Abstract
This study presents microscopic results of 50 samples and 5 in situ magnetic susceptibility profiles, field investigations and bedrock characteristics of northern Harrat Rahat Quaternary volcanic rocks, located in the southern part of Al-Madinah city. The petrographic data of volcanic rocks show mainly aphanitic (fine-grained) holocrystalline basaltic units, rich in olivine depicting porphyritic, glomeroporphyritic, ophitic, subophitic, dictytaxitic, seriatic, interstitial, and trachytic textures. Field observations and petrographic studies reveal 9 rock units, from oldest to youngest: olivine basalt, hawaiite, vesicular olivine basalt, alkali olivine basalt, mugearite, benmoreite, scoriaceous basalt, trachytoid basalt and pyroclastic rocks. The magnetic susceptibility data vary from one rock to another based on texture, alteration, grain size, opaque minerals content, and weathering. The Fe-rich and massive basaltic rocks generally reveal higher susceptibility, while basaltic scoria and vesicular, fractured and weathered basaltic rocks generally reveal lower susceptibility. Occurrence of highly mature opaque minerals might suggest an associated sulphide mineralization.
1. Introduction
The basaltic lava flow eruptions, exposed in the southern parts of Al-Madinah, have some fresh exposures of extrusive igneous rocks, which have been recently excavated for roads and building constructions. These basaltic exposures contain one of the most recent exposed volcanic rocks (1.7 m.y. to 1256 A.D., [Citation1]), and therefore, are considered as one of the best examples of the monogenic intra-continental volcanic fields that worth preserving as a geo-park [Citation2]. Also, it has a good potential for geothermal energy [Citation3]. Petrography is essential in studying these volcanic rocks to compare mineralogical and petrological changes between different basaltic flows. Theoretically, the neo-volcanic rocks are strongly magnetic and a variation in their susceptibility can be an important geological indicator [Citation4]; therefore, both petrology and magnetic susceptibility are combined in this study.
Magnetic susceptibility (MS) is one of the most easily measurable petrophysical parameters that reflect the magnetic response of a material to an external magnetic field. Thus, the magnetic susceptibility is controlled by the type and amount of magnetic minerals contained in the rock [Citation4]. Moreover, it is dominantly controlled by paramagnetic minerals (i.e. mafic silicates such as olivine, pyroxenes, amphiboles, micas, tourmaline, garnets), often by ferromagnetic minerals (e.g. iron oxides and sulphides, represented, for instance, by magnetite and/or pyrrhotite, respectively) and much less frequently by diamagnetic minerals (e.g. calcite and quartz, [Citation4]). In addition, the accessory mineral components are sensitive indicators of geological processes as well. Magnetic susceptibility is a measurement of how magnetizable the material is. Rocks with relatively high concentrations of magnetite, like basalt, may have much higher magnetic susceptibility values than the other fresh barren igneous rocks. Geoscientists in different countries have successfully used magnetic susceptibility techniques to map igneous rocks [Citation5–7].
The main aim of this study is to differentiate between different volcanic rocks located in the southern part of Al-Madinah city using in situ magnetic susceptibility, field characterizations, and detailed petrographic observations (Figure ). To achieve this goal, 5 profiles of basaltic rocks in the southern part of Al-Madinah city have been chosen for investigation. Profile 1 and profile 2 are located west of Ayr Mountain on eastern and western sides of Prince Sultan bin Abdul Aziz road, respectively, where the roads run near the contact between the massive basaltic units and Ayr Mountain, while Profile 3 is conducted on a scoria cone, south Ayr Mountain, along the third ring road (Figure ). Profile 4 is conducted on another younger scoria cone to the southeast of Jabal Ayr Mountain, and finally Profile 5 is chosen for its location near the historical flow and volcano (1256 A.D.). Figure shows locations of the five profiles on the geological map Al-Madinah Al-Munawwarah 1:250,000 quadrangle [Citation8].
Figure 2. Geological map of the northern part of Harrat Rahat showing the five basaltic units, and the location of the studied five profiles in Madinah area; Modified after [Citation8]; Rocks age after [Citation1,Citation9,Citation12,Citation17].
![Figure 2. Geological map of the northern part of Harrat Rahat showing the five basaltic units, and the location of the studied five profiles in Madinah area; Modified after [Citation8]; Rocks age after [Citation1,Citation9,Citation12,Citation17].](/cms/asset/1138867e-39b0-4cdf-8ee8-6363414a9b6b/tusc_a_1796477_f0002_oc.jpg)
2. Geological setting
The volcanism in western Arabian Shield is represented by sub-alkali to alkali basaltic fields, in which these intra-continental fields are mainly mafic in compositions, but they also include variants with intermediate composition such as mugearites, benmoreites, trachytes, and phonolites [Citation1,Citation9,Citation10]. [Citation1] stated that the volcanic activities are associated with the evolution of the Red Sea, occurred during Oligocene and continued to the historic times (till 1256 A.D.). The intra-continental volcanism produces different basaltic outcrops including lava flows and associated pyroclastic rocks, scoria cone, tuffs, lava tubes as well as shield cones from north to south of the Arabian Shield along the Red Sea. These flood basaltic rocks have several igneous rocks-related primary structures, such as vesicular and amygdaloidal columnar joints basaltic flow overlying loosely consolidated tephra beds (Figure ). The Harrat Al-Madinah represents the northern Harrat Rahat (Figure ) that is mainly formed by monogenic basaltic rock bodies and flows [Citation11]. The volcanic activity of Harrat Rahat was considered to be related to a younger (10 Ma) N-S trending crustal rift-system termed Makkah–Madinah–Nafud (MMN) volcanic line, almost parallel to the Red Sea structure [Citation12]. Moreover, [Citation13] discovered that the presence of right-stepping en-echelon open fractures indicates that the region is affected by a shear component of left lateral sense of movement. Meanwhile, the NNW to NW direction of the en-echelon fractures indicates an almost N–S trend of the main shear component due to an ENE to NE extension [Citation14].
The northern Harrat Rahat comprises 501 volcanic cinder cones in N–S, NNW–SSE, and NW–SE alignments with a spatial density up to 17 cones/ 10 km [Citation10]. These rocks are alkali to sub-alkali in nature and have Oceanic Island Basalt (OIB) affinity, which erupted away from tectonic boundaries. Different authors [Citation9,Citation10,Citation11,Citation13,Citation14] have reported pyroclastic cones and associated lave flows (Figure ), rare lava domes, and phreatomagmatic structures (including tuff cones, tuff rings, and maar explosion craters). This volcanic chain of scoria cones (from 30 to 200 m height) is surrounded by fields of coalescing low viscosity basaltic lava flows [Citation1]. The lavas are black to dark brown in colour exhibiting well-preserved primary structures such as lava tubes and ropy lava flow [Citation14, Figure ]. The scoria cones and lava flows (Figure ) are typically the most common types of effusive (steadily lava flows) eruptions in the Arabia Shield [Citation15]. Intermediate and silicic activities mainly produce domes and pyroclastic deposits [Citation16–18]. The lava flow followed stream channels between older flows toward Al-Madinah. The flow units are thinnest near the scoria cones, where flow fronts are 2–3 m high. However, they systematically thicken away from the cones; at 10 km, the flow fronts are 4–8 m height, and at the terminus, they are 7–8 m high [Citation17]. [Citation8] divided the rocks of northern Harrat Rahat into 5 major basaltic units (B1, B2, B3, B4, and B5) as shown in Figure . [Citation1] divided the B2–B5 units into 7 rock units, calling them as Al-Madinah Harrat, in which B2 consists of eroded olivine basalt and minor hawaiite flow; B3 consists of olivine basalt, hawaiite, and mugearite; B4 consists of olivine basalt, hawaiite, mugearite, benmoreite, and trachyte, where B5 is composed of olivine basalt and minor hawaiite.
The Neogene basalt (B1) occurs above the mountains, such as Jabal Ayr Mountain, while Quaternary basalt represents the lower units of northern Harrat Rahat (B2 and B3), and the upper northern Harrat Rahat (B4 and B5) [Citation9,Citation12,Citation17]. The lower (B2–B3) northern Harrat units were divided into three subunits: Qm1 (1.7–1.2 Ma), Qm2 (1.2–0.9 Ma), and Qm3 (0.9–0.6 Ma). The upper northern Harrat Rahat (B4–B5) is divided into four subunits: Qm4 (0.6–0.3 Ma), Qm5 (0.3 Ma–4500 BP), QM6 (4500–1500 PB), and QM7 (1500 P.B.–1256 A.D.). Therefore, Quaternary Al-Madinah basaltic rocks have been dated in a range that vary from 1.7 ma to recent [Citation1,Citation9,Citation12,Citation17]. All these eruptions are basaltic in composition and mostly produced by effusive Hawaiian style (lava flows out steadily), and Strombolian style (lava fragments) except the historical flow (1256–641 A.D. eruption) that has evidence of phreatomagmatic (interaction between magma and large amount of water) activity [Citation10,Citation19].
This study follows the geological map of [Citation8]; therefore, the 5 measured profiles that have been magnetically and petrographically studied are assigned to B2–B5 Quaternary units (Figure ). The studied basaltic rock units exhibit different volcanic landforms, reflecting different styles of activities (Figure ). Lave flows of B2 and lower B3 units are composed mainly of massive olivine basalts and hawaiite reflecting the Hawaiian style. The upper B3 and B5 Scoria cones are generally associated with vesicular olivine to alkali olivine basalt, mugearite, benmoreite, and trachyte as well as pyroclastic rocks, suggesting the Strombolian style. B4 and lower B5 have a-a, ruby structure, lava tube, and columnar joints at the upper part, suggesting phreatomagmatic activities (Figure ). Furthermore, these basaltic rocks extruded and surrounded the Pre-Cambrian rocks of Jabal Ayr and the oldest Neogene basaltic rock unit (B1) that overlies the Pre-Cambrian rocks of Jabal Ayr Mountain in the western part of the study area. In this respect, Jabal Ayr Pre-Cambrian rock units are part of the Furayh group that consists mainly of metavolcanoclastic rocks (i.e. metamorphosed sandstone, greywackes, conglomerate, and siltstone interbedded with meta-pyroclastic felsic rocks).
3. Material and methods
Polished thin sections of 50 collected volcanic samples were prepared in the laboratory for microscopic observations. The main goal was to analyse these thin sections for minerals, micro-texture, and structure by optical microscopy as critical clues to understanding the origin of the studied rocks. These results are then compared with the magnetic susceptibility results to confirm the presence of rock-forming minerals. Fresh samples were collected, transported, and preserved in safe bags to avoid any possible contaminations. The selected rock samples were then cut into chips. Figure shows slabs of different rock units with average diameters of 3 cm x 2 cm x 1 cm and their corresponding magnetic susceptibility values. The prepared slabs were then cut and polished with silicon carbide powder. An automated thin section machine, called Multi-Plate Grinder, was used for making slides up to a thickness of approximately 45 microns. Following this, heavy duty polishing table and hand polishing using silicon carbide were used to grind the slides down to 30 microns.
Figure 4. Photographs of the studied volcanic rocks with their magnetic susceptibility (MS) values measured in international system (SI). Ca = calcite and Ze = zeolite; Ca and Ze are depicted in thin sections given in Figure .
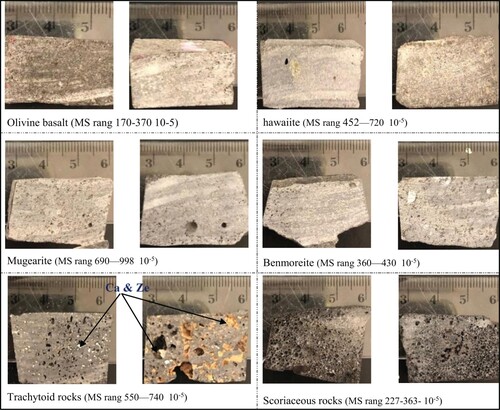
To study magnetic content of minerals, Bartington MS2 magnetic susceptibility meter was used on rock exposures in the field area. MS2 is a portable meter with an integral four-digit display. Two field sensors (MS2D and MS2F) were used in conjunction with the MS2 meter for surface measurements in the field. As described by [Citation20], these sensors are specifically designed for outdoor use. This set of instrument tolerates scratches, knocks, and normal temperature changes without showing any significant decrease in sensitivity. The mentioned sensors also tolerate immersion in water.
A measuring range of 1.0 was selected and the M/Z toggle switch centred. [Citation4] stated that, as a major advantage, field measurement of magnetic susceptibility on rock outcrops is very fast, one measurement taking a few seconds, and one can execute numerous detailed measurements in reasonable time. To do the same amount of measurements through taking samples and their subsequent measurement in laboratory would be impracticable. Field measurement enables us to detect subtle changes in magnetic mineral content within the geological body or outcrop that are not macroscopically observable. In this way, cryptic magnetic and non-magnetic layers, lenses, enclaves, and other bodies can be identified as well as gradual changes in the content of magnetic minerals.
4. Results
Results demonstrate the potential of using the magnetic susceptibility technique in discrimination between the studied basaltic rocks in combination with the petrographic studies. Careful sample selection, polished section examination, and magnetic susceptibility measurements in both geological field and laboratory cautiously have been achieved. In this respect, the obtained results would give a new insight into the subdivisions of the Harrat Al-Madinah volcanic field. Detailed magnetic susceptibility measurements were made on a variety of basaltic rocks at five profiles of the study area. A significant variation in magnetic susceptibility is observed from one rock body to another. These variations very much depend on the type of rocks, their mineralogical composition, and on alteration processes that affected the rocks after their formation. According to [Citation4], some chemical changes induced by the interplay of magmas are manifested in the susceptibility values and can be too weak that they are not simply visible in the rock composition. Detailed magnetic susceptibility results and the corresponding petrographic description of each profile are given below.
4.1. Profile 1: lower (B3) unit
Magnetic susceptibility was measured at 14 points at 4 m distance from each other. The measurements were started from the right side of the profile (north side geographically) along an eastern side of the road cut. An overall width of this profile is about 55 m and its height from the base is about 2.5 m. The points 9 and10 are located in an area where a weathered soil layer has been recognized. In general, the type of rock in this profile varies between massive and vesicular basalt with a sign of brownish colour weathering affects (Figure ). The colour of the rocks here varies from dark grey to light grey. The texture is generally brecciated with a significant decrease in pore spaces. Magnetic susceptibility readings show a significant variation from one point to another (Figure ). The highest susceptibility values at some points are most likely caused by strong iron bearing minerals, including magnetite and titanomagnetite. The effects of weathering at some points are very much indicated by low susceptibility values, which may be due to the alteration of high susceptibility magnetic minerals into low susceptibility minerals. Brownish colour of the collected sample indicates some sort of hematization, causing a decrease in magnetic susceptibility.
Figure 5. Variation of magnetic susceptibility along profile-1; vesicular olivine basalt (a and b), hawaiite (c and d), and mugearite (e and f). The microphotographs have been taken in crossed nicols. Ol = olivine, Plg. = Plagioclase, Fe-Ox = Iron oxides, Sa = Sanidine, Aug.= Augite.
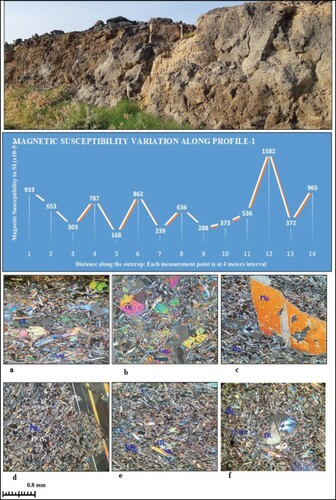
In thin section the coarse-grained vesicular olivine basalt shows a framework of plagioclase laths 1–3 mm long (Figure (a,b)) and depicts porphyritic to sub-ophitic textures. Moreover, large irregular vesicles bordered by phenocrysts to exhibit dictytaxitic texture are also observed. Angular vesicles (1–5 mm in diameters) form nearly 30% of the rock volume. These vesicles are sometimes filled with calcite and quartz as well as the opaque minerals that are lining the cavities and form rosettes of needle-like-shaped crystals. Moreover, the rock is composed essentially of glassy groundmass containing abundant olivine, clinopyroxene, and plagioclase phenocrysts with less frequent opaque minerals (magnetite and ilmenite). Skeletal coarse-grained (from 1 to 3 mm diameters), subhedral to anhedral spinal-rich macro-olivine crystals are the most obvious phenocrysts, while fresh clinopyroxine and plagioclase phenocrysts construct together a subophitic texture. Plagioclase is present either as fine-grained euhedral–subhedral prismatic to lathic crystallites or as large crystal forming phenocrysts within the vesicular glassy groundmass. The plagioclase feldspar is twinned and few of them show zoning and reaction boundary.
Hawaiite is olivine basalt with a composition between alkali basalt and mugearite. In thin section, hawaiite exhibits relatively equal sized olivine and pyroxene grains with pinkish-grey pyroxenes (Figure ). The studied hawaiite is intergranular-to-intersertal andesine (An50) basalts, which have variable petrographic characteristics. It commonly contains megacrysts and microxenoliths, whereas phenocrysts are set in an intergranular groundmass. The phenocrysts (up to 3 mm across, Figure (c,d)) are represented by olivine and plagioclase (An60). Moreover, skeletal colourless olivine microphenocrysts, granular clinopyroxene, and plagioclase are also embedded in fine-grained plagioclase (An50) and titano-augite groundmass. Meanwhile, varied amounts of apatite are also recorded as interstitial components, and occur as needles included in both plagioclase and olivine. Furthermore, equant opaque minerals and opaque patches are easily recorded and cover most of the thin section.
In thin sections, mugearites have abundant microphenocrysts of andesine plagioclase (An53–An48), skeletal brownish green fayalitic olivine and equant opaque minerals and patches (Figure (e,f)). Most samples have seriate textures with trachytic flow of the plagioclase laths. The groundmass is very fine grained, and composed essentially of oligoclase plagioclase laths (An17), olivine (< 5%), augite, pale green aegirine-augite, alkali feldspar, granular titano-magnetite reddish-brown amphibole, and apatite. Compared with the olivine basalts, phenocrysts here are generally less abundant and smaller. Calcite is also present as secondary alteration product. However, the highly evolved hawaiite-mugearite basalt is less abundant than alkali olivine basalt and it appears to have been extruded mainly in the later phase of volcanic activity [Citation14]. Most of the studied trachy-andesitic rocks are generally show trachytic to typical diktytaxitic textures. In contrast to olivine and alkali olivine basalt, they depicted lighter grey colour and tend to be more viscous and vesicular.
4.2. Profile 2: olivine basalt and hawaiite of (B2)
In general, basalts at this profile are not brecciated and are very well developed. A major natural phenomenon in this profile is the dark-grey columnar structure (Figure ). This profile contains two types of basaltic rocks. The area around point No.6 represents a weathered part with a width of 13 m. The upper part of this profile is mostly brown to light brown, indicating visible effects of weathering on the rock bodies. Horizontally, the colour of rocks is getting lighter to the left with the increase in pore spaces and mica-related minerals. Compared to profile-1, magnetic susceptibility values here are significantly lower (Figure ), indicating low concentration of ferromagnetic minerals and higher concentration of silica-dominant minerals.
Figure 6. Variation of magnetic susceptibility along profile-2. A photograph of this profile is also given on top; a and b are microphotographs in crossed nicols of the studied olivine basalt. Symbols as Figure , Ne = Nephline.
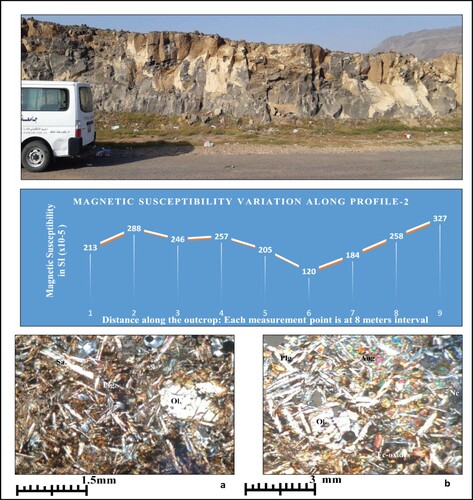
Magnetically, this phenomenon indicates the dominant presence of diamagnetic and paramagnetic minerals as a replacement to ferromagnetic minerals (magnetite and titanomagnetite). Weathering at different parts of this profile has probably caused a further decrease in magnetic susceptibility values, as evident from points 6 and 7. In thin section, the olivine basalt shows no visible difference in the main rock-forming minerals from those of profile-1. The main difference in composition is a decrease of opaque minerals in the olivine basalt. Subsequently, a decrease of iron rich minerals leads to a decrease in their magnetic susceptibility values.
4.3. Profile 3: unit (B3) scoria cone
This profile located to the south of Jabal Ayr. At this profile, magnetic susceptibility is measured at 4 points from bottom to top (at one-m distance). An overall width of this profile is about 7 m from the base. Rocks at this profile are not massive, but a mixture of loose materials including volcanic ash and weathered pebbles (unconsolidated scoria, Figure ). The colour of rocks here changes from black to brown and light. Generally, low values of magnetic susceptibility from this profile (Figure ) indicate the nature of rocks. As per our experience in the field, the unconsolidated and scoriaceous parts revealed lower values of magnetic susceptibility, indicating lower concentration of magnetite or titanomagnetite. A significantly higher value of susceptibility at point No. 4 may indicate an iron-rich inclusion of solid basaltic piece within the volcanic ash/scoria.
Figure 7. Variation of magnetic susceptibility along profile-3. A photograph of this profile is also given on top; microphotographs in crossed nicols of vesicular alkali olivine basalt are noted as c1–c4. Symbols as Figure ; Ca = Calcite, Ze = Zeolite and Ne = Nephline.
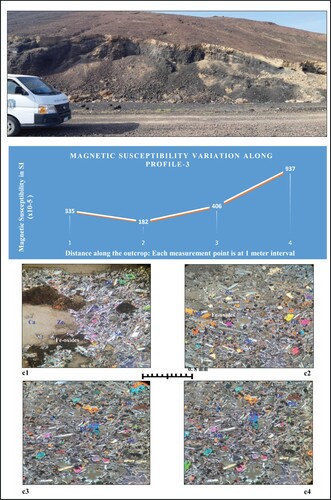
In thin section, the alkali olivine basalt shows uniform mineralogy and texture. Fine-grained textures vary from non-vesicular to vesicular, trachytic to dictytaxitic. The rocks here contain up to 10–20% olivine, 20–25% clinopyroxene, more than 50% plagioclase, and nearly 10% opaque minerals. A few alkali-rich minerals, such as k-feldspar and nepheline, can also be identified in polarized light (Figure (c1–c4)). The studied alkali olivine basalts generally contain olivine phenocrysts that are occasionally altered. The more fayalitic olivine rims are altered to iddingsite, particularly in the flows that are more vesicular and have trachytic texture. In addition, zoned clinopyroxene phenocrysts are also recorded in less amount than olivine. The phenocrysts of clinopyroxene are commonly ragged, probably due to their reaction with the melt. These phenocrysts and the groundmass crystals are titanium-rich diopsides. Microphenocrysts of labradorite and skeletal olivine are found in most samples. Fine-grained groundmass depicts intergranular-to-intersertal texture, and composed essentially of subhedral to anhedral brown titan-augite, olivine, Fe-oxides, and plagioclase laths. The groundmass consists mainly of plagioclase laths, ophitic to inter-granular clinopyroxene, and hornblende with disseminated iron oxide minerals. The groundmass plagioclase laths are labradorite (An72-An50) and the nepheline mineral grains filled the interstitial spaces between these plagioclase laths. Most of the amygdales are filled with carbonate minerals. Very rare glasses are also noticed in the groundmass. It is important to note that the opaque minerals depict erratic distribution and occur usually as patches and sometimes as filling materials in the voids. This erratic distribution gave rise to the magnetic susceptibility to increase reaching 937 at point 4, whereas the highest content of the iron bearing minerals was recorded in this sample (Figure (c1–c4)).
4.4. Profile 4: unit (B4/B5) scoria cone
This west–east directed profile is located in an area not far from profile 3. Here, six magnetic susceptibility measurements were made at 4 m interval. An overall width of this profile is 25 m and its height from the base is about 20 m. This profile clearly manifests two types of rocks; one is dominated by scoria deposits, and the other is occupied by solid basaltic rocks. The rocks here have dark colour scoria with increasing vesicular texture (rock being pitted with many cavities at its surface and inside). Some of the basaltic rocks have amygdaloidal texture as well, which have cavities or vesicles filled with secondary minerals, such as zeolites, calcite, quartz, or chalcedony.
As evident from the magnetic susceptibility graph (Figure ), the first two points have lower susceptibility values, which match very well with low magnetic scoria. The next three points (3–5) indicate a significant increase in susceptibility, which may be due to an increased concentration of ferromagnetic minerals and iron oxide patches (magnetite etc. Figure ). Low susceptibility value at point 6 is probably due to the effects of diamagnetic minerals in the vesicle of rocks. Under the microscope, both vesicular basaltic (B5 of [Citation8]) and mugearite rock units collected along this profile depict mineral contents and characteristics similar to that given in profile-3 and profile-1, respectively.
Figure 8. Variation of magnetic susceptibility along profile-4. A photograph of this profile is also given on top; Thin section microphotographs of benmoreite (b4 of [Citation8]) in crossed nicols are given at bottom as c1 and c2. Symbols as Figure .
![Figure 8. Variation of magnetic susceptibility along profile-4. A photograph of this profile is also given on top; Thin section microphotographs of benmoreite (b4 of [Citation8]) in crossed nicols are given at bottom as c1 and c2. Symbols as Figure 5.](/cms/asset/19da08f4-0577-4b4c-af62-ced8d6334aad/tusc_a_1796477_f0008_oc.jpg)
The sodium-rich trachy-andesite (B4 of [Citation8]), benmoreite exhibit porphyritic to glomeroporphyritic, and trachytic textures in the studied thin sections. Moreover, thin section observations indicate abundant phenocrysts of anorthoclase, fayalitic olivine, sanidine, and aegirine-augite (Figure ). The groundmass is fine-grained (0.1–0.4 mm), containing sanidine, fayalitic olivine, sodic plagioclase, aegirine–augite, and ilmenite with occasional interstitial calcite. A megacryst cluster of sodic plagioclase with a broad outer rim of anorthoclase is noticed. Some samples are aphyric and composed of variable amounts of olivine with apatite needles, sodic plagioclase, and equant opaque oxides. Similar to mugearites, the benmoreite groundmass commonly shows a trachytic flow texture and contains variable amounts of plagioclase laths (oligoclase), aegirine-augite, equant opaque oxide, fayalitic olivine, alkali feldspar, reddish-brown amphibole, and secondary calcite. It is important to note that the increase in magnetic susceptibility value (up to 1134) in this profile is related to the presence of high content of Fe-rich end member olivine (i.e. fayalite) and increase of iron oxide patche content (Figure (c1,c2)).
4.5. Profile 5: trachytoid and olivine basalts along (B4/B2) contact
This profile is also located at about 50 km south of Al-Madinah city center. Here, magnetic susceptibility was recorded at 5 points (2 m apart from each other) in the east–west direction. An overall width of this profile is about 9 m. Basaltic rocks in this profile have a variation in colour (i.e. from dark to light grey). The major geological phenomenon in this profile is a fissure eruption. The basalts here are generally porphyritic and trachytic in texture. Field observations suggest that felsic rocks here are intruded by the most recent mafic rocks. As shown in Figure , light colour basalt gave higher values of magnetic susceptibility, revealing an enhanced presence of Fe bearing minerals, especially magnetite. The dark colours rock show significantly lower susceptibilities, which may be due to their vesicular texture filled with diamagnetic grains (including calcite and zeolite).
Figure 9. Variation of magnetic susceptibility along profile-5. A photograph of this profile is given on top; Thin section microphotographs of trachytoid basaltic rocks (B4 of [Citation8]) in crossed nicols are given at bottom c1–c6, Tr = Trachytoid and Vb = Vesicular basalt; Symbols as Figure .
![Figure 9. Variation of magnetic susceptibility along profile-5. A photograph of this profile is given on top; Thin section microphotographs of trachytoid basaltic rocks (B4 of [Citation8]) in crossed nicols are given at bottom c1–c6, Tr = Trachytoid and Vb = Vesicular basalt; Symbols as Figure 5.](/cms/asset/86e8b83d-9e7b-4e98-ae0b-a8f53ade1758/tusc_a_1796477_f0009_oc.jpg)
In thin section, two rock types are depicted in the thin sections; the first one is located nearby volcanic cone and has been categorized as vesicular olivine basalt, similar to that given in profil-1; while the second rock type is exhibited as trachytoid basaltic rock (B4 of [Citation8]) that will be given in detail hereunder. The trachytoid basaltic rock units depict that the rock texture varies considerably from glassy to holocrystalline. All the studied samples contain microphenocrysts, and microxenoliths may reach 10 mm in length and cover less than 1% of the rock volume. Olivine megacrysts are more numerous than those of clinopyroxene. Olivine commonly forms well-rounded, resorbed, individual megacrysts up to 6.0 mm long (Figure ), which contain dark-brown inclusions of spinel. The clinopyroxene depicts subpoikilitically around equant olivine in microxenoliths. Such a texture suggests that olivine is of cumulate origin. In the largest microxenoliths, the olivine is slightly more fayalitic than the olivine megacrysts. Moreover, large megacrysts of plagioclase (An57) invariably occur as individual crystals. Their rounded form provides direct evidence of resorption. It is also noticed that some plagioclase phenocrysts are zoned (Figure ) and more sodic than the microphenocrysts of the host rock. Some megacrysts enclose small rounded crystals of relatively iron-rich olivine (Figure ).
Furthermore, fayalitic skeletal olivine grains and euhedral plagioclase crystals (An68) are recorded as microphenocrysts in the groundmass. More crystalline samples have interstitial purple clinopyroxene and titanomagnetite, which clearly began to crystallize later than the plagioclase and olivine microphenocrysts. The Na- rich alkali feldspar sanidines occur as phenocrysts and as prismatic crystals as well as laths in plagioclase groundmass. The opaque mineral patches are recoded in relatively moderate amount, while the mature opaque minerals are less frequently noticed (Figure ). Moreover, an increase in calcite and zeolite-filled amygdales content in the studied thin sections leads to decrease of MS value.
5. Discussion
In strongly magnetic rock units, the susceptibility is controlled mostly by the presence of ferromagnetic minerals [Citation4]. But, in rocks with the lower susceptibility, both paramagnetic and ferromagnetic minerals may control the susceptibility. A recorded wide variation in susceptibility values between the different studied basaltic rocks reflects change in mineral composition, rock texture (i.e. vesicular or amygdaloidal), rock structure (i.e. massive, jointed, fractured, etc.), and type of alteration. For example, in profile-2 (B2 unit of [Citation8]), the magnetic susceptibility is very low due to the increase of orthopyroxene and Mg-olivine content. While in that with iron end member olivine, susceptibility is relatively high (profile-5, B4 unit of [Citation7]). In general, the susceptibility depends on the ratio of Mg to Fe components in olivine. In titanomagnetites, the susceptibility depends on the amount of the titanium in the mineral; generally, it decreases with increasing Ti content. Variation of magnetic susceptibility within the basaltic rocks may also reflect alteration effects on accessory magnetic minerals (e.g. magnetite) as well. It is also noticed that, magnetic susceptibility exhibited lower values in the massive basaltic rocks than the vesicular and amygdaloidal rocks in which the iron oxide minerals filled the voids. Moreover, variation of magnetic susceptibility in the studied basaltic rocks of south Al-Madinah is also related to the presence of disseminated equant opaque minerals, and different Fe oxide patches that were recorded in the studied basaltic rocks. However, the multi-phases of titanomagnetite (viz, Ti-rich and Ti-poor titanomagnetite with some maghemitization) in addition to opaque minerals and patches are the most likely cause of magnetic susceptibility variations (e.g. profile-1; B3 unit of [Citation8] and profile-5; B4 unit of [Citation8]) in the studied basaltic rocks. The highest susceptibility values in profiles 4 and 5 at points 4 and 2, respectively, are probably related to the variation in rock structure and texture and are probably due to the contact effect (see profiles 4 and 5). Furthermore, the Fe end member olivine, disseminated and equant iron oxides as well as Ti-poor titanomagnetite were recorded in a high amount along the contact (see profiles 4 and 5). On the other hand, the lower susceptibility values are caused by Ti- and Mg-rich substitutes. Meanwhile, the presence of diamagnetic and paramagnetic minerals also played some role in reducing the susceptibility values in the studied rocks. As explained in the above profiles, rocks at some locations are clearly affected by weathering and alteration processes (i.e. profile-3; B3 unit of [Citation8] and profile-4; B4 unit of [Citation8]). These processes may have produced maghemitization/hematization, causing a reduction in magnetic susceptibility. The vesicular basaltic rocks in which their voids are barren from any iron oxide minerals and/or filled with diamagnetic minerals (e.g. calcite or zeolite) may reflect low magnetic susceptibility as recorded in profile 3 (points 1, 2, and 3, Figure ) and profile 5 (points 4 and 5, Figure ).
Based on the laboratory measurements of the magnetic susceptibility of the collected basaltic rock units, the most conspicuous observations emerged from the data comparison that was increasing the magnetic susceptibility values in mugearite rock unit (Figure , profile-1, B3 unit of [Citation8]). In this respect, increase of magnetic susceptibility values in these rock units is related to the presence of equant opaque minerals, skeletal fayalitic olivine, and iron oxide patches that are heavily recorded in the mugearite thin sections. Moreover, the lowest magnetic susceptibility values have been recorded in both vesicular and scoriaceous basaltic rocks (Figure ) which are probably due to the effects of diamagnetic minerals (e.g. calcite and zeolite) that filled the rock amygdales (see profile 3, point 1–3 and profile 4, point 1–3 thin sections microphotographs). A comparatively intermediate magnetic values (from ∼400 to 800) have been recorded in hawaiite and trachytoid basaltic rocks due to the decrease in mature opaque mineral and Fe patch content as depicted from the investigation of thin sections. As it turns out, this has further strengthened our confidence in using the magnetic susceptibility in defining the different basaltic rocks in such an environment.
6. Conclusion and recommendations
The basaltic flows and cones, exposed in south Al-Madinah, reveal a significant variation in magnetic susceptibility between the different basaltic types. As per their appearance, the collected basaltic samples are dark in colour and high in density. In addition, they generally occur as aphanitic or fine-grained holocrystalline rocks rich in olivine depicting porphyritic, glomeroporphyritic, vesicular, ophitic, subophitic, dictytaxitic, pilotaxitic, seriate, interstitial, phyric, and trachytic texture. The collected results based on magnetic susceptibility measurements in geological field and laboratory as well as geological observations and thin sections investigations revealed the presence of olivine basalt, vesicular olivine basalt, alkali olivine basalt, hawaiite, mugearite, benmorite, trachyte, trachy-andesite, trachy basalt, and trachytoid pyroclastic rock units in the studied basaltic rocks.
In B3 unit, both mugearite and hawaiite basaltic rocks are Fe-rich and carry the highest susceptibility in profile-1. Magnetite and titano-magnetite are the main opaque minerals, which cause higher magnetic susceptibility in the rocks of this profile. Weathering of the parent material may have produced some alteration in the magnetic mineralogy, causing medium to lower magnetic susceptibility at some points. Profile-2 (B2 unit) represents relatively light colour basaltic rocks. The rocks here have well-developed columnar structures, and have no sign of brecciation and vesicular texture. Significantly, low values of magnetic susceptibility from this profile may reveal the presence of Ti-rich titanomagnetite as opaque mineral along with some paramagnetic and diamagnetic minerals. Brownish colour in the upper part in this profile may indicate weathering-related alteration as well, which may have caused a reduction in magnetic susceptibility. Magnetic susceptibility values of the studied vesicular basaltic rocks in profile-3 (b3 unit) are generally low to medium. The highest susceptibility value at Point 4 is related to the increase of iron oxide patches. In profile-4 (B4 unit), dark colour scoria with increasing vesicular is the cause of relatively low susceptibility values at points 1, 2, and 6. The remaining three points (3, 4, and 5) from amygdaloidal basalts have higher values of susceptibility, most likely due to the presence of Ti-poor titanomagnetite. At profile-5 (B2/ B4 contact), the light coloured trachytoid rocks have higher susceptibility values because of Ti-poor titanomagnetite (e.g. points 1, 2, and 3). Overall, the studied basaltic rock units can be arranged in their magnetic susceptibility descending order as mugearite, trachytoid basaltic rocks, hawaiite, alkali olivine basalt, benmoreite, scoriaceous, and vesicular olivine basalt.
The presence of highly mature opaque crystals presumably points to the presence of sulphide mineralization in the region, thus a detailed geochemical exploration programme is recommended for these rocks to allocate the exact zone of mineralization(s). The detailed geochemical analyses are highly recommended to reveal their chemical element distribution and abundance in these basaltic rocks for uses in industrial sector such as cement production. To document the studied basaltic rocks with further clarity, detailed magnetic, petrographic, and geochemical studies are suggested.
Acknowledgements
Thin-section preparation and the microscopic examinations as well as MS studies are conducted under the umbrella of the Geology Dept., Taibah University. The authors owe special thanks to Abdalhameed Shehata and Meshaal Al-Shaary for their help in sampling, measurements, and thin-section work during their BSc. project. Finally, they thank Dr. Shaya Al Qahtani for his help and support.
Disclosure statement
No potential conflict of interest was reported by the author(s).
References
- Moufti MR, Moghazy AM, Ali KA. Geochemistry and Sr-Nd-Pb isotopic composition of the Harrat Madinah volcanic field, Saudi Arabia. Gondwana Res. 2012;21:670–689. doi: 10.1016/j.gr.2011.06.003
- Moufti MR. The intra-continental Al Madinah volcanic field, western Saudi Arabia: a proposal to establish Harrat Al Madinah as the first volcanic geopark in the Kingdom of Saudi Arabia. Geoheritage. 2013;5(3):185–206. doi: 10.1007/s12371-013-0081-9
- Al-Amri AM, Abdelrahman K, Mellors R, et al. Geothermal potential of Harrat Rahat, northern Arabian shield: geological constraints. Arab J Geosci. 2020;13: Article number: 268.
- Hrouda F, Chlupacova M, Chadima M. The use of magnetic susceptibility of rocks in geologic exploration, case study history. Brno: Teraplus. Geophysical Equipment’s Supplier; 2009. 27 p.
- Cullity BD. Introduction to magnetic materials. Addison-Wesley Publishing Company; 1972. 568 p.
- Dunlop DJ, Özdemir Ö. Rock magnetism: fundamentals and frontiers. Cambridge: Cambridge University Press; 1997. 522 p.
- O’Reilly W. Rock and mineral magnetism. Glasgow: Blackie; 1984. 220 p.
- Pellaton C. Geologic map of the Al Madinah quadrangle, sheet 24D, Kingdom of Saudi Arabia (with text): Saudi Arabian Deputy Ministry for Mineral Resources Geoscience Map GM-52, scale 1:250,000; 1981; 19 p.
- Camp VE, Roobol MJ. The Arabian continental alkali basalt province: part I. Evolution of Harrat Rahat, Kingdom of Saudi Arabia. Geol Soc Am Bull. 1989;101:71–95. doi: 10.1130/0016-7606(1989)101<0071:TACABP>2.3.CO;2
- Murcia H, Nemeth K, Moufi MR, et al. Late Holocene lava flow morphotypes of northern Harrat Rahat, Kingdom of Saudi Arabia: implications for the description of continental lava fields. J Asian Earth Sci. 2014;84:131–145. doi: 10.1016/j.jseaes.2013.10.002
- Moufti MR. The geology of Harrat Al-Madinah volcanic field Harrat Rahat, Saudi Arabia [PhD thesis]. Lancaster: University of Lancaster; 1985. 465 p.
- Camp VE, Roobol MJ. Geologic map of the Cenozoic lava field of Harrat Rahat, Kingdom of Saudi Arabia, Saudi Arabian Directorate General of Mineral Resources Geosciences Map GM-123, scale 1: 250,000 with text. 1991a. 37p.
- Moufti MR, Matsah MI, Soliman MA, et al. Arabian plume dynamics beneath Al-Madinah Al-Munawwarah region and its related geohazards. Final project report (ARP-26-79) submitted to King Abdulaziz City for Science and Technology, Riyadh; 2011. 382 p.
- Moufti MR, Moghazy AM, Ali KA. 40Ar/39Ar geochronology of the Neogene quaternary Harrat Al-Madinah intercontinental volcanic field, Saudi Arabia: implications for duration and migration of volcanic activity. J Asian Earth Sci. 2013;62:253–268. doi: 10.1016/j.jseaes.2012.09.027
- Coleman RG, Gregory RT, Brown GF. Cenozoic volcanic rocks of Saudi Arabia. Saudi Arabian Directorate General of Mineral Resources, Open-file report USGS-OF-03-93; 1983. p. 1–82.
- Gass IG, Mallick DJ. Jebel Khariz an upper Miocene stratovolcano of comenditic affinity on the south Arabian coast. Bull Volcanol. 1968;32:33–88. doi: 10.1007/BF02596586
- Camp VE, Hooper PR, Roobol MJ, et al. The Madinah eruption, Saudi Arabia: magma mixing and simultaneous extrusion of three basaltic chemical types. Bull Volcanology. 1987;49:489–508. doi: 10.1007/BF01245475
- Baker PE, Brosset R, Gass IG, et al. Jebel al Abyad: a recent alkalic volcanic complex in western Saudi Arabia. Lithos. 1973;6:291–314. doi: 10.1016/0024-4937(73)90090-X
- Moufti MR, Németh K, Murcia H, et al. Scientific basis of the geoheritage and geotouristic values of the 641 AD Al Madinah eruption site in the Madinah volcanic field, Kingdom of Saudi Arabia. Open Geol J. 2013b;7:31–44. doi: 10.2174/1874262920130702001
- Dearing J. Environmental magnetic susceptibility using the MS2 system. 2nd ed. Oxon: Bartington Instruments; 1999. 43 p.