expression of cleaved-caspase3 [Citation103]. In addition, the combination of TPL and BTZ can also overcome cell adhesion-mediated drug resistance [Citation103]. TPL combined with dexamethasone can promote apoptosis in MM cells by decreasing the expression of phospho-AKT and increasing Bad and P53 through the phosphatidylinositol 3-kinase (PI3 K) PI3k/Akt/NF-κB pathway [Citation104]. In addition, TPL can overcome dexamethasone resistance by increasing the expression of the glucocorticoid receptor (GR) through the down-regulation of miR142-5p and miR181a [Citation105].
The combined treatment of TPL and As₂O₃ can induce apoptosis in myelodysplastic syndromes (MDS) SKM-1 cells by increasing the expression of ROS, Bax, and caspase 3, and decreasing the expression of Bcl-2 [Citation106].
2.3 Targeted delivery of TPL using specific antibodies
CD26 is a type II glycoprotein that has been used as a poor prognostic factor, and CD26-positive cells have been found to be highly expressed in various hematologic tumors [Citation107–109]. Humanized anti-CD26 monoclonal antibody (YS110) has been coupled to TR1, a modified version of TPL at the sulfhydryl (SH) group, to produce an antibody–drug conjugate Y-TR1. Y-TR1 binds to CD26 on the cell surface and can cause cell death through immune-mediated cytotoxicity [Citation110, Citation111]. Y-TR1 significantly inhibits the proliferation of CD26-positive tumor cells without affecting CD26-negative cells. YS110 can be internalized to the nucleus to inhibit cell proliferation by suppressing the transcription of POLR2A, a subunit of RNA polymerase II. Y-TR1 inhibits cell proliferation by inhibiting general transcription factor IIH (TFIIH), which is required for the transcription of RNA polymerase II (Pol II) [Citation111, Citation112].
summarizes the important features of TPL combination therapy.
Table 1. The target and mechanism of action for combination therapy for treating hematological tumors.
3 Application of TPL structural analogues
Due to its poor water solubility and toxic side effects, the clinical application of TPL is limited to a certain extent. Therefore, a number of water-soluble structural TPL analogues with low toxicity have been developed [Citation72, Citation73]. At present, there are nearly ten types of TPL structural analogues (), including F60008, MRx102, and minnelide [Citation74]. Among them, F60008 (PG490-88) [Citation75], LLDT-246 [Citation76], LLDT-288 [Citation77], and LLDT-8 [Citation78, Citation79] are more commonly used in solid tumors. Minnelide, a prodrug of TPL, has entered phase 2 clinical trials in patients with advanced pancreatic cancer and has shown significant activity in preclinical models of pancreatic cancer [Citation80]. Minnelide has also shown good efficacy and tolerability in clinical trials of advanced gastrointestinal (GI) malignancies [Citation81].
A clinical trial of minnelide for leukemia is currently underway (phase 1, NCT03760523). This clinical trial will establish the maximum tolerated dose (MTD) and recommended phase 2 dose (RP2D) for minnelide monotherapy in patients with relapsed/refractory (R/R) AML who are ineligible for intensive chemotherapy.
Currently, the TPL structural analogues that have been used for the treatment of AML include MRx102 (18-benzoyloxy19-benzoylfuran triptolide) and minnelide. MRx102, which is soluble in water, is highly cytotoxic to human leukemia cells in vitro at nanomolar concentrations and reduces the viability of CD34+ AML mother cells. In xenotransplantation tumors with MV4-11 human AML cells, MRx102 (1.35 mg/kg/day) reduced tumor volume by 99%. It can inhibit tumor growth and metastasis by inducing cell apoptosis and inhibiting RNA transcription by downregulating the antiapoptotic proteins XIAP and Mcl2 and inhibiting the Wnt signaling pathway [Citation82–84].
Minnelide (14-O-phosphooxymethyl triptolide disodium salt), a water-soluble prodrug of TPL, is converted into the active form of TPL in vivo to exert its biological activity. Studies have shown that minnelide has an anti-leukemic effect in various leukemia models at a dose below the maximum tolerated dose (MTD) without affecting normal hematopoietic stem cells. Experiments in mice have shown that low-dose minnelide has an effect on both AML blasts and THP-1-expressing cells [Citation60]. Low-dose TPL(IC20) significantly reduces the colony formation ability of leukemia cell lines and the expression of stem cell markers [Citation13, Citation14, Citation53, Citation65, Citation80, Citation101], resulting in downregulation of c-Myc and leukemia cell cycle arrest at the G1/S phase [Citation85, Citation86].
Intriguingly, a recently developed water-soluble prodrug of TPL, TP-P1, is synthesized by linking TPL to cyclic amino acids through glycolic acid. Compared with minnelide, TPL was released more rapidly from TP-P1 into plasma. While maintaining its safety profile, 25 μg/kg TP-P1 could effectively inhibit tumor growth in an AML mouse model, while at a dose of 100 μg/kg, TP-P1 could eliminate tumor [Citation113]. TP-P1 can also significantly enhance the efficacy of FLT3 inhibitors [Citation113]. With the improved synthesis efficiency of TP-P1, the cost was greatly reduced [Citation113]. Therefore, TP-P1 has the potential to become a prodrug for treating AML.
4 Conclusions and prospects
An increasing number of studies have shown that TPL has a significant anti-AML effect. In response to the poor water solubility and toxic side effects of TPL, a number of TPL analogues have been developed. Combination therapies and targeted drug delivery systems further improved the efficacy. However, considering the complexity of the mechanism of action of TPL with different targets and signaling pathways, further studies are needed to understand how TPS exerts it effect in various types of hematological malignancies and different subtypes of AML. It is expected that these studies will provide a safe and effective new treatment strategy for personalized treatment of hematological malignancies.
Author contributions
T.X., Y.Z., S.G wrote the manuscript. SB.L conducted review and editing. All authors have read and agreed to the published version of the manuscript.
Disclosure statement
No potential conflict of interest was reported by the author(s).
Additional information
Funding
References
- Kupchan SM, et al. Triptolide and tripdiolide, novel antileukemic diterpenoid triepoxides from Tripterygium wilfordii. J Am Chem Soc. 1972 Oct 4;94(20):7194–7195.
- Datta R, Oki E, Endo K, et al. XIAP regulates DNA damage-induced apoptosis downstream of caspase-9 cleavage. J Biol Chem. 2000;275(41):31733–8.
- EfferthT LP, KonkimallaVS KB. From traditional Chinese medicine to rational cancer therapy. Trends Mol Med. 2007;13(8):353–361.
- Tang B, Zhu J, Zhang B, et al. Therapeutic potential of triptolide as an anti-inflammatory agent in dextran sulfate sodium-induced murine experimental colitis. Front Immunol. 2020 Nov 9;11:592084.
- Liang M, Fu J. Triptolide inhibits interferon-gamma-induced programmed death-1-ligand 1 surface expression in breast cancer cells. Cancer Lett. 2008;270(2):337–341.
- Philips P, Borja-Cacho D, Dudeja V, et al. Triptolide a potential therapeutic candidate for pancreatic cancer. J Am Coll Surg. 2007;205:S94.
- Zhou GX, Ding XL, Huang JF, et al. Apoptosis of human pancreatic cancer cells induced by Triptolide. World J Gastroenterol. 2008;14:1504–1509.
- Kang DW, Lee JY, Oh DH, et al. Triptolide-induced suppression of phospholipase D expression inhibits proliferation of MDA-MB-231 breast cancer cells. Exp Mol Med. 2009;41:678–685.
- Westfall SD, Nilsson EE, Skinner MK. Role of triptolide as an adjunct chemother-apy for ovarian cancer. Chemotherapy. 2008;54:67–76.
- Carter BZ, Kornblau SM, Tsao T, et al. Caspase- independent cell death inAML: caspase inhibition in vitro with pan-caspase inhibitors or in vivo by XIAP or Survivin does not affect cell survival or prognosis. Blood. 2003;102:4179–4186.
- Carter BZ, Milella M, Tsao T, et al. Regulation and targeting of antiapoptotic XIAP in acute myeloid leukemia. Leukemia. 2003;17:2081–2089.
- Carter BZ, Mak DH, Schober WD, et al. Triptolide induces caspase-dependent cell death mediated via the mitochondrial pathway in leukemic cells. Blood. 2006 Jul 15;108(2):630–637.
- Yuan-Fei Shi Y, Liu L, He L-L, et al. Combining triptolide with ABT-199 is effective against acute myeloid leukemia through reciprocal regulation of Bcl-2 family proteins and activation of the intrinsic apoptotic pathway. Cell Death Dis. 2020 Jul 22;11(7):555.
- Shi Y, Zhao H, Ye J, et al. Low-dose triptolide enhances antitumor effect of JQ1 on acute myeloid leukemia through inhibiting RNA polymerase II in vitro and in vivo. Mol Carcinog. 2020 Sep;59(9):1076–1087.
- Carter BZ, Mak DH, Schober WD, et al. Triptolide sensitizes AML cells to TRAIL-induced apoptosis via decrease of XIAP and p53-mediated increase of DR5. Blood. 2008;111:3742–3750.
- Li M, Zhang X, Zhou W-J, et al. Hsp90 inhibitor BIIB021 enhances triptolide-induced apoptosis of human T-cell acute lymphoblastic leukemia cells in vitro mainly by disrupting p53-MDM2 balance. Acta Pharmacol Sin. 2013;34:1545–1553.
- Zhou M, Gu L, Abshire T, et al. Incidence and prognostic significance of MDM2 on coprotein over expression in relapsed childhood acute lymphoblastic leukemia. Leukemia. 2000;14:61–67.
- Prives C. Signaling to p53: breaking the MDM2-p53 circuit. Cell. 1998;95:5–8.
- Wu X, Bayle JH, Olson D, et al. The p53-mdm-2 autoregulatory feedback loop. Genes Dev. 1993;7:1126–1132.
- Coleman ML, Sahai EA, Yeo M, et al. Membrane blebbing during apoptosis results from caspase-mediated activation of ROCK I. Nat Cell Biol. 2001;3:339–345.
- Sebbagh M, Renvoize C, Hamelin J, et al. Caspase-3-mediated cleavage of ROCK I induces MLC phosphorylation and apoptotic membrane blebbing. Nat Cell Biol. 2001;3:346–352.
- Parent N, Sane AT, Droin N, et al. Procaspase-2S inhibits procaspase-3 processing and activation, preventing ROCK-1-mediated apoptotic blebbing and body formation in human B lymphoma Namalwa cells. Apoptosis. 2005;10:313–322.
- Chang J, Xie M, Shah VR, et al. Activation of Rho-associated coiled-coil protein kinase 1 (ROCK-1) by caspase-3 cleavage plays an essential role in cardiac myocyte apoptosis. Proc Natl Acad Sci USA. 2006;103:14495–14500.
- Liu L, Li G, Li Q, et al. Triptolide induces apoptosis in human leukemia cells through caspase-3-mediated ROCK1 activation and MLC phosphorylation. Cell Death Dis. 2013 Dec 5;4(12):e941.
- Park S-W, Kim YI. Triptolide induces apoptosis of PMA-treated THP-1 cells through activation of caspases, inhibition of NF-κB and activation of MAPKs. Int J Oncol. 2013 Oct;43(4):1169–1175.
- Chen Q, Lu Z, Jin Y, et al. Triptolide inhibits Jak2 transcription and induces apoptosis in human myeloproliferative disorder cells bearing Jak2V617F through caspase-3-mediated cleavage of Mcl-1. Cancer Lett. 2010 May 28;291(2):246–255.
- Meng H-t, Zhu L, Ni W-m, et al. Triptolide inhibits the proliferation of cells from lymphocytic leukemic cell lines in association with downregulation of NF-κB activity and miR-16-1*. Acta Pharmacol Sin. 2011 Apr;32(4):503–511.
- Lou YJ, Jin J. Triptolide Down-regulates Bcr-abl Expression and Induces Apoptosis in Chronic Myelogenous Leukemia Cells. Leuk Lymphoma. 2004 Feb;45(2):373–376.
- Shi X, Jin Y, Cheng C, et al. Triptolide inhibits Bcr-Abl transcription and induces apoptosis in STI571-resistant chronic myelogenous leukemia cells harboring T315I mutation. Clin Cancer Res. 2009 Mar 1;15(5):1686–1697.
- Zhou G-S, Hu Z, Fang H-T, et al. Biologic activity of triptolide in t(8;21) acute myeloid leukemia cells. Leuk Res. 2011 Feb;35(2):214–218.
- Wen L, Chen Y, Zeng L-l, et al. Triptolide induces cell-cycle arrest and apoptosis of human multiple myeloma cells in vitro via altering expression of histone demethylase LSD1 and JMJD2B. Acta Pharmacol Sin. 2012 Jan;33(1):109–119.
- Zhao F, Chen Y, Li R, et al. Triptolide alters histone H3K9 and H3K27 methylation state and induces G0/G1 arrest and caspase-dependent apoptosis in multiple myeloma in vitro. Toxicology. 2010 Jan 12;267(1-3):70–79.
- Li H, Hui L, Xu W, et al. Triptolide modulates the sensitivity of K562/A02 cells to adriamycin by regulating miR-21 expression. Pharm Biol. 2012 Oct;50(10):1233–1240.
- White E, DiPaola RS. The double-edged sword of autophagy modulation in cancer. Clin Cancer Res. 2009 Sep 1;15(17):5308–5316.
- White EJ, Martin V, Liu JL, et al. Autophagy regulation in cancer development and therapy. Am J Cancer Res. 2011;1(3):362–372.
- Cho DH, Jo YK, Hwang JJ, et al. Caspase-mediated cleavage of ATG6/Beclin-1 links apoptosis to autophagy in HeLa cells. Cancer Lett. 2009 Feb 8;274(1):95–100.
- Zhu Y, Zhao L, Liu L, et al. Beclin 1 cleavage by caspase-3 inactivates autophagy and promotes apoptosis. Protein Cell. 2010 May;1(5):468–477.
- Kang R, Zeh HJ, Lotze MT, et al. The Beclin 1 network regulates autophagy and apoptosis. Cell Death Differ. 2011 Apr;18(4):571–580.
- Li H, Wang P, Sun Q, et al. Following cytochrome c release, autophagy is inhibited during chemotherapy-induced apoptosis by caspase 8-mediated cleavage of Beclin 1. Cancer Res. 2011 May 15;71(10):3625–3634.
- Chan S-F, Chen Y-Y, Lin J-J, et al. Triptolide induced cell death through apoptosis and autophagy in murine leukemia WEHI-3 cells in vitro and promoting immune responses in WEHI-3 generated leukemia mice in vivo. Environ Toxicol. 2017 Feb;32(2):550–568.
- Escherich G, Zimmermann M, Janka-Schaub G. Doxorubicin or daunorubicin given upfront in a therapeutic window are equally effective in children with newly diagnosed acute lymphoblastic leukemia. A randomized comparison in trial CoALL 07–03. Pediatr Blood Cancer. 2013 Feb;60(2):254–257.
- Lee HJ, Thompson JE, Wang ES, et al. Philadelphia chromosome-positive acute lymphoblastic leukemia: current treatment and future perspectives. Cancer. 2011 Apr 15;117(8):1583–1594.
- Luskin MR, Lee JW, Fernandez HF, et al. Benefit of high-dose daunorubicin in AML induction extends across cytogenetic and molecular groups. Blood. 2016;127:1551–1558.
- Fernandez HF, Sun Z, Yao X, et al. Anthracycline dose intensification in acute myeloid leukemia. N. Engl J Med. 2009;361:1249–1259.
- Murphy T, Yee KWL. Cytarabine and daunorubicin for the treatment of acute myeloid leukemia. Expert Opin Pharmacother. 2017 Nov;18(16):1765–1780.
- Ji JH, Min S, Chae S, et al. De novo phosphorylation of H2AX by WSTF regulates transcription-coupled homologous recombination repair. Nucleic Acids Res. 2019;47:6299–6314.
- Chen F, Liu Y, Wang S, et al. Triptolide, a Chinese herbal extract, enhances drug sensitivity of resistant myeloid leukemia cell lines through downregulation of HIF-1a and Nrf2. Pharmacogenomics. 2013 Aug;14(11):1305–1317.
- Matsunaga T, Imataki O, Torii E, et al. Elevated HIF-1a expression of acute myelogenous leukemia stem cells in the endosteal hypoxic zone may be a cause of minimal residual disease in bone marrow after chemotherapy. Leuk Res. 2012 Jun;36(6):e122–e124.
- Mak DH, Schober WD, Chen W, et al. Triptolide induces cell death independent of cellular responses to imatinib in blast crisis chronic myelogenous leukemia cells including quiescent CD34t primitive progenitor cells. Mol Cancer Ther. 2009;8:2509–2516.
- Wilczynski J, Duechler M, Czyz M. Targeting NF-aB and HIF-1 pathways for the treatment of cancer: part I. Arch. Immunol. Ther. Exp. (Warsz.). 2011;59(4):289–299.
- Wilczynski J, Duechler M, Czyz M. Targeting NF-kB and HIF-1 pathways for the treatment of cancer: part II. Arch. Immunol. Ther. Exp. (Warsz.). 2011;59(4):301–307.
- Marin-Hernandez A, Gallardo-Perez JC, Ralph SJ, et al. HIF-1a modulates energy metabolism in cancer cells by inducing over-expression of specific glycolytic isoforms. Mini Rev Med Chem. 2009 Aug;9(9):1084–1101.
- Tang X, Wang H, Fan L, et al. Luteolin inhibits Nrf2 leading to negative regulation of the Nrf2/ARE pathway and sensitization of human lung carcinoma A549 cells to therapeutic drugs. Free Radic Biol Med. 2011;50:1599–1609.
- Zhao H, Shi P, Deng M, et al. Low dose triptolide reverses chemoresistance in adult acute lymphoblastic leukemia cells via reactive oxygen species generation and DNA damage response disruption. Oncotarget. 2016 Dec 20;7(51):85515–85528.
- Blagosklonny MV, Robey R, Bates S, et al. Pre-treatment with DNA-damaging agents permits selective killing of checkpoint-deficient cells by microtubule-active drugs. J Clin Invest. 2000;105:533–539.
- Amico D, Barbui AM, Erba E, et al. Differential response of human acute myeloid leukemia cells to gemtuzumab ozogamicin in vitro: role of Chk1 and Chk2 phosphorylation and caspase 3. Blood. 2003;101:4589–4597.
- Liu Y, Sui JZ, Zhu LH, et al. [IL-6 Regulates the Chemosensitivity of Drug-Resistant Multiple Myeloma Cell Lines to Bortezomib through STAT3/Notch Signaling Pathway]. Zhongguo Shi Yan Xue Ye Xue Za Zhi. 2022;30(5):1474–1481.
- Srinivasarao M, Low PS. Ligand-Targeted Drug Delivery. Chem Rev. 2017 Oct 11;117(19):12133–12164.
- Piao X, Zhou J, Xue L. Triptolide decreases rheumatoid arthritis fibroblast-like synoviocyte proliferation, invasion, inflammation and presents a therapeutic effect in collagen-induced arthritis rats via inactivating lncRNA RP11-83J16.1 mediated URI1 and β-catenin signaling. Int Immunopharmacol. 2021;99:108010.
- Wu H, et al. Effects of triptolide on bone marrow-derived mesenchymal stem cells from patients with multiple myeloma. Exp Ther Med. 2019;17(5):3291–3298.
- Giri B, Gupta VK, Yaffe B, et al. Pre-clinical evaluation of minnelide as a therapy for acute myeloid leukemia. J Transl Med. 2019;17(163.
- Pan R, Hogdal LJ, Benito JM, et al. Selective BCL-2 inhibition by ABT-199 causes on-target cell death in acute myeloid leukemia. Cancer Discov. 2014 Mar;4(3):362–375.
- Burchert A. Maintenance therapy for FLT3-ITD-mutated acute myeloid leukemia. Haematologica. 2021 Mar 1;106(3):664–670.
- Röllig C, Serve H, Huttmann A, et al. Addition of sorafenib versus placebo to standard therapy in patients aged 60 years or younger with newly diagnosed acute myeloid leukaemia (SORAML): a multicentre, phase 2, randomised controlled trial. Lancet Oncol. 2015;16(16):1691–1699.
- Chen Y-B, Li S, Lane AA, et al. Phase I Trial of Maintenance Sorafenib after Allogeneic Hematopoietic Stem Cell Transplantation for Fms-like Tyrosine Kinase 3 Internal Tandem Duplication Acute Myeloid Leukemia. Biol Blood Marrow Transplant. 2014;20(12):2042–2048.
- Wang X, Ma L-M, Wang T, et al. [Effect of Low-Dose Triptolide and Sorafenib Alone and Their Combination on AML Cell Line MV411 and the Pathway of STAT5]. Zhongguo Shi Yan Xue Ye Xue Za Zhi. 2019 Aug;27(4):1088–1093.
- Lauten M, Beger C, Gerdes K, et al. Expression of heat-shock protein 90 in glucocorticoid-sensitive and -resistant childhood acute lymphoblastic leukaemia. Leukaemia. 2003;17:1551–1556.
- Hacıhanefioglu A, Gonullu E, Mehtap O, et al. Effect of heat shock protein-90 (HSP90) and vascular endothelial growth factor (VEGF) on survival in acute lymphoblastic leukemia: an immunohistochemical study. Med Oncol. 2011;28:846–851.
- Tong WG, Estrov Z, Wang Y, et al. The synthetic heat shock protein 90 (Hsp90) inhibitor EC141 induces degradation of Bcr-Abl p190 protein and apoptosis of Ph-positive acute lymphoblastic leukemia cells. Invest New Drugs. 2011;29:1206–1212.
- Lundgren K, Zhang H, Brekken J, et al. BIIB021, an orally available, fully synthetic small-molecule inhibitor of the heat shock protein Hsp90. Mol Cancer Ther. 2009;8:921–929.
- Yu W, Denu RA, Krautkramer KA, et al. Loss of SIRT3 Provides Growth Advantage for B Cell Malignancies. J Biol Chem. 2016 Feb 12;291(7):3268–3279.
- Kong T, et al. Triptolide induces mitochondria-mediated apoptosis of Burkitt, s lymphoma cell via deacetylation of GSK-3β by increased SIRT3 expression. Toxicol Appl Pharmacol. 2018;342:1–13.
- Li Z, et al. Design and synthesis of novel C14-hydroxyl substituted triptolide derivatives as potential selective antitumor agents. J Med Chem. 2009 Aug 27;52(16):5115–5123.
- Xu H, et al. Design, synthesis and anticancer activity evaluation of novel C14 heterocycle substituted epi-triptolide. Eur J Med Chem. 2014 Feb 12;73:46–55.
- Noel P, Von Hoff DD, Saluja AK, et al. Triptolide and Its Derivatives as Cancer Therapies. Trends Pharmacol Sci. 2019 May;40(5):327–341.
- Fidler JM, et al. PG490-88, a derivative of triptolide, causes tumor regression and sensitizes tumors to chemotherapy. Mol. Cancer Ther. 2003 Sep;2(9):855–862.
- Li M, et al. NF-kB signaling inhibition and anticancer activities of LLDT-246 on human colorectal cancer HCT-116 cells in vitro. Biomed Pharmacother. 2014 Jun;68(5):527–535.
- Xu H, et al. LLDT-288, a novel triptolide analogue exhibits potent antitumor activity in vitro and in vivo. Biomed Pharmacother. 2017 Sep;93:1004–1009.
- Tang W, Zuo J. Immunosuppressant discovery from Tripterygium wilfordii Hook f: the novel triptolide analog (5R)-5- hydroxytriptolide (LLDT-8). Acta Pharmacol Sin. 2012 Sep;33(9):1112–1118.
- Fu YF, et al. (5R)-5-Hydroxytriptolide (LLDT-8), a novel triptolide derivative, prevents experimental autoimmune encephalomyelitis via inhibiting T cell activation. J Neuroimmunol. 2006 Jun;175(1-2):142–151.
- Liu Y, et al. Low-dose triptolide in combination with idarubicin induces apoptosis in AML leukemic stem-like KG1a cell line by modulation of the intrinsic and extrinsic factors. Cell Death Dis. 2013 Dec 5;4(12):e948.
- Greeno E, Borazanci E, Gockerman J, et al. Abstract CT207: Phase I dose escalation and pharmokinetic study of 14-O-phosphonooxymethyltriptolide. Philadelphia: AACR; 2015.
- Carter BZ, et al. MRx102, a triptolide derivative, has potent antileukemic activity in vitro and in a murine model of AML. Leukemia. 2012 Mar;26(3):443–450.
- Reno TA, et al. The triptolide derivative MRx102 inhibits Wnt pathway activation and has potent anti-tumor effects in lung cancer. BMC Cancer. 2016 Jul 11;16:439.
- Fidler JM, et al. Preclinical antileukemic activity, toxi-cology, toxicokinetics and formulation development of triptolide derivative MRx102. Cancer Chemother Pharmacol. 2014 May;73(5):961–974.
- Wen SQ, Ma LM, Lu YJ, et al. Triptolide inhibits proliferation and induces apoptosis of imatinib resistant K562/G01 cells. Zhongguo Shi Yan Xue Ye Xue Za Zhi. 2013 Oct;21(5):1148–1152.
- Ma Y, Li Y, Huang M, et al. Triptolide inhibits T-cell acute lymphoblastic leukaemia by affecting aberrant epigenetic events in the Wnt signalling pathway. J Chemother. 2022 Jun 6: 1–10.
- Nakazato T, Sagawa M, Kizaki M. Triptolide induces apoptotic cell death of multiple myeloma cells via transcriptional repression of Mcl-1. Int J Oncol. 2014;44(4):1131–1138.
- Zhao F, Chen Y, Zeng L-l, et al. Effects of triptolide on RIZ1 expression, proliferation, and apoptosis in multiple myeloma U266 cells. Acta Pharmacol Sin. 2010;31(6):733–740.
- Kim J-H, Park B. Triptolide blocks the STAT3 signaling pathway through induction of protein tyrosine phosphatase SHP-1 in multiple myeloma cells. Int J Mol Med. 2017;40(5):1566–1572.
- Wen L, et al. Triptolide induces cell apoptosis by targeting H3K4me3 and downstream effector proteins in KM3 multiple myeloma cells. Curr Pharm Biotechnol. 2015;17(2):147–160.
- Yinjun L, et al. Triptolide inhibits transcription factor NF-kappaB and induces apoptosis of multiple myeloma cells. Leuk Res. 2005;29(1):99–105.
- Long C, Xu Q-B, Ding L, et al. Triptolide inhibits human telomerase reverse transcriptase by downregulating translation factors SP1 and c-Myc in Epstein-Barr virus-positive B lymphocytes. Oncol Lett. 2021;21(4):280.
- Zhang C, et al. Inhibitory effect of triptolide on lymph node metastasis in patients with non-Hodgkin lymphoma by regulating SDF-1/CXCR4 axis in vitro. Acta Pharmacol Sin. 2006;27(11):1438–1446.
- Guan J, et al. Triptolide induces DNA breaks, activates caspase-3-dependent apoptosis and sensitizes B-cell lymphoma to poly(ADP-ribose) polymerase 1 and phosphoinositide 3-kinase inhibitors. Oncol Lett. 2017;14(4):4965–4970.
- Huang Y, et al. Antitumor effect of triptolide in T-cell lymphoblastic lymphoma by inhibiting cell viability, invasion, and epithelial-mesenchymal transition via regulating the PI3K/AKT/mTOR pathway. Onco Targets Ther. 2018;12(11):769–779.
- Kang D, Liu Y, Song Y, et al. Triptolide shows high sensitivity and low toxicity against acute myeloid leukemia cell lines through inhibiting WSTF-RNAPII complex. Front Oncol. 2022 Feb 16;12:811850.
- Zhang D, et al. Raman spectrum reveals the cell cycle arrest of Triptolide-induced leukemic T-lymphocytes apoptosis. Spectrochim Acta A Mol Biomol Spectrosc. 2015;15(141):216–222.
- Zhao F, et al. Role of triptolide in cell proliferation, cell cycle arrest, apoptosis and histone methylation in multiple myeloma U266 cells. Eur J Pharmacol. 2010;646(1-3):1–11.
- Wu X, et al. Triptolide promotes ferroptosis by suppressing Nrf2 to overcome leukemia cell resistance to doxorubicin. Mol Med Rep. 2023;27(1):17.
- Nam LB, Choi WJ, Keum Y-S. Triptolide downregulates the expression of NRF2 target genes by increasing cytoplasmic localization of NRF2 in A549 cells. Front Pharmacol. 2021 Sep 8;12:680167.
- Shi P, Zha J, Feng J, et al. Low-dose triptolide enhanced activity of Idarubicin against acute myeloid leukemia stem-like cells via inhibiting DNA damage repair response. Stem Cell Rev Rep. 2021 Apr;17(2):616–627.
- Chen Q, et al. Therapeutic synergy of Triptolide and MDM2 inhibitor against acute myeloid leukemia through modulation of p53-dependent and-independent pathways. Exp Hematol Oncol. 2022;11(1):23.
- Zhu M, et al. Role of Apg-1 in HSF1 activation and bortezomib sensitivity in myeloma cells. Exp Hematol. 2020;81:50–59.
- Yang M, et al. Triptolide overcomes dexamethasone resistance and enhanced PS-341-induced apoptosis via PI3k/Akt/NF-kappaB pathways in human multiple myeloma cells. Int J Mol Med. 2008;22(4):489–496.
- Huang X, et al. Triptolide enhances the sensitivity of multiple myeloma cells to dexamethasone via microRNAs. Leuk Lymphoma. 2012;53(6):1188–1195.
- Hua H-Y, et al. Arsenic trioxide and triptolide synergistically induce apoptosis in the SKM 1 human myelodysplastic syndrome cell line. Mol Med Rep. 2016;14(5):4180–4186.
- Ebian HF, Abdelnabi AM, Abdelazem AS, et al. Peripheral blood CD26 positive leukemic stem cells as a possible diagnostic and prognostic marker in chronic myeloid leukemia. Leuk Res Rep. 2022 May 9;17:100321.
- Maitre E, Cornet E, Salaün V, et al. Immunophenotypic analysis of hairy cell leukemia (HCL) and hairy cell leukemia-like (HCL-like) disorders. Cancers (Basel). 2022 Feb 18;14(4):1050.
- Sharma P, Sachdeva MUS, Naseem S, et al. Identification of peripheral blood CD26+ leukemic stem cells has a potential role in the rapid diagnosis of chronic myeloid leukemia. Int J Lab Hematol. 2022 Jun;44(3):518–523.
- Angevin E, Isambert N, Trillet-Lenoir V, et al. First-in-human phase 1 of YS110, a monoclonal antibody directed against CD26 in advanced CD26-expressing cancers. Br J Cancer. 2017;116(9):1126–1134.
- Hayashi M, Madokoro H, Yamada K, et al. novel antibody-drug conjugate with anti-CD26 humanized monoclonal antibody and transcription factor IIH (TFIIH) inhibitor, triptolide, inhibits tumor growth via impairing mRNA synthesis. Cancers (Basel). 2019 Aug 8;11(8):1138.
- Hayashi M, Madokoro H, Yamada K, et al. A humanized anti-CD26 monoclonal antibody inhibits cell growth of malignant mesothelioma via retarded G2/M cell cycle transition. Cancer Cell Int. 2016 Apr 30;16:35.
- Kang D, et al. Discovery of a novel water-soluble, rapid-release triptolide prodrug with improved drug-like properties and high efficacy in human acute myeloid leukemia. Eur J Med Chem. 2022 Dec 5;243:114694.