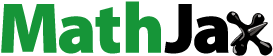
Abstract
A tropical wild fern, Diplazium esculentum, locally known as Pakis, was investigated in this study as a low-cost adsorbent for the removal of toxic crystal violet (CV) dye. The adsorbent–adsorbate system reached equilibrium in 180 min and data obtained from batch adsorption experiment were best fitted with the Sips isotherm model. High maximum adsorption capacity (qmax) of 350.86 mg/g compared to many other reported adsorbents revealed the potential of this fern as a low-cost, new adsorbent for the removal of CV. Adsorption mechanism followed the pseudo second-order kinetics. Another attractive feature is its ability to be regenerated and reused, especially when using the base treatment, while maintaining high adsorption of >96% even at the fifth consecutive cycles.
1. Introduction
Even though 70% of the earth surface is covered by water but only about 1% of it is drinkable. Because of the importance of drinking water in everyday life, it is vital to protect and conserve their sources from any harmful or dangerous contaminants. The main threat is pollution, which decreases the quality and quantity of water. The rise of the world population and industries worsens the quality and quantity of water [Citation1]. For instance, each year an alarming 5–10 billion tons of untreated industrial wastes are being dumped into rivers, oceans, and other waterways. As an example, textile industries themselves use a lot of synthetic dyes in their dyeing process to meet the ever-growing demand and this creates a lot of dye effluents. These dye effluents usually contain synthetic dyes and other chemicals, which are toxic, carcinogenic, mutagenic, or teratogenic to various microbiological and fish species. Despite the number of successful systems employing various physicochemical and biological decolourization processes, economical removal of colour from effluents still remains a major problem.
One of the synthetic dyes is crystal violet (CV), a green crystalline solid which dissolves in water and turns the solution into violet in colour, which is commonly used as a stain to classify bacteria. This dye is a threat to the aquatic environment as it is readily absorbed into fish tissue from water exposure and is reduced metabolically by fish, promoting tumour growth in some species of fish. Several studies had reported the carcinogenic and mutagenic effects of CV in rodents [Citation2–4]. Hence, removal of CV from wastewater and water sources is crucial and in this study, this dye was chosen as a model pollutive dye to be removed by the adsorption process.
Adsorption is one of the favourable techniques to remove pollutive dyes from wastewater, as this technique is simple and straightforward and it does not require highly skilled manpower. This technique is also cost-effective, and utilizes materials that are in abundance, of no value, and thrown away as wastes. Some of these materials include wastes from agriculture and industries, aquatic plants, clay, peat, and many others [Citation5–10].
In this study, we investigated the use of waste derived from a new adsorbent, namely Diplazium esculentum, which is a vegetable fern found in East to South Asia and Oceania. Diplazium is a genus of the cliff fern family with an approximate 400 known species. The habitat of these ferns can be found in lower forestry as well as in irrigation ditches and roadside gulley. D. esculentum is available all year long and it is locally known as “Pakis”, commonly sold in the markets in Brunei Darussalam. This plant is very popular and the local people usually stir-fried it in sambal prawn sauce, cooked it as a curry dish, or blanched and eaten it as a salad dish. The top part of Pakis and the young leaves are edible, while the stem, which is tasteless and not as tender, is thrown away as waste. In this study, we utilized the Pakis stem (PS) as a natural adsorbent for the removal of CV, which is a positively charged dye, from aqueous solution. To date, to the best of our knowledge, the study of PS as an adsorbent to remove any dyes or pollutants is scarcely. Hence this work will be the first of such study.
2. Materials and methods
Diplazium esculentum was randomly purchased from a local market in the Brunei-Muara District in Brunei Darussalam. The tender edible fronds were separated from the stems, which are usually harder compared to the fronds and are thrown away. These Diplazium esculentum stems (PS) were used as the adsorbent in this study. Before proceeding to the experiment, the PS samples were thoroughly washed with distilled water to remove dust and any other substances that may be present on the surface of the stems. The samples were then dried to a constant mass in an oven at 70°C before being blended using a blender and sieved using laboratory metal sieves to obtain particle size less than 350 µm in diameter. The samples were kept in a sealed plastic bag until further use.
The methods used in this study were referring to a paper by Lim et al. [Citation11] with few modifications. A stock solution of 1000 mg/L CV was prepared and diluted using serial dilution to obtain the required dye concentrations. Adsorption process was followed by measuring the absorbance of aqueous CV solutions, using the Shimadzu UV 1601 UV-Visible spectrophotometer (Japan) set at wavelength 590 nm, before and after mixing the PS adsorbent with the known concentration of CV solution at the desired time. The adsorption or removal efficiency of CV on the adsorbent was then calculated using Equation (1).
(1)
(1) where
and
is the concentration of CV before and after adsorption, respectively.
The effect of contact time was evaluated on the mixture of 0.050 g of PS adsorbent in 25.0 mL of 100 mg/L CV. The adsorption was monitored at every 30 min intervals from 0 to 240 min. The effect of pH on the adsorption efficiency was examined by modifying the pH of the 100 mg/L CV solution (ambient pH 5.05) to be in the range of 2–10, via the addition of 0.1 mol/L NaOH or 0.1 mol/L HCl. For ionic strength, the presence of NaCl was also tested along with KNO3 with both the salt concentrations ranging from 0 to 1.0 mol/L, with the use of 100 mg/L CV. Kinetics experiments were performed using two different CV dye concentrations (100 and 500 mg/L) at 3 min interval for 30 min followed by 10 min interval for another 30 min and finally at 30 min interval until its optimum shaking time. Regeneration study was carried out following method by Kooh et al. [Citation12].
The thermodynamics of the adsorption of CV on the PS adsorbent was evaluated by analysing the adsorption efficiency of the dye at different temperatures (298, 313, 323, 333 and 343 K) for the mixture of 300 mg/L CV with 0.050 g of PS adsorbent. Using the Gibbs free energy () equation,
, where R is gas constant and T is the temperature and the van’t Hoff plot of ln K against 1/T, the thermodynamics parameters were determined.
In this study, all the PS-CV mixtures were maintained using the ratio between the adsorbent (0.050 g) and dye (25.0 mL) of 1:500 (g:mL). As the adsorption experiments were carried out at high CV concentration, the uptake of the dye should increase with the concentration of dye, as it is normally observed [Citation13]. Therefore, this paper is mostly focused on the effects of CV concentration, pH, contact time, and temperature. All the experiments were carried out in duplicate unless otherwise stated and the data are presented as their average value.
3. Results and discussion
In order to evaluate the potential of PS as a possible low-cost adsorbent to remove CV from aqueous solution, the effects of several parameters on the adsorption capacity were investigated. One of the parameters was the contact time required for the PS-CV system to reach equilibrium. Here, a total of 4 h contact time was evaluated and the results indicated that 180 min were sufficient for the system to reach equilibrium, as shown in Figure . Therefore, all subsequent experiments were carried out using 180 min shaking time, except for kinetics study.
The pH dependence of CV removing efficiency is shown in Figure . The maximum efficiency of 100 mg/L CV occurs at pH 8 with 92.47% removal. In principle, this finding implies the mechanism governing the adsorption process. With pKa of CV being 9.4, the pH-dependent adsorption efficiency can be understood based on the protonation state of the functional groups on the surface of the adsorbent. In the basic environment, some functional groups such as carboxylic acid (pKa ≈5) are deprotonated, generating negative charge on the surface of the adsorbent and attract the cationic dyes. On contrary, at highly acidic pH, below pKa, protonation of the surface functional groups occurs, and the capability of the surface functional groups to bind the positively charged dyes is reduced due to electrostatic repulsion. Thus, we can consider that the increase in binding capacity of the adsorbent on CV with the increase in pH is due to the positive nature of the adsorbent and it becomes negatively charged at high pH. We may note that the removing efficiency tends to be constant with a variation being less than 2.0% in the medium pH within the range of 3–10. This indicates that the medium at pH 3 already exceeds the pKa of the surface functional groups, therefore the effect of pH is no more observed at higher pH. The different types of functional groups and their concentrations on the adsorbent surface may also be the reason for the different effects of pH on the CV. Overall, the pH-dependent adsorption efficiency indicated that the adsorption mechanism of the positively charged CV onto PS adsorbent is chemisorption, where the adsorption is controlled by the chemical or electrostatic interaction between CV and the functional groups on the surface of the adsorbent.
In comparison, other reported adsorbents such as bitter gourd waste showed removal efficiency of CV to be less than 30% at pH 2 [Citation14]. The concentration of CV in the solution is also the important feature in the removing efficiency. For instance, a very recent report on the use of water hyacinth as adsorbent showed a drastic decrease by a factor of more than 40% in the adsorption of CV when the dye concentration was increased from 100 to 500 mg/L [Citation15]. Our study conducted at 100 mg/L CV concentration showed that PS was able to maintain a high removing efficiency of more than 75%. This finding suggests the potential of PS to adsorb CV efficiently in aqueous solution, and thus to remove CV in real-life application of wastewater treatment. In such an application, the adsorbent with constant removal efficiency at various wastewater pH is more advantageous.
It is noteworthy that wastewater may contain several materials and salts. The presence of the interfering salts may affect the electrostatic forces between adsorbent and dyes and this could in turn affect the removal of the dyes. In a system where there are electrostatic forces of attraction between the adsorbent and adsorbate, the high salt concentration may cause a decrease in adsorption. The converse is true if there are repulsive forces between adsorbent and adsorbate. In this latter case, the removal of adsorbates increases with salt concentration. To clarify this issue, in this study, the effect of two different salts (NaCl and KNO3) on the adsorption process was evaluated. Figure shows that in the absence of salt, the CV removing efficiency was more than 90%, and in the presence of salt, the removing efficiency also remained at above 90%. This means that the presence of salt in the wastewater does not affect the CV’s removing efficiency, indicating the stability of PS as an adsorbent. This finding is again an advantage for a real application, and thus PS is a good alternative for the removal of toxic dyes such as CV from wastewater. This is unlike many adsorbents which showed a drastic reduction in the removal of dyes as salt concentration increases. Example includes bitter gourd waste which showed a reduction of ∼ 70% at 1 mol/L NaCl [Citation14].
Adsorption isotherm of CV by PS was evaluated. Diversified isotherm models with two parameters (Langmuir, Freundlich, Dubinin-Radushkevic (D–R), Temkin) and three parameters (Sips and Redlich–Peterson (R-P)) were chosen in an attempt to describe the adsorption process of CV onto PS. Simulation plots in Figure ruled out the Langmuir model (R2 < 0.22) which is based on monolayer adsorption onto the homogeneous surface as well as the Freundlich model, a multi-layer adsorption onto the heterogeneous surface, since both greatly deviated from the experimental data. By ruling out the Langmuir isotherm model, the maximum adsorption capacity (qmax) obviously should be estimated from different isotherm models. Similarly, we also can rule out D-R model. Although its R2 is higher than 0.98, the overall error values were the highest among all the six isotherm models, as gathered in Table . Despite the high R2 values exhibited by both the Temkin and R-P isotherm models, their large error values are indicative that they are not suitable models for the adsorption of CV onto PS. Hence, out of the six isotherm models used, the Sips model is the best fit model as it gave the overall lowest error values and good R2 value (more than 0.96). In addition, its simulation plot showed close proximity to experimental data. We recall that the Sips model is sometimes known as the Langmuir-Freundlich model, where it tends toward the Langmuir isotherm model at high concentrations and adopts the Freundlich model at low concentrations. Since the Sips model provides the maximum adsorption capacity, thus based on this particular isotherm model, the qmax for the adsorption of CV by PS was estimated to be 350.86 mg/g, higher than many reported adsorbents, including those that are chemically modified or synthesized, as presented in Table in detail.
Table 1. Correlation coefficient (R2) and error values given by the theoretical fitting of different isotherm models on the adsorption isotherm data.
Table 2. Maximum adsorption capacity (qmax) of CV by PS and other selected biosorbents.
Thermodynamics of the adsorption process were investigated by evaluating the adsorption of CV onto PS at different temperatures from 298 to 343 K. From the van Hoff’s plot of ln K versus 1/T (data not shown), we found that the adsorption was an endothermic process with the enthalpy (ΔH°) being 5.21 kJ/mol. The qmax values at 298, 313, 323, 333 and 343 K, based on the Langmuir isotherm model, showed only a small incremental uptake with an increase in temperature and were found to be 144.2, 145.1 mg/g, 146.0, 146.1 mg/L and 149.3 mg/g, respectively. An increase in negativity in the Gibbs free energy (ΔG°) with temperature suggests a spontaneous adsorption process. The entropy (ΔS°) of 40.08 J/mol K indicates an increase in the randomness of the adsorbent–adsorbate system.
Considering that the dyes arrive onto the surface of adsorbents faster than they can diffuse into the solid, the accumulation of the dyes at the surface creates a pseudo-equilibrium. Most of the adsorption kinetics can be explained by two simplified kinetic models; namely pseudo first order and pseudo second order.
Theoretically, adsorption is considered to be first-order in adsorption capacity. This model only predicts the behaviour over the observation supporting the validity and is in agreement with chemisorption being the rate-limiting step. The pseudo first order can be mathematically explained by Equation (2).
(2)
(2) where qe (mmol g−1) is the amount of dye adsorbed at equilibrium, qt (mmol g−1) is the amount of dye adsorbed at time t (min) and k1 (min−1) is the first order reaction rate constant. The constant k1 and qe can be calculated from the slope and ordinate-intercept of the linear plot of log (qe − qt) against t. On the other hand, the pseudo second order describes that the rate-limiting step could be due to the chemisorption process between the adsorbent and adsorbate that involved the exchange of electrons. The pseudo second order is mathematically given by Equation (3).
(3)
(3) where k2 is the second-order reaction rate constant (g/mmol min). Thus, in this study, adsorption kinetics of CV onto PS were also investigated in detail by using the two kinetics models. The linear plots of both models were shown in Figure (A,B). From the linear plots of the two models on the adsorption kinetic data, we can specify the mechanism of the adsorption process. The selection was made based on two criteria, i.e. the correlation coefficient (R2) and the comparison between the theoretical and experimental adsorption capacities (qcal and qexp, respectively).
Figure 5. Plotted kinetic models of (A) pseudo first order and (B) pseudo second order based on the experimental data obtained, using 100 mg/L (

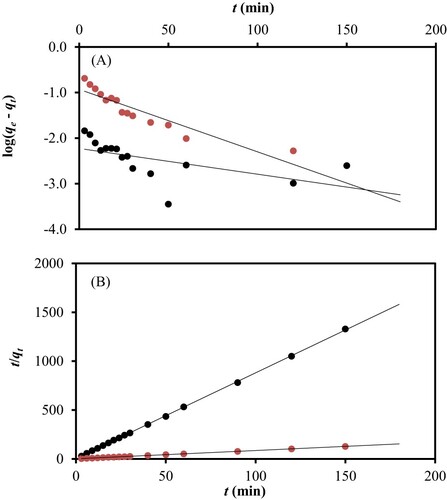
From Table , the correlation coefficients (R2) of pseudo first-order for 100 and 500 mg/L of CV were 0.3307 and 0.8429, respectively, whereas the linear plots of the experimental data using pseudo second-order model both showed unity. This shows that PS fits better with the pseudo second-order kinetics and this is further confirmed by the closeness of the qcal and experimental qexp adsorption capacities obtained from pseudo second-order.
Table 3. Parameters of kinetics models.
In regeneration study, PS was first loaded with CV dye and the percentage removal for CV dye was 98.9%. The spent PS was then subjected to various treatment methods over 5 consecutive adsorption–desorption cycles. From Figure , there was no noticeable difference in percentage removal using distilled water either by shaking the dye loaded sample at 250 rpm for 2 h or by quick rinsing of the spent adsorbent, other than it slowly decreased in effectiveness with each progressive cycle. From the various regeneration methods, base treatment with 0.1 M NaOH was the best where the spent adsorbent was able to achieve above 90% removal of CV dye throughout the five cycles. All the other desorbing methods slowly decrease in effectiveness over the five cycles. This could be due to the removal of surface fats and wax as a result of base treatment, thereby exposing surface functional groups for better adsorption with the dye molecules. Another possible reason could be due to deprotonation which can take place under basic condition thereby making the surface more negatively charged and thus attracts positively charged cationic dye. PS also demonstrated that it was still able to adsorb more than 60% CV dye in the fifth cycle, as shown by the control where no treatment was applied to the spent PS. Hence, this study shows the potential of utilizing PS as an effective low-cost adsorbent as it can be regenerated and reused.
Figure 6. Regeneration of spent PS for five cycles with various desorbing agents such as acid (orange), base (grey) and water with different methods of by shaking (yellow) and rinsing (blue) along with control (green).
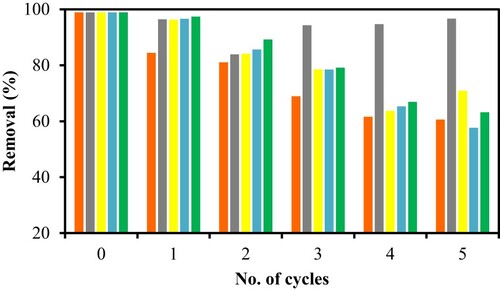
The characterizations on the adsorption of CV onto PS were evaluated by FTIR and SEM. FTIR indicates the presence of the functional groups on the surface of the adsorbent and the involvement of the functional groups in the adsorbent–adsorbate interactions. On the other hand, SEM may show a distinct difference on the surface of the PS before and after the adsorption process.
FTIR results (Figure ) revealed a broad peak at 3399 cm−1, indicating the presence of hydroxyl group (OH) of PS. The vibrational band of alkyl (CH) and C = C group appears at 2918 and 1658 cm−1, respectively. The vibrational spectrum of PS containing CV showed shifts on the hydroxyl and alkyl groups, while C = C peak can be observed at the same position. This means that hydroxyl and alkyl groups may take part in the adsorbent–adsorbate interaction. Upon the adsorption, several new peaks at 1587 cm−1 (C = C stretch of benzene ring), 1366 cm−1 (C–N stretch of aromatic tertiary amine) and 1170 cm−1 (C–N vibration) which could be attributed to their presence in the structure of CV were also observed. This further confirms the adsorption of CV molecules by PS.
As shown in Figure , the surface of PS is rough, allowing the dye molecules to be easily attached to the surface of the PS particles. Morphological difference of the surface of PS particle could be clearly seen, where the surface of PS after adsorption becomes smoother.
4. Conclusion
Pakis stem (PS) has shown its potential as a new low-cost adsorbent, in particular toward organic dyes. The ability of PS to work in a wide range of pH and resilient to the presence of salts shows the worthiness in using PS to be applied as an adsorbent in real wastewater. In addition, the high adsorption capacity of 351 mg/g was obtained in this study and it was far superior in comparison with most of the reported adsorbents used in removing CV in simulated wastewater.
Disclosure statement
No potential conflict of interest was reported by the author(s).
Additional information
Funding
References
- Mackuľak T, Bodík I, Smolinská M, et al. Automotive industry wastewater treatment by mixture of enzymes. Monatshefte für Chemie-Chemical Monthly. 2016;147(1):159–164. doi: 10.1007/s00706-015-1579-4
- Mani S, Bharagava RN. Exposure to crystal violet, its toxic, genotoxic and carcinogenic effects on environment and its degradation and detoxification for environmental safety. Rev Environ Contam Toxicol. 2016;237:71–104.
- Littlefield NA, Blackwell B-N, Hewitt CC, et al. Chronic toxicity and carcinogenicity studies of gentian violet in mice. Fundam Appl Toxicol. 1985;5(5):902–912. doi: 10.1016/0272-0590(85)90172-1
- Littlefield NA, Gaylor DW, Blackwell B-N, et al. Chronic toxicity/carcinogenicity studies of gentian violet in Fischer 344 rats: two-generation exposure. Food Chem Toxicol. 1989;27(4):239–247. doi: 10.1016/0278-6915(89)90162-2
- Ehsan A, Bhatti HN, Iqbal M, et al. Native, acidic pre-treated and composite clay efficiency for the adsorption of dicationic dye in aqueous medium. Water Sci Technol. 2017;75(4):753–764. doi: 10.2166/wst.2016.435
- Cherifi H, Djezar S, Korichi A, et al. Biosorption of methylene blue by activated sludge. Desalin Water Treat. 2016;57(48–49):22842–22851. doi: 10.1080/19443994.2016.1144692
- Zare MA, Husain SW, Tehrani MS, et al. Pentaazatetraethylene supported polyacrylamide (PAA-N5) as a novel adsorbent for the efficient removal of industrial dyes from aqueous solutions: adsorption isotherms and kinetics. Monatshefte für Chemie-Chemical Monthly. 2017;148(2):191–197. doi: 10.1007/s00706-016-1806-7
- Caponi N, Collazzo GC, Jahn SL, et al. Use of Brazilian Kaolin as a potential low-cost adsorbent for the removal of malachite green from colored effluents. Mater Res. 2017. doi: 10.1590/1980-5373-mr-2016-0673.
- Manna S, Roy D, Saha P, et al. Rapid methylene blue adsorption using modified lignocellulosic materials. Process Saf Environ Prot. 2017;107:346–356. doi: 10.1016/j.psep.2017.03.008
- Liu S, Wang H, Guan R, et al. Dye adsorption kinetics of organoamine-templated hollow silica microspheres with different porous structures. Monatshefte für Chemie-Chemical Monthly. 2017;148(7):1171–1176. doi: 10.1007/s00706-017-2016-7
- Lim LBL, Priyantha N, Zaidi NAHM. A superb modified new adsorbent, Artocarpus odoratissimus leaves, for removal of cationic methyl violet 2B dye. Environ Earth Sci. 2016;75(16):1179. doi: 10.1007/s12665-016-5969-7
- Kooh MRR, Dahri MK, Lim LBL. Jackfruit seed as a sustainable adsorbent for the removal of Rhodamine B dye. J Environ Biotechnol Res. 2016;4(1):7–16.
- Muhammad A, Shah AHA, Bilal S. Comparative study of the adsorption of acid blue 40 on polyaniline, magnetic oxide and their composites: synthesis, characterization and application. Materials (Basel). 2019;12:2854–2876. doi: 10.3390/ma12182854
- Lim LBL, Priyantha N, Mek KJ, et al. Potential use of Momordica charantia (bitter gourd) waste as a low-cost adsorbent to remove toxic crystal violet dye. Desalin Water Treat. 2017;82:121–130. doi: 10.5004/dwt.2017.21020
- Kulkarni MR, Revanth T, Acharya A, et al. Removal of crystal violet dye from aqueous solution using water hyacinth: equilibrium, kinetics and thermodynamics study. Res Eff Technol. 2017;3(1):71–77.
- Lim LBL, Priyantha N, Zehra T, et al. Adsorption of crystal violet dye from aqueous solution onto chemically treated Artocarpus odoratissimus skin: equilibrium, thermodynamics, and kinetics studies. Desalin Water Treat. 2016;57(22):10246–10260. doi: 10.1080/19443994.2015.1033474
- Dahri MK, Kooh MRR, Lim LBL. Artocarpus odoratissimus (Tarap) core as an adsorbent for the removal of crystal violet dye from aqueous solution. J Mater Environ Sci. 2017;8(10):3706–3717.
- Lim LBL, Priyantha N, Cheng HH, et al. Adsorption characteristics of Artocarpus odoratissimus leaf toward removal of toxic crystal violet dye: isotherm, thermodynamics and regeneration studies. J Environ Biotechnol Res. 2016;4(1):32–40.
- Lim LBL, Priyantha N, Cheng HH, et al. Parkia speciosa (Petai) pod as a potential low-cost adsorbent for the removal of toxic crystal violet dye. Sci Bruneiana. 2016;15:99.
- Chieng HI, Lim LBL, Priyantha N, et al. Sorption characteristics of peat of Brunei Darussalam III: equilibrium and kinetics studies on adsorption of crystal violet (CV). Int J Earth Sci Eng. 2013;6(4):791–801.
- Wang Y, López-Valdivieso A, Zhang T, et al. Preparation of microscale zero-valent iron-fly ash-bentonite composite and evaluation of its adsorption performance of crystal violet and methylene blue dyes. Environ Sci Pollut Res. 2017;24(24):20050–20062. doi: 10.1007/s11356-017-9426-2
- Miyah Y, Lahrichi A, Idrissi M, et al. Assessment of adsorption kinetics for removal potential of crystal violet dye from aqueous solutions using Moroccan pyrophyllite. J Assoc Arab Univ Basic Appl Sci. 2017;23:20–28.
- Muthukumaran C, Sivakumar VM, Thirumarimurugan M. Adsorption isotherms and kinetic studies of crystal violet dye removal from aqueous solution using surfactant modified magnetic nanoadsorbent. J Taiwan Inst Chem Eng. 2016;63:354–362. doi: 10.1016/j.jtice.2016.03.034
- Chieng HY, Lim LBL, Priyantha N. Enhancement of crystal violet dye adsorption on artocarpus camansi peel through sodium hydroxide treatment. Desalin Water Treat. 2017;58:320–331. doi: 10.5004/dwt.2017.0326
- Jerold M, Vasantharaj K, Joseph D, et al. Fabrication of hybrid biosorbent nanoscale zero-valent iron-Sargassum swartzii biocomposite for the removal of crystal violet from aqueous solution. Int J Phytoremediation. 2017;19(3):214–224. doi: 10.1080/15226514.2016.1207607