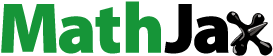
ABSTRACT
C-phycocyanin (C-PC) is a protein–pigment widely used in nutrition and pharmaceutical applications. High purity of C-PC is a limiting factor required for its medical use; hence purity improvement with successful plausible pharmaceutical application is the state of the art of the present work. Highly purified C-PC (A620/A280 = 6.6) was obtained from Thermosynechococcus elongatus. Combined enzymatic cell wall degradation, cellular exposure to high pressure, sonication, and one-time homogenization was found to be an effective purification protocol. Purified complex was confirmed by SDS-PAGE and UV-absorption spectral analysis. Cytotoxic effect of the obtained C-PC was assessed in vitro using a panel of cancer cells. Results showed that MCF-7 cells were most sensitive to C-PC (IC50 = 158.9 µM) compared to Caco-2 (IC50 = 258.3 µM) and HepG2 (IC50 = 277.5 µM) cells. Our findings shed the light on the potential medical use of C-PC isolated from T. elongatus in controlling cancer cells.
1. Introduction
Besides chlorophyll (Chl) a, cyanobacteria have additional effective light-capturing antennae, phycobiliproteins (PBPs), that have an essential role in the energy transfer process to Chl a. Most cyanobacterial species have three protein pigment complexes, C-phycocyanin (C-PC, λmax 610–620 nm), allophycocyanin (APC, λmax 650–655 nm), and phycoerythrin (PE, λmax 540–570 nm) that are covalently linked to chromophores [Citation1]. Phycocyanin is a blue-colored pigment that gains a lot of attention for its use as a food supplement, a fluorescent biomedical marker, as well as in various pharmaceutical applications [Citation2].
Medical and industrial applications of C-PC are dependent upon its purity, where it is always contaminated with other photosynthetic proteins, especially allophycocyanin. The maximum absorbance of C-PC is around 620 nm; hence its purity is commonly evaluated as the absorbance ratio A620nm/A280nm. C-phycocyanin with purity of 0.7 is considered to be of food grade, whereas a purity of 3.9 is deemed to be of reagent grade, and C-PC with a purity of more than 4.0 is of highly pure quality and can be considered as analytical grade [Citation3,Citation4]. Several studies have pointed to the role of C-PC as an antioxidant, anti-cancer, neuroprotective, immunomodulatory, anti-inflammatory agent, as well as a non-toxic photosensitizer in tumour treatment [Citation5–7]. The importance of pure C-PC resides in its anti-proliferative and pro-apoptotic effects on different types of cancer cell lines [Citation8–11], where there are no recorded side effects on normal cells [Citation5,Citation12]. For this reason, the purity of C-PC is considered the main challenge among researchers [Citation3,Citation13]. Although Arthrospira platensis stands to be the main source for industrial C-PC, it has been also reported in other several cyanobacteria, e.g. Calothrix sp. [Citation14], Oscillatoria sp. [Citation15], Phormidium sp. [Citation16], and Synechocystis sp. [Citation17].
Several suggested simplified purification protocols are available that yielded large amounts of C-PC but with quite low purity [Citation18–23]. Lauceri et al. [Citation2] and Muthukumar et al. [Citation21] suggested low cost protocols based on membrane chromatography (MC) that reached a purity ratio of 4.2.
The present work aimed at improving the extraction and isolation procedure of C-PC from Thermosynechococcus elongatus (T. elongatus), hoping to enhance its purity. In addition, the in vitro cytotoxic effect of the purified C-PC was assessed against three cancer cell lines representing the most frequent cancer types in Egypt, including colorectal adenocarcinoma, hepatocellular carcinoma, and mammary gland breast carcinoma, in order to unravel its likely medical applications.
2. Materials and methods
2.1. Cultivation conditions
The unicellular thermophilic T. elongatus was grown in 20 L Blue–Green (BG-11) medium in a 25 L fermenter [Citation24]. After inoculation, cells were exposed to constant white light (80 µE*m−2*s−1) that was increased to 100 µE*m−2*s−1 after 24 h. The culture was incubated at 55°C and 5% (v/v) CO2 flow [Citation25]. Cells were collected at OD750nm = 3.5 and concentrated using Amicon® (DC10 LA) ultrafiltration unit to 2 L followed by centrifugation at 2000g for 15 min. T. elongatus pellets were washed for three times using a buffer containing 20 mM MES (pH 6.5), 10 mM CaCl2, and 10 mM MgCl2.
2.2. C-phycocyanin (C-PC) extraction
The washed T. elongatus cells were suspended in 50 ml buffer containing Lysozyme (20 mM MES, 10 mM CaCl2, 10 mM MgCl2 and 0.2% (w/v) Lysozyme) [Citation26]. The suspension was then stirred in the dark at 37°C for 30 min. After incubation, cells were centrifuged at 2500 g for 15 min at 4°C. Pellets were re-suspended in HEPES buffer, pH 7.5 (20 mM HEPES, 10 mM CaCl2, and 10 mM MgCl2) and exposed to 2000 psi pressure using Parr bomb at 4°C for 30 min.
Subsequently, the suspension was homogenized once using a glass homogenizer followed by a 5 min sonication in an ice bath using FS110D ultrasonic sonicator (Fisher Scientific; Frequency 40 KHz, Ultrasonic power 185W). Crude extract was collected by centrifugation at 3000 g for 15 min at 4°C and sediment was discarded. C-phycocyanin crude extract was precipitated by adding 40% ammonium sulphate in two steps. The precipitated crude extract was re-suspended in 20 ml buffer containing 20 mM HEPES, pH 7.5, 10 mM CaCl2, and 10 mM MgCl2, followed by dialysis against the same buffer for 24 h. Dialysis buffer was refreshed every 2 h for 5 cycles and finally left overnight to remove all existing ammonium sulphate.
2.3. Purification step
The crude extract of C-PC was filtered and loaded onto an ion exchange column (POROS HQ/M column) previously equilibrated by 8 column volumes (CV) of HEPES buffer (20 mM HEPES, pH 7.5, 10 mM CaCl2, and 10 mM MgCl2). After loading the sample, the bound C-PC protein was washed by 6 CV HEPES buffer. Linear gradient of 0–200 mM MgSO4 was carried out with a step at 35 mM at which the purified C-PC was eluted. The change in MgSO4 concentration was monitored via changes in conductivity (mS). Finally, the purified C-PC was dialyzed against HEPES buffer, pH 7.5 for 5 h.
2.4. Lyophilization of C-phycocyanin
To lyophilize the purified C-PC, it was concentrated using Amicon® with molecular weight cut-off (MWCO) of 10,000 Da. The concentrated pure C-PC was then transferred into perforated lids eppendorf tubes and frozen using liquid nitrogen. The frozen phycocyanin was exposed to dark freeze-drying overnight under high vacuum (LYOVAC GT 2, LeyBold-Heraeus) for 18 h. Steps of the C-PC extraction and purification process are summarized in Figure .
2.5. SDS-PAGE analysis
Purified C-PC was fractionated using SDS polyacrylamide gel electrophoresis (SDS-PAGE) according to Schägger and Von Jagow [Citation27]. Accordingly, 4 µl of concentrated pure C-PC were mixed with 4 µl of 60 mM Tris buffer, pH 6.8 containing 2% SDS, 0.01% bromophenol blue, 5% β-Mercaptoethanol, and 10% glycerol. The mixture was then incubated at 65°C for 15 min and loaded on the gel. After electrophoresis, the gel was stained using 0.25% Coomassie Brilliant Blue (R-250) and destained by 30% methanol and 10% acetic acid mixture. PageRuler protein marker ladder (10–250 kDa), purchased from Thermo Scientific was used for determining the molecular weight of C-PC.
2.6. Absorption spectral analysis
For spectroscopic measurements, HEPES buffer (20 mM HEPES, pH 7.5, 10 mM MgCl2, and 10 mM CaCl2) was used for dissolving C-PC. Room temperature absorption spectra were performed in the range of 250–750 nm using Beckman Du7400. The concentration of C-PC was estimated according to the following equation:
2.7. Assessment of cell viability using neutral red uptake (NRU) cytotoxicity assay
The cytotoxic effect of the purified C-PC was assessed in vitro using three distinct human cancer cell lines namely; colorectal adenocarcinoma (Caco-2), hepatocellular carcinoma (HepG2), and the breast cancer cell line (MCF-7) obtained from Vacsera (Dokki, Egypt). The cells were cultured in high glucose (4.5 g/l) DMEM (Lonza Verviers SPRL, Belgium) supplemented with 10% fetal bovine serum (FBS), 100 units/ml penicillin and 100 mg/ml streptomycin, pH 7.4 in 25 cm2 tissue culture flasks (Greiner Bio-One, Germany) at 37°C in a 5% CO2 incubator. Cells from cultures between passages 5–10 were seeded for 48 h in a 96-well plate (Greiner Bio-One, Germany) at a density of 1.5 × 104 cells/well. Different concentrations of the purified C-PC (31.25, 62.5, 125, 250, 500, and 1000 µM) were added in triplicates and incubated for 48 h at 5% CO2 at 37°C. Neutral red (3-amino-7-dimethylamino-2-methyl-phenazine hydrochloride) uptake (NRU) assay was performed based on the procedure outlined by Repetto et al. [Citation29]. After treatment of cell lines, 100 µl neutral red (42 µg/ml) were added and the plates were incubated for 2 h at 37°C. Subsequently, cells were washed and the dye was extracted using 50% acidified ethanol. The absorbance was then measured at 490 nm using a microplate reader (Bio-Rad Laboratories Inc., Hercules, California, US). The proportion of surviving cells was calculated according to the following formula:
where Ablank is the absorbance of medium only, Atreated sample is the absorbance in the presence of different concentrations of C-PC and Acontrol is the absorbance of untreated cells (100% viable), where cell suspensions without any treatment are spiked with their respective culture medium supplemented with 10% FBS. Nonlinear regression and half maximal inhibitory concentration (IC50) calculations were performed using GraphPad Prism 6.0 software (GraphPad Software).
2.8. Statistical analysis
The data were expressed as means ± SD of three independent experiments. Statistical analysis was performed using the GraphPad Prism software, version 6.0. The data were analysed using one-way analysis of variance (ANOVA) test and Tukey’s multiple comparisons post-test. Differences were considered significant at p < 0.05 and highly significant at p < 0.0001.
3. Results
3.1. Purification of C-phycocyanin (C-PC)
High molecular weight impurities were successfully removed from the C-PC crude extract using two-step ammonium sulphate precipitation followed by dialysis. As shown in Figure , the elution profile shows the removal of most protein impurities during the washing step and the pure C-PC was eluted at 35 mM MgSO4 (7.2 mS). The presence of one peak strongly indicates the purity of the extracted C-PC. The presence of a step period at 35 mM MgSO4 helped in good separation between C-PC and allphycocyanin (APC), which was eluted at about 55 mM MgSO4 (8.3 mS).
Figure 2. Purification of C-PC extracted from Thermosynechococcus elongatus by ion exchange chromatography (IEC). Crude extract was diluted by equilibration buffer (20 mM HEPES, 10 mM CaCl2, 10 mM MgCl2) before loading into POROS 50HQ/M column. Gradient of 0–200 mM MgSO4 with 5 CV step at 30% was performed to elute pure C-PC.
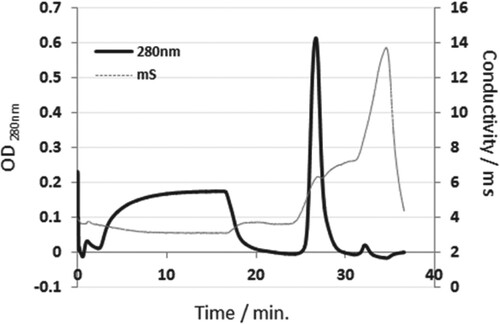
3.2. SDS-PAGE analysis
Results of SDS-PAGE showed low protein impurities in phycocyanin crude extract that were eliminated after chromatographic purification (Figure , Lane 1). Both α and β subunits of C-PC were visualized around 15 and 16.5 kDa for α-phycocyanin and β-phycocyanin, respectively (Figure , Lane 2). Condensed protein subunits, located around 15–18 kDa, point to the presence of some traces of allophycocyanin (18 kDa). Disappearance of all impurities and appearance of two bands only in lane 2 gives additional confirmation for its purity and homogeneity (https://www.uniprot.org/).
3.3. Absorption spectral analysis
C-phycocyanin purity was evaluated through UV-Vis-absorption spectra of crude extract and purified complexes after dialysis of both. High absorbance of crude extract at 619 nm and very small shoulders at 650 and 680 nm reveal low impurities of allophycocyanin, photosystem I and photosystem II. However, pure C-PC exhibited one main high absorption peak at 619 nm without any additional peaks or shoulders. Moreover, the purified phycocyanin showed high A620nm/A280nm ratio that reached 6.6 (Figure ).
3.4. Assessment of cell viability
In the present study, the cytotoxic effect of the purified C-PC was assessed against three types of cancer cell lines, Caco-2, HepG2, and MCF-7 by the NRU cell viability assay. The cytotoxic effect of C-PC was determined based on the concentration that induced half maximal growth inhibition (IC50) of treated cells compared to controls (untreated cells). Caco-2, HepG2, and MCF-7 cells were exposed to various concentrations of C-PC (0, 31.25, 62.5, 125, 250, 500, and 1000 µM) for 48 h. As shown in Figure (A), the cell viability was gradually reduced in a dose-dependent manner in the above-mentioned cell lines. Except for the 31.25 µM concentration which showed no significant difference compared to untreated Caco-2 cells, a highly significant decrease in cell viability (p < 0.0001) was observed in case of all other concentrations of CP-C added to the cells. In case of HepG2 cells, a highly significant dose-dependent decrease in cell viability (p < 0.0001) was observed in comparison with untreated cells. Addition of 31.25 µM of C-PC to MCF-7 cells resulted in a significant decrease (p < 0.05) in viability of cells compared to untreated cells, while a highly significant decrease (p < 0.0001) was observed upon addition of higher concentrations. The IC50 values of C-PC on Caco-2, HepG2, and MCF-7 cells were 258.3, 277.5 and 158.9 µM for 48 h exposure, respectively. Representative morphological changes in C-PC treated cancer cell lines are shown in Figure (B). Microscopic examinations of the untreated cancer (control) cells revealed their characteristic epithelial (Caco-2 and MCF-7) or epithelial-like (HepG2) morphology and prolific growth in monolayers (Figure (B) I, III, & V). Upon treatment with 500 µM C-PC for 48 h, Caco-2 treated cells showed moderate decrease in cell numbers (Figure (B) II). More profound morphological changes were evident in HepG2 and MCF-7 treated cells (Figure (B) IV and VI). The treated cells were rounded up, decreased in size and number, and lost their cell to cell adhesion. Moreover, some of the C-PC treated HepG2 and MCF-7 cells showed membrane blebbing (Figure (B)). The results obtained demonstrated the cytotoxic effect of the purified C-PC on the three studied cancer cell lines.
Figure 5. Cytotoxic effects of C-PC on cancer cell lines. (A) Dose-response curves showing % of cell viability after 48 h incubation of Caco-2, HepG2 and MCF-7 cells against log of different C-PC concentrations. Data are expressed as mean ± SD; (n = 3). (B) Morphological changes induced by C-PC on Caco-2 (I & II), HepG2 (III & IV), and MCF-7 (V & VI) cells. Cells were either untreated (I, III, and V) or treated with 500 µM of C-PC (II, IV, and VI) for 48 h, and photographed under a light microscope (150 ×).
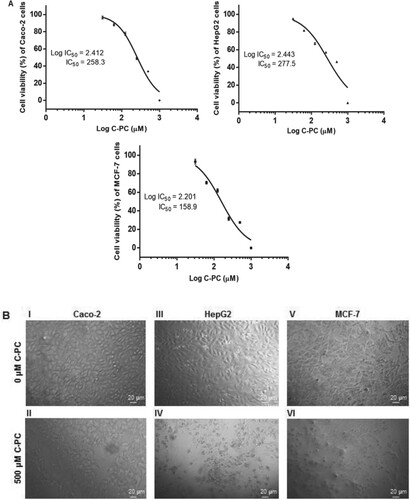
5. Discussion
Although there are several reported protocols concerning isolation and purification of C-PC from various cyanobacterial species, still purification of C-PC from Arthrospira (Spirulina) platensis has great value among researchers for its medical applications [Citation15]. On the contrary to previously reported purification protocols, an improvement in A620/A280 ratio was performed in the present study based on reduction of protein impurities in crude extract via combination of mild isolation and ammonium sulphate precipitation of C-PC [Citation2].
The combination of Lysozyme with 2000 psi and one-time homogenization was found to be a mild and effective isolation strategy. The isolation protocol applied herein came into agreement with that reported by Gupta and Sainis [Citation30] who suggested that enzymatic degradation of Anacystis nidulans cell wall preserved the structure of integral membrane proteins (IMPs). Enzymatic cell wall degradation was recommended also by Santiago-Santos et al. [Citation14]. Moreover, ammonium sulphate precipitation was applied by Kumar et al. [Citation31]. In this study, the combination of mild cell wall degradation, cold sonication, and selective precipitation of C-PC by 40% ammonium sulphate greatly reduced the contamination by other proteins. Reduction of impurities was monitored through chromatographic (IEC) purification, SDS-PAGE, and absorption spectra. For biochemical and medical applications, C-PC is considered pure when the purity ratio (A620/A280) is greater than 4.0. Since the present maximum absorbance of C-PC was 619 nm, a high A619/A280 ratio of 6.6 was achieved, that is considered one of the best reported values. The previously recorded A620/A280 ratio of pure C-PC were 4.15 for Phormidium ceylanicum [Citation17], 4.98 for Spirulina platensis [Citation32], 4.52 for Phormidium fragile [Citation13], 4.85 for mesophilic Synechococcus sp. [Citation33], 5.59 for Spirulina platensis [Citation23], and 6.69 for Spirulina platensis [Citation34].
Over the past few decades, using natural products for the treatment or control of diverse cancerous diseases has acquired great attention [Citation35]. This might be attributed to the fact that chemotherapeutic drugs employed for treatment of cancer are associated with several adverse side effects and short effective half-lives in vivo. Indeed, natural products isolated from cyanobacteria are known for their potent anti-cancer activity [Citation10,Citation12,Citation36]. Phycocyanin is a natural biologically active agent that is isolated and purified from various microalgae and seaweeds [Citation37]. Several studies have highlighted the multiple pharmacological effects of phycocyanin which include antioxidant [Citation38], anti-cancer [Citation39], anti-inflammatory [Citation40], and immunostimulatory effects [Citation41,Citation42].
Previous assessment of the anti-tumour activity of C-PC have been focused on the C-PC isolated mainly from Spirulina platensis [Citation5], in addition to other rich sources including Arthronema africanum [Citation43], Limnothrix sp. [Citation44,Citation45], Porphyra yezoensis [Citation46], and Porphyra haitanensis [Citation47]. To the best of our knowledge, the potential anti-tumour effect of C-PC isolated from T. elongatus remains unexplored. Accordingly, we attempted to unravel one aspect of the biological effects of C-PC isolated from T. elongatus, which is its cytotoxic activity as an initial step on the way of determining its plausible anti-tumour property.
Based on our results, MCF-7 cells were more sensitive to the purified C-PC than Caco-2 and HepG2 cells, where the IC50 of C-PC in MCF-7 (158.9 µM) was less compared to that in case of Caco-2 (258.3 µM) and HepG2 (277.5 µM) cancer cells. Previously, Ravi et al. [Citation12] have reported the sensitivity of the triple negative MDA-MB-231 breast cancer cells to PC (IC50 = 5.98 ± 0.95 µM with PC exposure for 24 h) as compared to other cells including MCF-7. Recently, Safaei et al. [Citation45] have reported the inhibitory effects of a highly purified C-PC isolated from Limnothrix sp. NS01 on the proliferation of human breast cancer cells (MCF-7). The reported IC50 values for 24, 48, and 72 h of exposure were 5.92, 5.66, and 4.52 µg/µl corresponding to 197.3, 188.7, and 150.7 µM, respectively. Formerly, the inhibiting effect of C-PC on cell viability of various human cancer cell lines, encompassing MCF-7 cells, has been previously reported with an effective inhibition exerted on pancreatic cancer cells [Citation48]. Moreover, the inhibitory effects of PC obtained from Spirulina platensis on human ovarian cancer SKOV-3 cells has been previously reported with IC50 values of 216.6 and 163.8 µM for 24 and 48 h exposure, respectively [Citation5].
Several studies have determined the mechanisms underlying the inhibitory effect of C-PC isolated from various rich sources on the growth of different cancer cells. Mainly, cell cycle arrest, induction of apoptosis, induction of autophagic cell death, inhibition of DNA replication, and generation of reactive oxygen species (ROS) were reported among the underlying mechanisms [Citation11,Citation48–50]. However, further clarification of the molecular mechanisms underlying the effect of C-PC on cancer as well as normal cells is mandatory in order to develop an effective strategy and to direct its clinical application [Citation39].
Conclusion
Our study revealed a new method for the purification of C-phycocyanin from the thermophilic Cyanobacterium T. elongatus that led to a highly purified C-PC with A620/A280 ratio of 6.6. Also, to the best of our knowledge, it is the first time that the cytotoxic effect of C-PC from T. elongatus was assessed in vitro against various cancer cell lines representing the most abundant cancer types in Egypt, which are colorectal adenocarcinoma, hepatocellular carcinoma, and mammary gland breast carcinoma. Further in vitro as well as in vivo studies are required to unravel the underlying mechanisms that will shed the light on the potential use of C-PC from T. elongates as an anti-cancer drug.
Disclosure statement
No potential conflict of interest was reported by the author(s).
References
- MacColl R. Cyanobacterial phycobilisomes. J Struct Biol. 1998;124:311–334. doi:10.1006/jsbi.1998.4062.
- Lauceri R, Chini Zittelli G, Maserti B, et al. Purification of phycocyanin from Arthrospira platensis by hydrophobic interaction membrane chromatography. Algal Res. 2018;35:333–340. doi:10.1016/j.algal.2018.09.003.
- Chaiklahan R, Chirasuwan N, Loha V, et al. Separation and purification of phycocyanin from Spirulina sp. using a membrane process. Bioresour Technol. 2011; 102:7159–7164. doi:10.1016/j.biortech.2011.04.067.
- Patil G, Raghavarao KSMS. Aqueous two phase extraction for purification of C-phycocyanin. Biochem Eng J. 2007;34:156–164. doi:10.1016/j.bej.2006.11.026.
- Liu Q, Huang Y, Zhang R, et al. Medical application of Spirulina platensis derived C-phycocyanin. Evid Based Complement Alternat Med. 2016;2016:1–14. doi:10.1155/2016/7803846.
- Wu Q, Liu L, Miron A, et al. The antioxidant, immunomodulatory, and anti-inflammatory activities of Spirulina: an overview. Arch Toxicol. 2016;90:1817–1840. doi:10.1007/s00204-016-1744-5.
- Yu P, Wu Y, Wang G, et al. Purification and bioactivities of phycocyanin. Crit Rev Food Sci Nutr. 2017;57:3840–3849. doi:10.1080/10408398.2016.1167668.
- Bharathiraja S, Seo H, Manivasagan P, et al. In vitro photodynamic effect of phycocyanin against breast cancer cells. Molecules. 2016;21:1470), doi:10.3390/molecules21111470.
- Bingula R, Dupuis C, Pichon C, et al. Study of the effects of betaine and/or C-phycocyanin on the growth of lung cancer A549 cells in vitro and in vivo. J Oncol. 2016;2016:1–11. doi:10.1155/2016/8162952.
- Hao S, Li S, Wang J, et al. C-phycocyanin suppresses the in vitro proliferation and migration of non-small-cell lung cancer cells through reduction of RIPK1/NF-κB activity. Mar Drugs. 2019;17:362), oi:10.3390/md17060362.
- Jiang L, Wang Y, Liu G, et al. C-Phycocyanin exerts anti-cancer effects via the MAPK signaling pathway in MDA-MB-231 cells. Cancer Cell Int. 2018;18:1–14. doi:10.1186/s12935-018-0511-5. doi: 10.1186/s12935-017-0498-3
- Ravi M, Tentu S, Baskar G, et al. Molecular mechanism of anti-cancer activity of phycocyanin in triple-negative breast cancer cells. BMC Cancer. 2015;15:768), doi:10.1186/s12885-015-1784-x.
- Soni B, Kalavadia B, Trivedi U, et al. Extraction, purification and characterization of phycocyanin from Oscillatoria quadripunctulata isolated from the rocky shores of Bet-Dwarka, Gujarat, India. Process Biochem. 2006;41:2017–2023. doi:10.1016/j.procbio.2006.04.018.
- Santiago-Santos MC, Ponce-Noyola T, Olvera-Ram´ırez R, et al. Extraction and purification of phycocyanin from Calothrix sp. Process Biochem. 2004;39:2047–2052. doi:10.1016/j.procbio.2003.10.007.
- Hao S, Yan Y, Li S, et al. The in vitro anti-tumor activity of phycocyanin against non-small cell lung cancer cells. Mar Drugs. 2018;16:178), doi:10.3390/md16060178.
- Singh NK, Parmar A, Madamwar D. Optimization of medium components for increased production of C-phycocyanin from Phormidium ceylanicum and its purification by single step process. Bioresour Technol. 2009;100:1663–1669. doi:10.1016/j.biortech.2008.09.021.
- Ramos A, Acién FG, Fernàndez-Sevilla JM, et al. Development of a process for large-scale purification of C-phycocyanin from Synechocystis aquatilis using expanded bed adsorption chromatography. J Chromatogr B. 2011;879:511–519. doi:10.1016/j.jchromb.2011.01.013.
- Bermejo R, Ramos A. Pilot scale recovery of phycocyanin from Spirulina platensis using expanded bed adsorption chromatography. Chromatographia. 2012;75:195–204. doi:10.1007/s10337-012-2200-1.
- Minkova K, Tchorbadjieva M, Tchernov A, et al. Improved procedure for separation and purification of Arthronema africanum phycobiliproteins. Biotechnol Lett. 2007;29:647–651. doi:10.1007/s10529-006-9274-5.
- Moraes CC, Kalil SJ. Strategy for a protein purification design using C-phycocyanin extract. Bioresour Technol. 2009;100:5312–5317. doi:10.1016/j.biortech.2009.05.026.
- Muthukumar S, Muralikrishnan T, Mendhe R, et al. Economic benefits of membrane chromatography versus packed bed column purification of therapeutic proteins expressed in microbial and mammalian hosts. J Chem Technol Biotechnol. 2017;92:59–68. doi:10.1002/jctb.5064.
- Soni B, Trivedi U, Madamwar D. A novel method of single step hydrophobic interaction chromatography for the purification of phycocyanin from Phormidium fragile and its characterization for antioxidant property. Bioresour Technol. 2008;99:188–194. doi:10.1016/j.biortech.2006.11.010.
- Yan SG, Zhu LP, Su HN, et al. Single step chromatography for simultaneous purification of C-phycocyanin and allophycocyanin with high purity and recovery from Spirulina (Arthrospira) platensis. J Appl Phycol. 2011;23:1–6. doi:10.1007/s10811-010-9525-7.
- Rippka R, Deruelles J, Waterbury JB, et al. Generic assignments, strain histories and properties of pure cultures of cyanobacteria. J Gen Microbiol. 1979;111:1–61. doi:10.1099/00221287-111-1-1.
- Prodöhl A, Ambill M, El-Mohsnawy E, et al. Modular device for hydrogen production: Optimization of (individual) components. In: Miyake J, Igarashi Y, Rögner M, editors. Biohydrogen III. Amsterdam: Elsevier; 2004. p. 171–177.
- El-Mohsnawy E, Kopczak MJ, Schlodder E, et al. Structure and function of intact photosystem 1 monomers from the cyanobacterium Thermosynechococcus elongatus. Biochemistry. 2010;49:4740–4751. doi:10.1021/bi901807p.
- Schägger H, von Jagow G. Tricine-sodium dodecyl sulfate-polyacrylamide gel electrophoresis for the separation of proteins in the range from 1 to 100 kDa. Anal Biochem. 1987;166:368–379. doi:10.1016/0003-2697(87)90587-2.
- Bennett A, Bogorad L. Complementary chromatic adaptation in a filamentous blue-green alga. J Cell Biol. 1973;58:419–435. doi:10.1083/jcb.58.2.419.
- Repetto G, del Peso A, Zurita JL. Neutral red uptake assay for the estimation of cell viability/cytotoxicity. Nat Protoc. 2008;3:1125–1131. doi:10.1038/nprot.2008.75.
- Gupta A, Sainis JK. Isolation of C-phycocyanin from Synechococcus sp., (Anacystis nidulans BD1). J Appl Phycol. 2010;22:231–233. doi:10.1007/s10811-009-9449-2.
- Kumar D, Dhar DW, Pabbi S, et al. Extraction and purification of C-phycocyanin from Spirulina platensis (CCC540). Ind J Plant Physiol. 2014;19:184–188. doi:10.1007/s40502-014-0094-7.
- Uday Bhaskar S, Gopalaswamy G, Raghu R. A simple method for efficient extraction and purification of C-phycocyanin from Spirulina platensis Geitler. Indian J Exp Biol. 2005;43:277–279.
- Abalde J, Betancourt L, Torres E, et al. Purification and characterization of phycocyanin from the marine cyanobacterium Synechococcus sp. IO9201. Plant Sci. 1998;136:109–120. doi:10.1016/S0168-9452(98)00113-7.
- Patil G, Chethana S, Sridevi AS, et al. Method to obtain C-phycocyanin of high purity. J Chromatogr A. 2006;1127:76–81. doi:10.1016/j.chroma.2006.05.073.
- Manna A, Saha P, Sarkar A, et al. Malabaricone-A induces a redox imbalance that mediates apoptosis in U937 cell line. PLoS ONE. 2012;7:e36938), doi:10.1371/journal.pone.0036938.
- Pagels F, Guedes AC, Amaro HM, et al. Phycobiliproteins from cyanobacteria: chemistry and biotechnological applications. Biotechnol Adv. 2019;37:422–443. doi:10.1016/j.biotechadv.2019.02.010.
- de Jesus Raposo MF, de Morais RM, de Morais AM. Health applications of bioactive compounds from marine microalgae. Life Sci. 2013;93:479–486. doi:10.1016/j.lfs.2013.08.002.
- Pleonsil P, Soogarun S, Suwanwong Y. Anti-oxidant activity of holo- and apo-c-phycocyanin and their protective effects on human erythrocytes. Int J Biol Macromol. 2013;60:393–398. doi:10.1016/j.ijbiomac.2013.06.016.
- Jiang L, Wang Y, Yin Q, et al. Phycocyanin: a potential drug for cancer treatment. J Cancer. 2017;8:3416–3429. doi:10.7150/jca.21058.
- Zhu C, Ling Q, Cai Z, et al. Selenium-containing phycocyanin from Se-enriched Spirulina platensis reduces inflammation in dextran sulfate sodium-induced colitis by inhibiting NF-kB activation. J Agric Food Chem. 2016;64:5060–5070. doi:10.1021/acs.jafc.6b01308.
- Chen HW, Yang TS, Chen MJ, et al. Purification and immunomodulating activity of C-phycocyanin from Spirulina platensis cultured using power plant flue gas. Process Biochem. 2014;49:1337–1344. doi:10.1016/j.procbio.2014.05.006.
- Nemoto-Kawamura C, Hirahashi T, Nagai T, et al. Phycocyanin enhances secretary IgA antibody response and suppresses allergic IgE antibody response in mice immunized with antigen-entrapped biodegradable microparticles. J Nutr Sci Vitaminol. 2004;50:129–136. doi:10.3177/jnsv.50.129.
- Gardeva E, Toshkova R, Yossifova L, et al. Antitumor activity of c-phycocyanin from Arthronema africanum (Cyanophyceae). Braz Arch Biol Technol. 2014;57:675–684. doi:10.1590/S1516-89132014005000018.
- Gantar M, Dhandayuthapani S, Rathinavelu A. Phycocyanin induces apoptosis and enhances the effect of topotecan on prostate cell line LNCaP. J Med Food. 2012;15:1091–1095. doi:10.1089/jmf.2012.0123.
- Safaei M, Maleki H, Soleimanpour H, et al. Development of a novel method for the purification of C-phycocyanin pigment from a local cyanobacterial strain Limnothrix sp. NS01 and evaluation of its anticancer properties. Sci Rep. 2019;9:1–16. doi:10.1038/s41598-019-45905-6. doi: 10.1038/s41598-018-37186-2
- Zhang LX, Cai CE, Guo TT, et al. Anti-cancer effects of polysaccharide and phycocyanin from Porphyra yezoensis. J Mar Sci Technol. 2011;19:377–382.
- Yufeng L, Lizhi X, Chengwu Z. The inhibition of phycocyanin from Porphyra haitanensis on the growth of human leukemia HL-60 cells. Chin J Mar Drugs. 2000;19:20–24.
- Liao G, Gao B, Gao Y, et al. Phycocyanin inhibits tumorigenic potential of pancreatic cancer cells: role of apoptosis and autophagy. Sci Rep. 2016;6:34564), doi:10.1038/srep3456. doi: 10.1038/srep34564
- Deniz I, Ozen MO, Yesil-Celiktas O. Supercritical fluid extraction of phycocyanin and investigation of cytotoxicity on human lung cancer cells. J Supercrit Fluids. 2016;108:13–18. doi:10.1016/j.supflu.2015.10.015.
- Pan R, Lu R, Zhang Y, et al. Spirulina phycocyanin induces differential protein expression and apoptosis in SKOV-3 cells. Int J Biol Macromol. 2015;81:951–959. doi:10.1016/j.ijbiomac.2015.09.039.