ABSTRACT
The use of disinfectants has drastically increased in response to the growing public awareness of risks of microbial infection. However, this practice has a negative impact on the environment as it results in dumping large volumes of antimicrobial and microbicidal compounds in aquatic and terrestrial ecosystems. Chloroxylenol (PCMX) is a phenolic compound that poses public health and environmental risks art high concentrations. Here, a novel disinfectant was proposed to increase the lethality of PCMX by adding copper (Cu) to it. However, it was necessary to demonstrate that this mixture is fungicidal and not merely fungistatic, and that the addition of Cu does, in fact, increase PCMX lethality. We evaluated the fungicidal efficacy of PCMX/Cu on Candida albicans. PCMX/Cu synergy was explored on biofilm formation, biochemistry, and morphology of C. albicans. Inhibition increased with PCMX increased concentration. The combination of Cu and PCMX disrupted fungal ultrastructure, biofilm formation, and biochemical characteristic.
1. Introduction
Chloroxylenol is more commonly known as parachlorometaxylenol (PCMX) [Citation1]. It is an antiseptic and disinfectant applied to living tissue and nonliving surfaces to kill microorganisms [Citation2]. A dramatic increase in PCMX consumption has resulted in huge quantities being dumped into sewage systems and entering aquatic ecosystems [Citation3]. Thus, strategies are being sought to reduce the lethal dose of PCMX and decrease its consumption [Citation4]. Copper (Cu) has been used as an antimicrobial agent since antiquity. From 2008–2011, the United States Environmental Protection Agency (EPA) [Citation5] registered > 300 Cu compounds as antimicrobials [Citation6]. Low concentrations of Cu are lethal to fungal cells [Citation7]. However, trace levels of Cu prevent neutrophils from killing the pathogenic yeast Candida albicans [Citation8]. C. albicans comprises part of the natural human microflora but it is also one of the most common fungal pathogens [Citation9]. It is responsible for > 90% of all serious fungal infections [Citation11]. In humans, C. albicans may infest the skin [Citation10], GI tract, mouth, and vagina [Citation11]. New antifungal agents are required to control C. albicans as it has developed resistance to numerous existing drugs [Citation12].
C. albicans can form biofilms in which densely crowded cells are embedded in a sticky matrix adhering to a surface. This trait is associated with the pathogenicity of C. albicans. Biofilm formation may be mitigated with disinfectants [Citation13,Citation14]. However, other reports have indicated that biofilm is resistant to common antifungal compounds, the host immune system, and various environmental factors [Citation15,Citation16]. We previously showed that a mixture of PCMX and Cu lowered the lethal dose of PCMX. We studied the effects of this composition on C. albicans growth, resistance, and germ tube formation [Citation4]. The objectives of the present study are to demonstrate that this mixture is fungicidal rather than fungistatic and investigate the synergy between Cu and PCMX. We also evaluated the ability of this mixture to alter biofilm formation, biochemical content, and morphological features in C. albicans.
2. Materials and methods
2.1. Materials
2.1.1. Organism
Candida albicans was obtained in slant Malt yeast extract glucose peptone agar medium (MYGP) from the Regional Center for Fungi, Alazhar University, Cairo, Egypt.
2.1.2. Media
Malt yeast extract glucose peptone medium (MYGP) was used to culture C. albicans culturing at 25 ± 1 °C. The pH was adjusted to 5.7 [Citation17].
2.1.3. Chloroxylenol (PCMX)
Chloroxylenol (PCMX) was purchased in the form of Dettol® which is 4.8% (v/v) chloroxylenol [Citation18]. The PCMX concentrations used in the C. albicans growth assay were 0, 10, 20, 30, 40, 50, 60, and 70 ppm.
2.1.4. Cu and PCMX/Cu mixture
The source of Cu was crystalline copper sulfate heptahydrate (CuSO4·5H2O, Sigma-Aldrich) [Citation19]. The Cu concentration was 0.2 mM both alone and in mixture with 20 ppm PCMX. These reagents were applied in the C. albicans biofilm formation, TEM, and biochemical content assays. These treatments were compared with additive-free control C. albicans cultures.
2.2. Methods
2.2.1. Growth measurements
C. albicans were incubated in MYGP broth at 25°C for 3 d. Absorbance of the culture was measured by spectrophotometer (Spectro 23 RS; LaboMed Labware Co. Inc., Jiangsu, China) at A550nm to compare the effects of the PCMX, Cu, and PCMX/Cu treatments.
2.2.2. Determination of the fungicidal effect of the PCMX/Cu mixture on C. albicans
C. albicans was inoculated on MYGP broth amended with 50 ppm PCMX and 0.2 mM Cu and incubated at 25°C for 3 d. Absorbance was measured at A550nm. A 100-µL aliquot of this culture were used as an inoculum for additive-free MYGP to validate the fungicidal effect of the PCMX-Cu mixture [Citation20].
2.2.3. Determination of biofilm formation
To establish C. albicans pathogenicity, the ability of the cultures to form biofilm was assessed. Ten-mL tubes each containing 5 mL MYGP broth were inoculated with C. albicans sampled from 24-h culture plates. The inoculated tubes were incubated for 24 h at 37°C, emptied, rinsed with phosphate-buffered saline (PBS; pH 7.2), and oven dried at 60°C for 2 h. The tubes were then stained with 0.1% w/v crystal violet (Sigma-Aldrich) and excess stain was washed off with milli-Q water. Two mL of 70% (v/v) aqueous ethanol (70%) was used to dissolve the stained biofilm on the wall and bottom of the tube. Colour intensity was measured spectrophotometer at A565nm to determine the amount of biofilm formed by C. albicans [Citation21].
2.2.4. Biochemical analyses
2.2.4.1. Determination of total protein
C. albicans were cultured in MYGP media, media with 20 ppm PCMX, with 0.2 mM Cu, or with PCMX / Cu mixture. Total protein was extracted as previously described [Citation22] and measured by spectrophotometry at A750nm according to a previously described method [Citation23].
2.2.4.2. Determination of total lipids
Total lipids were extracted from C. albicans cultures according to a previously described procedure [Citation24] and determined by the phosphovanillin method [Citation24]. Phosphovanillin reagent (2.5 mL) was added to the lipid extracts and a blank (0.5 mL H2SO4) and absorbances were measured at A520nm. Cholesterol was used as a reference standard. Data were expressed in mg g−1 dry C. albicans.
2.2.4.3. Determination of total carbohydrate
Total soluble carbohydrates were determined by the anthrone technique as previously described [Citation25]. The clear supernatant was diluted with a known volume of distilled water. Then a 3-mL aliquot was transferred to a clean test tube to which 6 mL freshly prepared anthrone reagent (2 g L−1 in 95% (v/v) sulfuric acid) was added. The tubes were heated in a boiling water bath for 3 min and left to cool. The colour was measured at A620nm against a blank consisting of distilled water and the reagents. Sucrose was used as a reference standard. Data were expressed in mg g−1 dry C. albicans.
2.2.4.4. Determination of citric, oxalic, and glyconic acids
Control and treated C. albicans cultures in MYGP broth were incubated for 3 d at 25 ± 1°C. Mycelia were harvested, washed, and oven dried as previously described [Citation26]. They were sonicated in milli-Q water and centrifuged at 2800 g. The supernatants of triplicate samples were analysed by HPLC to determine the citric, oxalic, and glyconic acid levels. The HPLC system was an Agilent 1100 fitted with a Model G1311A quaternary pump and a Model G1314A UV/VIS detector set to A254nm. A Hypersil Elite C18, 5-µm, 250 mm × 4.6 mm column was used for organic acid separation. The mobile phase was 0.2 M H3PO4:methanol (90:10) and applied isocratically at a flow rate of 0.8 mL min−1. Data were integrated and calculated in ChemStation and expressed in mg mL−1 [Citation27].
2.3. Transmission electron microscopy (TEM) study
All MYGP-based C. albicans cultures were examined as previously described [Citation28]. under a JEOL JSM 3500 transmission electron microscope (TEM; JEOL Ltd., Tokyo, Japan) at the Electron Microscope Unit of the Faculty of Science, Alexandria University, Alexandria, Egypt [Citation29].
3. Results & discussion
Here, we attempted to demonstrate that 50 ppm PCMX amended with 0.2 mM Cu is fungicidal rather than fungistatic. Our intentions were to formulate a new fungicide that could help avoid over application of commercial PCMX (Dettol®) and to establish whether there is a synergy of efficacy between Cu and PCMX. We subjected C. albicans to nonlethal doses of this new mixture to assess its impact on fungal biofilm formation, biochemical content, and morphological features. We choose these three markers as they are indications of vitality of C. albicans cells after the applications of the different treatments.
3.1. Percent inhibition of C. albicans by PCMX, Cu, and the mixture
The percent inhibition levels of C. albicans by PCMX, Cu, and PCMX + Cu were determined by comparing growth under these treatments with that of the control. Figure shows the percent inhibition of C. albicans in response to various concentrations of PCMX (0–70 ppm), 0.2 mM Cu, and PCMX + Cu. The percent inhibition of C. albicans increased with PCMX concentration. C. albicans inhibition increased from 3.9–100% by increasing the PCMX concentration from 10–70 ppm. An inverse relation between PCMX concentration and fungal growth was reported for Mucor [Citation30]. Here, we obtained total C. albicans inhibition at 70 ppm PCMX and at 50 ppm PCMX + 0.2 mM Cu. In contrast, 0.2 mM Cu alone inhibited C. albicans by only 16.9% inhibition.
Figure 1. Percent inhibition of C. albicans in response to various concentrations of PCMX and mixtures of different concentrations of PCMX + 0.2 mM Cu. Dark blue solid line: % inhibition of C. albicans in response to 0.2 mM Cu alone as reference. (N = 3).
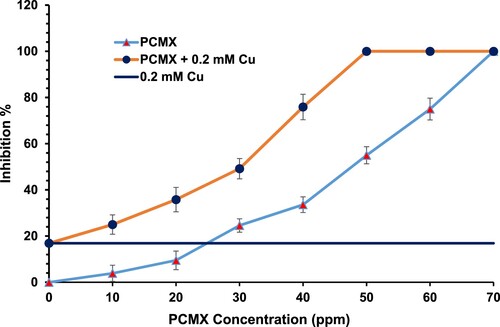
The toxicity due to Cu ions that enhance the PCMX toxicity may due to the mode of action of Cu as they promote free radical generation via the Fenton reaction, which causes lipid and protein peroxidation [Citation31]. The addition of 0.2 mM Cu to 50 ppm PCMX raised the percent inhibition of C. albicans from 55% to 100%. The foregoing data indicate that the addition of Cu to the PCMX has a synergistic effect on the percent inhibition of C. albicans. The efficacy of the PCMX-Cu mixture was higher than the sum of those for the separate PCMX and 0.2 mM Cu. Similar results were reported in our previous work [Citation4]. However, in the present study, we obtained relatively lower percent inhibitions of C. albicans at 50 and 60 ppm PCMX and observed total inhibition only at 70 ppm PCMX or 50 ppm PCMX + 0.2 mM Cu. Moreover, here we recorded only 16.9% inhibition of C. albicans with standalone Cu as opposed to 43.2% in a previous study [Citation4]. One possible explanation for this discrepancy is the Cu source: as it is Cu sulfate in the present study rather than copper chloride in the previous study. Total inhibition of C. albicans was achieved with 70 ppm PCMX or 50 ppm PCMX plus 0.2 mM Cu. These PCMX concentrations are markedly lower than those used for commercial PCMX (Dettol®). A common practice is to apply ∼2,000 ppm commercial PCMX [Citation18]. The label recommends 10 mL Dettol® in 250 mL water (=2,000 ppm PCMX). Total inhibition of C. albicans was achieved in 180 s with 5,000 ppm PCMX and in 60–90 s with 50,000 ppm PCMX [Citation32]. Other reports indicated that 5,000 ppm totally inhibited C. albicans after 20 min [Citation33]. The MLC (minimal lethal concentration) of PCMZ against Staphylococcus aureus was reported to be 1,250 ppm [Citation34]. However, the present study showed that only 70 ppm PCMX or 50 ppm PCMX + 0.2 mM Cu sufficed for the total inhibition of C. albicans.
3.2. PCMX/Cu mixture fungicidal efficacy against C. albicans
Spore forming microorganisms can be difficult to control as disinfectants may be sporostatic but not necessarily sporicidal [Citation35]. It was reported that phenolics are strongly microbicidal and destroy vegetative bacteria, fungi, and most viruses except hepatitis B. However, they are not reliably sporicidal [Citation36]. Therefore, the proposed PCMX-Cu mixture and lower concentrations of previously known disinfectants should be evaluated for their sporicidal efficacy. To investigate the fungicidal effect of PCMX-Cu, three-day C. albicans grown in MYGP broth with 50 ppm PCMX and 0.2 mM Cu were re-inoculated into additive-free MYGP broth and monitored by spectrophotometry at A550nm for 2 wks. No growth was detected in these subcultures. Thus, the PCMX-Cu mixture had fungicidal efficacy. We wanted to establish whether PCMX, Cu, and PCMX + Cu alter the biochemical responses and morphological features of C. albicans at very low PCMX concentrations. To that end, we used 20 ppm PCMX, 0.2 mM Cu and 20 ppm PCMX + 0.2 mM Cu to obtain viable C. albicans for these experiments.
3.3. Determination of biofilm formation by C. albicans after treatment with PCMX, Cu, and PCMX + Cu
The ability of C. albicans to form biofilm is an indicator of its pathogenicity. In the present study, we explore the fungicidal efficacy of the proposed new disinfectant by investigating the ability of C. albicans to form biofilm in the presence of only 20 ppm PCMX, 0.2 mM Cu, or a mixture of these compounds. The lowest reduction in biofilm formation relative to that for the control was found with the Cu only followed by the PCMX only (Table ). The lowest biofilm formation (i.e. the highest reduction) relative to that for the control was obtained with the PCMX/Cu mixture (Table ). In an earlier study, chlorhexidine inactivated C. albicans biofilm formation. Thus, it was efficacious as a disinfectant in this case [Citation13]. In another previous study, Dettol® reduce C. albicans biofilm on the surfaces of acrylic resin samples after 8 h immersion. By the end of the experiment, the PCMX had totally eliminated all C. albicans biofilm [Citation14]. In contrast, another report indicated that C. albicans biofilms were inherently resistant to most known antifungal drugs. Consequently, C. albicans infections are particularly difficult to combat [Citation15]. Moreover, resistance of C. albicans biofilms to classic antifungal drugs is multifactorial and mechanistically complex. There are no drugs available for the treatment of biofilm-based microbial infections. Another study reported that C. albicans biofilms are formed via hyphal-specific adhesions and may be able to resist the therapeutic action of certain drugs [Citation15]. The aforementioned discrepancies may be explained by the fact that different disinfectants were used in each of these experiments.
Table 1. Changes in C. albicans biofilm concentration after 24 h in response to 20 pmm PCMX, 0.2 mM Cu, and PCMX / Cu.
3.4. Changes in total protein, lipid, and carbohydrate in response to PCMX, Cu, and PCMX / Cu
PCMX, Cu, and PCMX / Cu all suppressed the biochemical functions and activity of C. albicans. PCMX + Cu caused the maximum reductions in total protein, lipid, and carbohydrate (98.8%, 48.4%, and 59.6% respectively) followed by standalone PCMX at 20 ppm. The lowest % reductions in these parameters were obtained with standalone 0.2 mM Cu (Table ). Thus, all three treatments inhibited the physiological capacity of C. albicans to produce the components vital for its survival and growth. The new mixture (PCMX + Cu) was the most potent and toxic to C. albicans. PCMX coagulates cellular proteins and nucleic acids which arrests metabolic functions and leads to rapid cell death [Citation37]. The modes of action of phenols vary with application concentration. At high doses, they are cellular poisons. They rapidly disrupt cell walls and membranes and precipitate proteins. They damage cell walls by altering the selective permeability of the cytoplasmic membrane and causing the leakage of vital intracellular substances. At lower concentrations, they inactivate critical enzyme systems [Citation38]. The Cu used in the present study may have oxidized the cellular proteins of C. albicans [Citation39]. Cu compounds precipitate proteins and coagulate the cytoplasm. Cu reacts with the sulfhydryl groups of amino acids and other compounds [Citation40]. Cu may have also lowered the total lipid content in C. albicans by peroxidizing its membrane lipids and disrupting its plasma membranes [Citation41]. PCMX / Cu caused the maximum decrease in total carbohydrate content in C. albicans (59.6%). PCMX alone reduced total C. albicans carbohydrate by 35%. Standalone Cu lowered total C. albicans carbohydrate by only 12.52% (Table ). In contrast to our findings concerning the effects of PCMX and Cu on C. albicans metabolite biosynthesis, another study indicated that fungi produce numerous extracellular proteins, organic acids, and other substances enabling them to adapt to severe environmental constraints and conditions [Citation42].
Table 2. Alterations of total protein, lipid, and carbohydrate (mg g−1 dry mass) in C. albicans in response to 20 ppm PCMX, 0.2 mM Cu, and PCMX / Cu.
3.5. Alteration of certain C. albicans organic acids by PCMX, Cu, and PCMX / Cu
PCMX inhibited the production of citric, oxalic, and glyconic acids by C. albicans. In contrast, Cu alone actually enhanced the production of citric and glyconic acids (Table ). Cu increased citric and glyconic acid production by 11.5% and 100-fold, respectively, and decreased oxalic acid by 41% relative to the control. A mixture of 20 ppm PCMX and 0.2 mM Cu reduced citric acid by 100% (Table ). A previous study reported that adsorption of Cu oxychloride onto fungal surfaces induced the excretion of acidic complexing agents which promote fungicide solubilization and tolerance [Citation42]. In the present study, PCMX + Cu totally inhibited organic acid production in C. albicans. Addition of Cu to PCMX reduced the production of oxalic and other acids. These metabolites confer toxic metal tolerance in fungi. An earlier report stated that the fungi may be able to endure environments with high concentrations of toxic metals by combining and neutralizing the contaminants with oxalic acid to form metal oxalates [Citation43].
Table 3. Changes in mg mL−1 concentrations of citric, oxalic, and glyconic acids in C. albicans in response to 20 ppm PCMX, 0.2 mM Cu, and PCMX / Cu.
3.6. Effects of PCMX, Cu, and PCMX / Cu on C. albicans morphology
The absolute thickness of the C. albicans cell membrane showed a maximum increase in response to the PCMX + Cu treatment. It was 3.6-fold thicker than that of the control. In contrast, standalone Cu increased the absolute C. albicans membrane thickness by 2.2-fold relative to the control (Table and Figure ). Standalone PCMX treatment had no effect or influence on the absolute C. albicans membrane thickness. However, it did increase the relative C. albicans membrane thickness by 2.2-fold compared with the control. The standalone Cu and PCMX / Cu treatments increased the relative C. albicans membrane thickness by 3.3-fold and 4.8-fold, respectively, relative to the control. The comparative changes in the absolute and relative C. albicans membrane thicknesses confirmed the synergy of efficacy between Cu and PCMX. The present study demonstrated that the synergy between Cu and PCMX accounted for the maximum increase in C. albicans cell membrane thickness. Transmission electron microscopy revealed that the increase in C. albicans membrane thickness observed in response to the treatments may have been the result of Cu binding to the cell membrane. Candida utilis grown in Cu-containing media presented with dense Cu-containing particles, lipid globules, vacuoles, and cell membrane thickening [Citation44]. Moreover, another mechanism by which fungi tolerate heavy metals it that they may be able to bind them in their cell membranes [Citation45]. The chemical composition of the cell membranes of Cunninghamella blakesleena were altered after it was grown in media containing Cu or cobalt [Citation46].
Figure 2. Micrographs of ultrathin sections of C. albicans in (A) absence of Cu or PCMX; (B) presence of 0.2 mM Cu; (C) presence of 20 ppm PCMX; and (D) presence of 0.2 mM Cu + 20 ppm PCMX. The micrograph illustrate some of the important cell structure e.g. cell wall (CW), cell membrane (CM), and vacuoles (V).
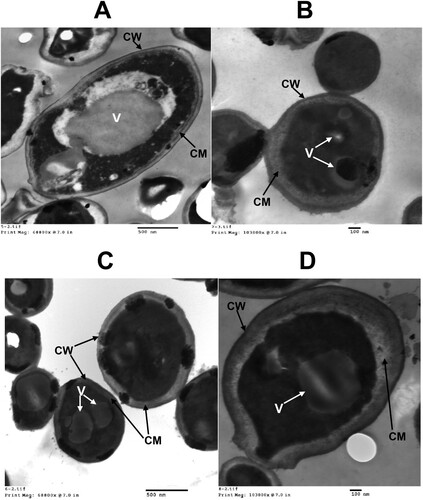
Table 4. C. albicans Plasma membrane (nm), cell diameter (nm), and relative mebrane thickness (%) after treatment with 20 ppm PCMX, 0.2 mM Cu, and PCMX / Cu as seen under transmission electron microscope (TEM).
4. Conclusions
The % inhibition of C. albicans increased with PCMX concentration and the addition of 0.2 mM Cu to the PCMX. Maximum inhibition (100%) was observed for the 70 ppm PCMX and 50 ppm PCMX +0.2 mM Cu treatments. There was a synergy of efficacy between PCMX and Cu. Addition of 0.2 mM Cu sulfate to PCMX reduced the lethal dose of PCMX against C. albicans to 50 ppm. Here, we demonstrated that 50 ppm PCMX + 0.2 mM Cu is fungicidal and not merely fungistatic. C. albicans vitality was diminished after treatment with only 20 ppm PCMX + 0.2 mM Cu. The combination of Cu and PCMX disrupted fungal ultrastructure and the production of biofilm, proteins, lipids, carbohydrates, and organic acids.
Acknowledgements
This work was funded by the Deanship of Scientific Research (DSR) of the University of Jeddah, Jeddah, Saudi Arabia (Grant No. UJ-31-18-DR). The authors also thank DSR for their technical and financial support.
Disclosure statement
No potential conflict of interest was reported by the author(s).
Additional information
Funding
References
- Chan TY, Critchley JA. Is chloroxylenol nephrotoxic like phenol? A study of patients with Dettol poisoning. Vet Hum Toxicol. 1994;36(3):250–251.
- WHO, World Health Organization. WHO model formulary 2008, Stuart MC, Kouimtzi M, Hill SR, editors. 2009. https://apps.who.int/iris/handle/10665/44053
- Basha KM, Rajendran A, Thangavelu V. Recent advances in the Biodegradation of Phenol. A review. Asian J Exp Biol Sci. 2010;1:219–234.
- Gharieb MM, Elhefnawy MA, Ibrahim A, et al. Copper enhancement of Dettol Lethality to Candida albicans. J Pure Appl Microbiol. 2015;9:521–528.
- Environmental Protection Agency (EPA). The toxic chemical release inventory. (United States); 2015.
- Ladomersky E, Petris MJ. Copper tolerance and virulence in bacteria. Metallomics. 2015;7(6):957–964. doi: 10.1039/C4MT00327F
- Morgan JJ, Stumm W. Chemical processes in the environment, relevance and chemical speciation. In: Merian E, editor. Metal and their compounds in the environment-occurrence analysis, and biological relevance. Weinheim: VCH; 1991. p. 67–103.
- Li C, Li Y, Ding C. The role of copper homeostasis at the host-pathogen axis: from bacteria to fungi. Int J Mol Sci 2019;20(1):175–189. doi: 10.3390/ijms20010175
- Seladi-Schulman J. About Candida albicans: Natural yeast and problematic infections, Medical News Today. 2018. Availablefrom: https://www.medicalnewstoday.com/articles/322722.php.
- Kühbacher A, Burger-Kentischer A, Rupp S. Interaction of Candida Species with the skin. Microorganisms. 2017;5(2):32–43. doi: 10.3390/microorganisms5020032
- Kauffman CA. Fungal infections. Proc Am Thorac Soc. 2006;3:35–40. doi: 10.1513/pats.200510-110JH
- Zida A, Bamba S, Yacouba A, et al. Anti- Candida albicans natural products, sources of new antifungal drugs: a review. J Mycol Méd. 2017;27:1–19. doi: 10.1016/j.mycmed.2016.10.002
- Mc Courtie J, Mac Farlane TW, Samaranayake LP. Effect of chlorhexidine gluconate on the adherence of Candida species to denture acrylic. J Med Microbiol. 1985;20:97–104. doi: 10.1099/00222615-20-1-97
- Zoccolotti JO, Tasso CO, Arbeláez M, et al. Properties of an acrylic resin after immersion in antiseptic soaps: Low-cost, easy-access procedure for the prevention of denture stomatitis. PloS one. 2018;13(8):1–22. e0203187. doi: 10.1371/journal.pone.0203187
- Gulati M, Nobile CJ. Candida albicans biofilms: development, regulation, and molecular mechanisms. Microbes Infect. 2016;18(5):310–321. doi: 10.1016/j.micinf.2016.01.002
- Cavalheiro M, Teixeira MC. Candida biofilms: threats, challenges, and promising strategies. Front Med. 2018;5:28–42. doi: 10.3389/fmed.2018.00028
- De Jong J, Put HMC. Biology and activities of yeasts. Soc Appl Bacteriol. 1980;9:289–292.
- Reckitt Benckiser Healthcare (UK) Limited (Licence holder of manufacturer of dettol); 2019.
- Greenwood NN, Earnshaw A. Chemistry of the elements. 2nd ed. Oxford: Butterworth-Heinemann; 1997; ISBN, 0-7506-3365-4.
- CLSI document M07-A9. Methods for dilution antimicrobial susceptibility tests for bacteria that grow aerobically. Approved Standard—Ninth Edition. Wayne (PA): Clinical and Laboratory Standards Institute; 2012.
- Janakiram B, Myneni RB, Ashok Kumar K, et al. Methods of determination of biofilm formation by Candida albicans. Res J Microbiol. 2017;12:90–96.
- Zaidi KU, Mani A, Thawani V, et al. Total protein profile and drug resistance in Candida albicans isolated from clinical samples. Mol Biol Int. 2016;2016:1–6. doi: 10.1155/2016/4982131
- Lowry OH, Rosebrough NJ, Farr AL, et al. Protein measurement with the Folin phenol reagent. J Biol Chem. 1951;193(1):265–275.
- Barnes H, Blackstock J. Estimation of lipids in marine animals and tissues: detailed investigation of the sulphophosphovanilun method for ‘total’ lipids. J Exp Mar Biol Ecol. 1973;12:103–118. doi: 10.1016/0022-0981(73)90040-3
- Umbriet WW, Burris RH, Stauffer JF, et al. Manometric techniques, a manual describing methods applicable to the study of tissue metabolism. Minneapolis: Burgess Publishing Company; 1959. p. 239.
- Gadd GM, Griffiths AJ. Influence of pH on toxicity and uptake of copper in Aureobasidium pullulans. Trans Br Mycol Soc. 1980;75:91–96. doi: 10.1016/S0007-1536(80)80198-7
- Cottier F, Tan AS, Xu X, et al. MIG1 regulates resistance of Candida albicans against the Fungistatic effect of weak organic acids. Eukaryotic Cell. 2015;14(10):1054–1061. doi: 10.1128/EC.00129-15
- Kierans M, Staines AM, Bennett H, et al. Silver tolerance and accumulation in yeasts. Biol Met. 1991;4:100–106. doi: 10.1007/BF01135386
- Gharieb MM, Gadd GM. Influence of nitrogen source on the solubilization of natural gypsum (CaSO4.2H2O) and the formation of calcium oxalate by different oxalic and citric acid producing fungal strains. Mycol Res. 1999;103:473–481. doi: 10.1017/S0953756298007382
- Okore CC, Mbanefo ON, Onyekwere BC, et al. Antimicrobial efficacy of selected disinfectants. Am J Biol Life Sci. 2014;2(2):53–57.
- Ehrensberger KM, Bird AJ. Hammering out details: regulating metal levels in eukaryotes. Trends Biochem Sci. 2011;36:524–531. doi: 10.1016/j.tibs.2011.07.002
- Atayese AO, Effedua HI, Oritogun KS, et al. Comparative study of the antimicrobial activity of chlorinated and non-chlorinated antiseptics against C. albicans. Academia Arena. 2010;2(9):35–40.
- Olorode OA, Okpokwasli GC. The efficacy of disinfectants on abattoirs’ Candida albicans isolates in Niger Delta region. F1000 Res. 2012;1:20–28. doi: 10.12688/f1000research.1-20.v1
- Azeez A, Utsalo JS, Epoke JJ. Epidemiological profile of Methicillin-resistant Staphylococcus aureus in a poor-resourced area of Sub-Saharan Africa. African J Clin Exp Microbiol. 2011;11(3):150–158.
- Johnston MD, Lambert RJW, Hanlon GW, et al. A rapid method for assessing the suitability of quenching agents for individual biocides as well as combinations. J Appl Microbiol. 2002;92:784–789. doi: 10.1046/j.1365-2672.2002.01584.x
- Talaro K, Talaro A. Foundation in microbiology. 2nd ed. New York: Wm. C. Brown Publishers, Mc Graw Hill; 1996. p. 30–77.
- Cordeiro Y, Macedo B, Silva JL, et al. Pathological implications of nucleic acid interactions with proteins associated with neurodegenerative diseases. Biophys Rev. 2014;6(1):97–110. doi: 10.1007/s12551-013-0132-0
- Pelczar MJ, Chan ECS, Kleig NR. Microbiology concepts and applications. 1st ed. New York: McGraw Hill Inc; 1993. p. 221–237.
- Yoshida Y, Furuta S, Niki E. Effects of metal chelating agents on the oxidation of lipids induced by copper and iron. Biochim Biophys Acta. 1993;1210(1):81–88. doi: 10.1016/0005-2760(93)90052-B
- Hugges MN, Poole RK. Metals, microorganisms and biotechnology. Metals and microorganisms. Heidelberg, New York: Springer; 1989. p. 303–358.
- Avery SV, Howlett NG, Radice S. Copper toxicity towards Saccharomyces cerevisiae: dependence on plasma membrane fatty acid composition. Appl Environ Microbiol. 1996;62:3960–3966. doi: 10.1128/AEM.62.11.3960-3966.1996
- Coulibaly L, Gourene G, Agathos NS. Utilization of fungi for biotreatment of raw wastewaters. Afr J Biotechnol. 2003;2:620–630. doi: 10.5897/AJB2003.000-1116
- Gharieb MM, Ali M, El-shoura AA. Transformation of copper oxychloride fungicide into copper oxalate by tolerant fungi and the effect of nitrogen source on tolerance. Biodegradation. 2004;15(1):49–57. doi: 10.1023/B:BIOD.0000009962.48723.df
- Khovrytchev MP, Strunk C, Schuhmann E, et al. Effect of Cu+2 ions on the morphological, cytological, and physiological state of Candida utilis cells in continuous culture. Z. Allg Mikrobiol. 1977;17(1):29–45.
- Garcia-Taledo A, Babich H, Stotzky G. Training of Rhizopus stolonifer and Cumninghamella blakesleeana to cadmium, cobalt, nickel and lead. Can J Microbiol. 1985;31:485–492. doi: 10.1139/m85-090
- Venkateswerlu G, Stotzky G. Binding of metals by cell walls of Cumninghamella blakesleeana grown in the presence of copper or cobalt. Appl Microbiol Biotechnol. 1989;31:619–625. doi: 10.1007/BF00270806