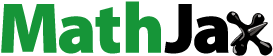
Abstract
Based on commercial bentonite (bento) and two metal precursors, including NiCl2 and CoCl2, diverse series of composites were prepared by incipient wet impregnation. The prepared composites were characterized by X-ray diffraction, scanning electron microscopy and energy-dispersive X-Ray spectroscopy. X-ray diffraction studies show that the crystallite size decreased as increasing the wt. % of cobalt. Moreover, the presence of Co in the EDX spectra for all bento concentrations, suggests its successful incorporation and probably the formation of Ni–Co oxide. The dielectric constant ε′, the dielectric modulus (M′, M′′) and the ac conductivity (σac) were also studied. It was found that ε′ decreases with frequency in all samples, a clear dielectric α relaxation observed from dielectric loss M′′. σac decreases by increasing Co content and the conduction mechanism is correlated barrier hopping (CBH). σac(T) is thermally activated and the activation energy Ea decreased with increasing Co content.
1. Introduction
In the last two decades, there are continuous developments for environment treatment at both academic and industrial scales. Clean alternatives, particularly electricity storage needs to be either stored or to be transformed in other forms of energy to meet actual growing consumer’s needs. Among electronic components, Electrochemical supercapacitors (ECs), or ultracapacitors, can be rapidly charged and discharged and relied upon to store energy reliably for long periods [Citation1]. Some works are focalized on the development of capacitors, principally, how to increase their capacity for energy storage, to be useful for higher requirements. In fact, these trends represent an industrial-commercial challenge where these devices need to be extensively used [Citation2].
Bentonite (bento) is a clay mineral that belongs to the smectite group and consists predominantly of the mineral montmorillonite and an aluminium hydro silicate [Citation3]. Bentonite composition is mainly SiO2 about 70%, with a smaller fraction of Al2O3, circa 15% [Citation4]. It generally has a composition formula as Nax(H2O)4(Al2−xMg0.83)Si4O10(OH)2 [Citation5].
The crystal structure of montmorillonite is layered with two tetrahedral sheets of SiO4 and one octahedral sheet of Al (O, OH) 6 sandwiched between them [Citation3]. Bentonite, with a layer structure containing a larger amount of mesoporous is widespread, easily available, low-cost and not destructive for environment [Citation6]. Bentonite can be used as a catalyst support because of its strong interaction between metal and supports and has many reported applications in the catalytic field [Citation7,Citation8]. Moreover, bentonite indicated a good adsorption efficacy in the heavy metal ion adsorption of industrial water wastes [Citation9]. Also, bentonite is considered to be an inorganic filler for the preparation of alkaline polymer composite with higher conductivity. S. Sang et al. showed that the bentonite has both negative and positive effects on the ionic conductivity of alkaline solid polymer electrolyte [Citation10]. The determination of the electrical conductivity in porous and powder materials is still under investigation due to its complexity.
Since three decades, transition metal oxides used as electrode active materials for ECs have been extensively developed, especially for noble or based metal oxides such as MnO2, NiO, etc. [Citation1]. Duo to pseudo-capacitive behaviour, lower cost and environmental compatibility, these materials have been demonstrated as a good candidate when compared to RuO2known to be the state-of-the-art supercapacitor material [Citation1]. On the other hand, nickel (Ni) is one of the most used elements in the manufacturing process of metallic alloys, coins and batteries [Citation11]. At the solid state, Ni has a face-centred cubic (fcc) crystal structure. Then, at lower temperatures, its electrical resistivity at 20°C is 68.44 nΩ m, which can be neglected. Moreover, nickel is versatile and would form an alloy constituted with most metals [Citation12].
Cobalt (Co) and Ni-based alloys have been studied as prominent engineering coatings due to their improved properties compared to Ni or Co alone [Citation12]. Also, Ni–Co alloy has high mechanical strength, moderate thermal conductivity and outstanding electrocatalytic and magnetic properties [Citation12]. Despite the dielectric properties of different Ni-based composites are widely investigated [Citation13,Citation14], Ni–Co/bentonite gives rise to new types of nanocomposite with original properties. The dielectric properties are considered to be one of the most interesting parameters governing the utilization of Ni–Co/bentonite in innovative research work.
In this work, Wet impregnation methodology was applied to synthesize 1%Co–Ni/bentonite, 3%Co–Ni/bentonite, and 5%Co–Ni/bentonite. The structural and elemental analysis were investigated by using X-ray diffraction (XRD), scanning electron microscopy (SEM) and energy-dispersive X-Ray spectroscopy (EDX) techniques. Our aim is to carry out the structural, and dielectric properties of the synthesized samples by comparison with each other. The effects of Co content on the electrical conductivity and the dielectric loss of Ni/ bentonite are also investigated.
2. Experimental
2.1. Materials and methods
Bentonite and cobalt chloride were obtained from Sigma-Aldrich (USA). Nickel Chloride was purchased from “ASAGGAF-Pharma-HOLYLAND” (production of K.S.A). All chemicals and solvents were used without any purification.
2.2. Composites preparation
The Ni–Co/bentonite nanocomposites were synthesized by wet impregnation process using cobalt chloride and nickel chloride. Several solutions with fixed 5wt. % for nickel chloride and different x wt. % for cobalt chloride (x = 1wt. %, 3wt. % and 5wt. %) were prepared. The preparation method starts with dispersion bentonite in water under continuous stirring. Then, a desired amount of metal salts was dissolved in the previous solution. The mixture was stirred for 3 h at room temperature intensively. The aqueous solution was evaporated to form the mixture as a pale white powder at 393 K for overnight. The obtained composites were marked as pure bentonite (0%Ni–0%Co), 1%Co–Ni/Bento, 3%Co–Ni/Bento and 5%Co–Ni/Bento. The samples were grounded using mortar and pestle to gain a fine powder of pellets. Otherwise, large particles would hinder the pellets to be smooth. The small amount of the fine powder sample (0.30 g) loaded into the hydraulic die-set ket where the pellets being made.
2.3. Characterization techniques
To analyse the phase structure of the coatings, X-ray Diffractometer (JEOL JDX-8030, Tokyo, Japan) was used. Cu Kα radiation of wavelength λ = 0.15418 nm was generated at 40 kV and 30 mA.
The morphology and element analysis of the surface of the composite coatings was observed by Scanning Electron Microscopy (SEM) (JEOL JSM-IT300, Tokyo, Japan), equipped with an energy-dispersive X-ray spectroscopy module (EDS, Oxford, UK), equipped with an energy-dispersive X-ray spectroscopy module (EDS, Oxford, UK)
The dielectric measurements have been made using “Solartron Frequency and Analytical Response analyzer” instrument for measuring the impedance spectra associated with our samples that were in the form of circular pellets, ∼ 1 mm thick and ∼ 0.5 cm in radius. The pellets were connected in series with small 47 Ω resistor to stabilize the signal. The measurement frequency range was f = 0.1 kHz – 0.3 MHz at an AC amplitude of 0.3 V and zero DC voltage in the temperature range 298–358 K. We used copper tapes as the electrodes that were fixed at the pellet with a thin layer of adhesive.
3. Results and discussion
3.1. Characterization of Co based composites
3.1.1 . X- ray diffraction
The XRD patterns of pure bentonite, 1%Co–Ni/bento, 3%Co–Ni/bento and 5%Co–Ni/bento are shown in Figure . Note: all samples except bentonite have 5%Ni and all of them are supported on bentonite. Diffraction peaks were found at 2θ = 22.2°, 26.8° and 36.4°corresponded to the SiO2 (JCPDS card NO. 46-1045), and the diffraction peaks at 2θ = 19.8°, 35.2° and 62.2°, corresponding to the montmorillonite (JCPDS card No. 29-1499), which exhibited the presence of bentonite, similar XRD patterns were observed by Jiang et al. [Citation4,Citation15].
Figure 1. XRD patterns of pure bentonite, 1%Co–Ni/bentonite, 3%Co–Ni/bentonite and 5%Co–Ni/bentonite.
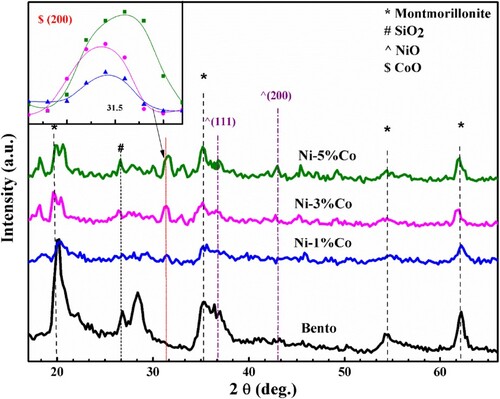
It is clearly seen the intensity of NiO diffraction peaks in the 1%Co–Ni/bento was remarkably weaker than the intensity of NiO diffraction peaks in the 3%Co–Ni/bento and 5%Co–Ni/bento, can be attributed to the low percentage used of these oxides located below the detection limit of the technique. Furthermore, the intensity of CoO diffraction peaks in the 1%Co–Ni/bento was remarkably weaker than the intensity of CoO diffraction peaks in the 3%Co–Ni/bento and 5%Co–Ni/bento, this is due to the increases of Co content.
The XRD pattern of 1%Co–Ni, 3%Co–Ni and 5%Co–Ni was fitted with a Gaussian function. The values of the integral intensity I (area) and the full width at half maximum Δw, peak height and the crystallite size were calculated by Scherrer’s equation of the CoO diffraction peak (200) at ∼ 31.4 were extracted and listed in Table .
Table 1. Variation of the integral intensity I, the width at half maximal intensity Δw and the crystallite size with Co concentration.
It is noted that the crystallite size decreased as increasing the wt. % of cobalt. The mean size of 27.12 nm for 1%Co–Ni/Bento reaches 21.86 nm and 17.35 nm, respectively, for 3%Co–Ni/Bento and 5%Co–Ni/Bento.
3.1.2. Scanning electron microscopy
The SEM images of the pure bentonite, 1%Co–Ni/bento, 3%Co–Ni/bento and 5%Co–Ni/bento are depicted in Figure . Whereas, the Co concentration change is small, its, effects on the morphologies and the composite structures are clearly seen. For all samples, particles are of irregularly spherical geometry and their morphologies did not change compared to pure bentonite. Also, all samples show that Co–Ni nanoparticles were uniformly dispersed on the surface of bentonite.
In Figure (A), pure bentonite presents a regularly shaped bulk and the size of particles are the smallest amongst all doped samples. The 1%Co–Ni/bento (in Figure (B)) shows a big aggregated chunk particle of Co–Ni. From Figure (C), exhibited a smaller Co–Ni particle size compared to 1%Co–Ni/bento and the particle looks more distributed. Figure (D), displays narrower Co–Ni particles than in 1%Co–Ni/bento and 3%Co–Ni/bento but in presences of some chunk particles compared to 3%Co–Ni/bento, which is a good agreement with the XRD results. Moreover, adding more wt.% of Co prohibits the aggregation of the particles.
3.1.3. X-ray energy-dispersive spectrometer (EDX)
The element compositions of pure bentonite, 1%Co–Ni/bento, 3%Co–Ni/bento and 5%Co–Ni/bento were further examined by using the EDX spectra (Figure ).
EDX spectra show clearly peaks for O, Co and Ni, suggesting the successful growth of nickel–cobalt oxide in 3%Co–Ni/bento and 5%Co–Ni/bento. The presence of Co in the EDX spectra of 3%Co–Ni/bento and 5%Co–Ni/bento suggested the incorporation of Co in the previous samples and probably successful formation of Ni–Co oxide. However, in 1%Co–Ni/bento result, the percentage of Co was zero due to the sample actual percentage located below the detection limit of the technique. Furthermore, 3%Co–Ni/bento sample has the highest percentage of O (<45%) while pure bento had the lowest % of O (∼15). On the other hand, pure bento has the highest percentage (∼50%) Si amongst all samples and all other samples show the presence of Si as well which supports the results of XRD data.
3.2. Dielectric properties of Ni-based composites
3.2.1. Dielectric properties of co based composites
We have studied the dielectric properties for (Co)x(Ni/bento) composites in the temperature range 298–358 K and over the frequency range (100 Hz–0.3 MHz).
3.2.2. Dielectric permittivity (ε′)
The variation of the dielectric constant ε′ with frequency for pure bento and (Co)x(Ni/bento) at different temperatures is presented in Figure . The dielectric constant ε′ decreases with increasing frequency in all samples this may be related to the decrease of the dipoles number. Also, it was found that the value of ε′ increases with temperature. This behaviour is due to the fact that by increasing temperatures, leads to decrease in the viscosity of composite which gain sufficient energy to orient easily under the externally applied field effect. Also, the values of ε′ for 1% Co exhibit relatively higher dielectric constant than pure bento which can be attributed to Maxwell–Wegner ionic conduction. The dielectric constant decreases for 3% Co and 5% Co which contributes to the polarization due to the addition of Co [Citation12]. This result could be explained assuming that the Co was masked by bentonite as is clear in SEM result. Another reason for the decrease of polarization with Co content increase might be that the Co has a wider interacting zone with bentonite matrix. In this situation, the surface area effect combined with the resulting reduction in polarization of bentonite lead to the decreases in the internal field. This reduction might be the essential parameter contributing to the decreases of ε′ for (Co)x(Ni/bento) composites.
3.2.3. The electric modulus
The electric modulus can be used to analysis the dielectric behaviour according to complex electric modulus M* which defined as:
(1)
(1) where M′ and M′′ are the real and imaginary parts of the electric modulus, and
(2)
(2)
(3)
(3) The calculated values of M′ at different temperatures as a function of frequency are shown in Figure .
It is clear that M′ decreased with increasing temperature and at low frequency it tends to be zero, indicating the removal of the electrode polarization for the investigated samples owing to thermally activated nature of dielectric constant. In addition, M′ increased with Co content, this behavior is consistent with the behaviour of ε′ versus Co.
The frequency dependence of M′′ for (Co)x(Ni/bento) at different temperatures are displayed in Figure for (1% and 3% of Co content), two peaks appear indicating relaxation processes. The first peak shifts to lower frequency with increasing temperatures and corresponds to α relaxation process, which is attributed to micro-Brownian motion. However, the second peak appearing at high frequency may be attributed to the conductivity current relaxation [Citation13]. On the other hand, no peaks appear for 5% Co content which may be related to the increase of conductivity of the composite sample.
Ac conductivity (σac)
The ac conductivity can be calculated using the following relation:
(4)
(4) In this expression, σt is the total conductivity, σdc is the low-frequency conductivity, ω is the angular frequency,
is the permittivity of the free space and tan δ is loss tangent. The frequency dependence of σac for (Co)x(Ni/bento) composites at different fixed temperatures shown in Figures –.
Figure 7. Variation of log () versus log (f) for (Co)x(Ni/bento) at different temperatures, and the solid line is the fitting according (
.
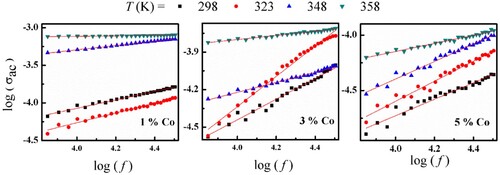
At low frequency the electrical conductivity could be constant which attributed to the dc conductivity, where values are summarized in Table . By increasing frequency, the conductivity increases gradually which indicates that the charge carriers are transported by hopping via defect sites along the chains. This behaviour is due to the increase of the absorbed energy which leads to an increase in the number of the charge carriers that contribute to the conduction process. Also, it is obvious that the conductivity decreased by increasing the Co content, this is due to the distribution of Co allows the formation of conductive three-dimensional networks throughout the composite, which assisting the charge carriers to hop from one cluster to another, as previously reported [Citation14]. The ac conductivity dependence of frequency is given by ( ), where s is the universal exponent factor and B is constant. The s values were calculated from the slope of ln (σac) versus ln (f) and summarized in Table . The values of s are less than unity and decrease with increasing temperature. This means that the correlated barrier hopping (CBH) model is a suitable mechanism to explain the behaviour of the ac conduction for (Co)x(Ni/bento) composites [Citation15]. Here the introduction of Co into (Ni/bento) generates more charge carriers and traps centres which lead to the observed hopping conduction mechanism [Citation16].
Table 2. The frequency exponent, s and dc conductivity for (Co)x(Ni/bento) at different temperatures.
The activation energy (Ea) for (Co)x(Ni/bento) composites were calculated by Arrhenius relation:
(5)
(5) where σo, Ea, k and T are the pre-exponential factor, activation energy, Boltzmann’s constant, and the absolute temperature, respectively. The variation of (ln
) versus (1000/T) are illustrated depicted in Figure . It is shown that
increases with increasing temperature, this is due to the increase in the absorbed energy which enhances the number of charge carriers in the conduction process. The values of
are listed in Table for all investigated samples from f = 1 kHz to 0.2 MHz. The values of
are decreased with increasing frequency and decreases by increasing the Co content in (Co)x(Ni/bento) composites.
4. Conclusion
Different composites were prepared and characterized using bentonite, NiCl2 and CoCl2, by incipient wet impregnation. The results show that the crystallite size is gradually decreased with increasing the wt. % of cobalt. The diameter of these particles of nanometric size is 27.12, 21.86 and 17.35 nm, respectively, for 1%Co–Ni/Bento, 3%Co–Ni/Bento and 5%Co–Ni/Bento. Furthermore, the presence of Co in the EDX spectra for all Ni/bento concentrations supports the Co incorporation in the original matrix and probably the formation of Ni–Co oxide. SEM results displayed that particles in all samples have irregular spherical geometry. By referring to pure bentonite, the resulting morphologies are unchanged in Cox -Ni/bentonite samples. On the other hand, the decrease of both the dielectric constant by adding Co content with increasing frequency, may be due to the decrease of the dipoles number that effectively contributing to the polarization. α – relaxation peak observed from electric modulus M″ proves the preponderance of micro-Brownian motion. The conductivity decreases with increasing the Co content and the conduction mechanism may be correlated barrier hopping. In addition; the temperature dependence of the σac of (Co)x(Ni/bento) undergoes thermally activated behaviour, also activation energy decreases by increasing the Co content.
Figure 8. Variation of (ln) as a function of (1000/T) for (Co)x(Ni/bento) composites at fixed frequencies.
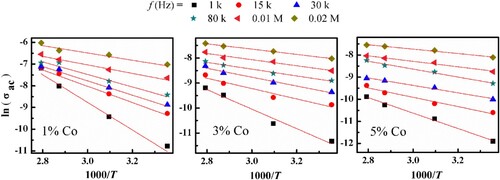
Table 3. Activation energies of (Co)x(Ni/bento) composites according to the Arrhenius relation.
Disclosure statement
No potential conflict of interest was reported by the author(s).
References
- Conway B, Pell W. Double-layer and pseudocapacitance types of electrochemical capacitors and their applications to the development of hybrid devices. J Solid State Electrochem. 2003;7(9):637–644. doi: 10.1007/s10008-003-0395-7
- Deng W, Ji X, Chen Q, et al. Electrochemical capacitors utilising transition metal oxides: an update of recent developments. RSC Adv. 2011;1(7):1171–1178. doi: 10.1039/c1ra00664a
- Saltas V, Vallianatos F, Triantis D. Dielectric properties of non-swelling bentonite: The effect of temperature and water saturation. J Non Cryst Solids. 2008;354(52–54):5533–5541. doi: 10.1016/j.jnoncrysol.2008.03.050
- Jiang Y-X, Xu H-J, Liang D-W, et al. Adsorption of Basic Violet 14 from aqueous solution on bentonite. C R Chim. 2008;11(1–2):125–129. doi: 10.1016/j.crci.2007.04.005
- Sang S, Zhang J, Wu Q, et al. Influences of bentonite on conductivity of composite solid alkaline polymer electrolyte PVA-bentonite-KOH-H2O. Electrochim Acta. 2007;52(25):7315–7321. doi: 10.1016/j.electacta.2007.06.004
- Jeenpadiphat S, Tungasmita DN. Esterification of oleic acid and high acid content palm oil over an acid-activated bentonite catalyst. Appl Clay Sci. 2014;87:272–277. doi: 10.1016/j.clay.2013.11.025
- Li Z, Sun Y, Yang Y, et al. Comparing biochar-and bentonite-supported Fe-based catalysts for selective degradation of antibiotics: Mechanisms and pathway. Environ Res. 2020;183:109156. doi: 10.1016/j.envres.2020.109156
- Amaya J, Suarez N, Moreno A, et al. Mo or W catalysts promoted with Ni or Co supported on modified bentonite for decane hydroconversion. New J Chem. 2020;44(7):2966–2979. doi: 10.1039/C9NJ04878B
- Kakaei S, Khameneh ES, Rezazadeh F, et al. Heavy metal removing by modified bentonite and study of catalytic activity. J Mol Struct. 2020;1199:126989. doi: 10.1016/j.molstruc.2019.126989
- Sang S, Zhang J, Wu Q, et al. Influences of bentonite on conductivity of composite solid alkaline polymer electrolyte PVA-bentonite-KOH-H2O. Electrochim Acta. 2007;52(25):7315–7321. doi: 10.1016/j.electacta.2007.06.004
- Sallam SM, El-Saharty A, Ahmed A-M. Adsorption of toxic Ni (II) from an aqueous solution by bentonite. Int J Ecol Ecobiol. 2017;2(1):158–165.
- Karimzadeh A, Aliofkhazraei M, Walsh FC. A review of electrodeposited Ni-Co alloy and composite coatings: Microstructure, properties and applications. Surf Coat Technol. 2019;372:463–498. doi: 10.1016/j.surfcoat.2019.04.079
- Küçük İ, Vural S, Köytepe S, et al. Synthesis, characterization and dielectric properties of nickel-based polyoxometalate/polyurethane composites. Polymer-Plastics Technology and Materials. 2019;58(13):1445–1460. doi: 10.1080/25740881.2018.1563123
- Kashir A, Jeong H-W, Lee G-H, et al. Dielectric properties of strained nickel oxide thin films. J Korean Phys Soc. 2019;74(10):984–988. doi: 10.3938/jkps.74.984
- Jiang Y, Huang T, Dong L, et al. Ni/bentonite catalysts prepared by solution combustion method for CO2 methanation. Chin J Chem Eng. 2018;26(11):2361–2367. doi: 10.1016/j.cjche.2018.03.029
- Abdel-Baset T.A., Hassen A. Dielectric relaxation analysis and Ac conductivity of polyvinyl alcohol/polyacrylonitrile film. Physica B: Condensed Matter. 2016;499:24–28. DOI: 10.1016/j.physb.2016.07.002