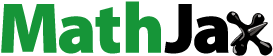
Abstract
The impact of diffusion thermo on transient natural convection flow of a chemically reactive fluid with heat source/sink in a tube is investigated. The Laplace transform technique enables the governing equations to be solved analytically with the solutions inverted by the Riemann-sum approximation method. Constant values of Prandtl number and Schmidt number of 0.71 and 0.75 are used for the study. A time variable ranging from 0.2 to a steady-state, chemical reaction, and heat source/sink parameters ranging from −0.6–0.6, and the Dufour number ranging from 0–0.8 are used for the study. This study reveals that the Dufour number elevates the Nusselt number and the skin friction. Furthermore, it posits that when the Dufour number is small, the temperature heightens with time, and then switches over to decreasing with time when the Dufour number is large. The present model finds application in the pharmaceutical, petroleum, and paint-making industries, etc.
Nomenclature
= | Dimensional fluid concentration | |
= | Specific heat at constant temperature | |
= | Mass diffusion coefficient | |
= | Dufour number. | |
= | Acceleration due to gravity | |
= | Dimensionless heat source/sink | |
= | Dimensional chemical reaction | |
= | Dimensionless chemical reaction | |
= | Buoyancy ratio parameter | |
= | Nusselt number | |
= | Prandtl number | |
= | Heat generation/absorption coefficient | |
= | Radius of tube | |
= | Dimensional radius | |
= | Dimensionless radius | |
= | Schmidt number | |
= | Sherwood number | |
= | Dimensional time | |
= | Dimensionless time | |
= | Dimensional fluid temperature | |
= | Dimensional velocity | |
= | Dimensionless velocity |
Greek symbols
= | Coefficient of thermal diffusion | |
= | Thermal expansion coefficient | |
= | Coefficient of mass expansion | |
= | Dimensionless fluid concentration | |
= | Kinematic viscosity | |
= | Fluid density | |
= | Dimensionless fluid temperature | |
= | Skin-friction |
1. Introduction
Investigations by scholars on the flow of fluids with chemical reactions are due to its usage in many processes such as extraction of petroleum products, nuclear activities, refrigeration, etc. A chemical reaction is either generative when concentration species are created in the fluid or destructive when concentration species of the fluid are destroyed. Ganesan and Rani [Citation1] and Rani et al. [Citation2] showed in their respective research that generative chemical reaction reduces the velocity and concentration and heighten the temperature. Deka and Chaliha [Citation3] reported that chemical reaction decelerates the velocity and accumulates the concentration while Paul and Deka [Citation4] on the other hand discovered that chemical reaction accelerates the velocity. Kumar and Bansal [Citation5], and Kumar and Rizvi [Citation6] showed that chemical reaction dampens the momentum. Chitra and Kavitha [Citation7] discovered that chemical reaction enhances concentration. Arifuzzaman et al. [Citation8] revealed that destructive chemical reaction lowers the concentration. Al-Mamun et al. [Citation9] discovered that for a Casson fluid flow on a stretching sheet, the concentration declines more pronouncedly for destructive than a regenerative chemical reaction. Rana et al. [Citation10] indicated that an exothermic reaction releases heat into a Williamson fluid while an endothermic reaction takes heat out of it. Ferdows and Al-Mdallal [Citation11] presented that the flow profiles are lessened as the chemical reaction improves with the reverse effect observed as the order of the reaction is raised.
The generation or absorption of heat in fluid motion plays a key role in numerous physical processes. These include the cooling problem, combustion process, nuclear waste storage, foodstuffs storage, etc. Barik et al. [Citation12] cogitated how chemical reaction with heat generation affects natural convection MHD flow on a moving vertical plate and revealed the velocity to be unaffected by the heat source. Rehman et al. [Citation13] examined how heat source/sink influences the magnetized Williamson fluid flow indicating that as heat generation heightens the temperature enhances. Dadheech et al. [Citation14] imposed a heat source to study the entropy optimization for all kinds of fluids, Newtonian or non-Newtonian. Gharami et al. [Citation15] employed the moving cylinder to show that a chemical reaction reduces velocity while heat generation/absorption elevates it for the flow of a nano-fluid. Marin et al. [Citation16] investigated the thermal effect of a dipolar body together with its structure and revealed that the temperature reduces to naught outside a bounded domain. Idowu et al. [Citation17] analyzed the effect of heat generation and absorption in MHD Casson fluid natural convection flow and reported that the heat generation/absorption parameter elevates the velocity. Shakir et al. [Citation18] obtained that the movement of electrons actively degrades a composite oxide. References to more studies on the flow of fluids with chemical reactions in presence of a heat source are obtainable in [Citation19–25].
The generation of energy flux that is influenced by composition gradient birth the diffusion thermo effect also known as the Dufour effect which though often neglected comes into prominence when there is a need to separate gas molecules that are heavier from those that are lighter. The diffusion thermo effect (Dufour effect) has been studied alongside the thermal diffusion effect (Soret effect) [Citation25–27]. Observably, some authors have investigated only the Dufour effect on fluid flows either experimentally, numerically, or analytically while playing down the role of the Soret effect to get a good grasp of the behaviour of the flow parameters. Beginning with the early works of Eckert et al. [Citation28] and Sparrow et al. [Citation29], many studies have been conducted on different flow formations and using different geometries. Kumar et al. [Citation30] used a permeable surface that is vertical and semi-infinite to show that the diffusion thermo effect uplifts the temperature and velocity. Vedavathi et al. [Citation31] studied the chemically reactive MHD Casson fluid flow over a vertical plate as it is affected in particular by the Dufour effect and heat source/sink. Sharmilaa and Kaleeswari [Citation32] used plates to investigate the Dufour effect in their study and showed that an increase in the Dufour number raises the temperature and lowers the velocity profiles. Ganesh et al. [Citation33] employed a circular wavy enclosure that is partially heated to investigate the flow of a Casson fluid. Reza-E-Rabbi et al. [Citation34, Citation35] revealed that for fluid flow on a stretching sheet, a rapid suppression of the concentration occurs with a rise in the chemical reactions while the Dufour number boosts the temperature of the fluid. The Dufour number lowers velocity and concentration but heightens the temperature as discovered in studies like Rani and Kim [Citation36], and Rani and Reddy [Citation37] whereas in El-Kabeir and Chamkha [Citation38], the Dufour number increases concentration and decreases temperature.
This study aims to present a semi-analytical, numerical as well as analytical investigation of the diffusion thermo effect on a transient free-convective flow of a chemically reactive binary fluid with heat source/sink through a tube having symmetric boundary conditions. The authors carefully surveyed the existing literature and were unable to find a study like the present. This study has applications in the pharmaceutical industry, petroleum industry, paint making industry, etc.
2. Mathematical analysis
A tube that is vertical and infinite having radius is being considered where the axial
and radial
axes are taken respectively vertically and horizontally to the axis of the tube. A fluid that is viscous and incompressible flows inside the tube. Both the fluid and tube have identical temperatures
and concentrations
initially
. At the time
, the wall of the tube has its temperature and concentration changed to
and
respectively (See ). Due to fully developed conditions, the flow is time-dependent and in the radial direction
. There is a heat source or sink expressed linearly in the form
that is dependent on the temperature which
is defined as a heat source term when it is less than zero and as a heat sink term when it is greater than zero. There is also a chemical reaction in the fluid which is of the first order and homogenous. The mass fluxes are also assumed to influence the thermal fluxes giving rise to the diffusion thermo or Dufour effect. The equations of motion in vector form are:
The continuity equation is
(1)
(1) The momentum equation is
(2)
(2) The energy equation is
(3)
(3) The concentration equation is
(4)
(4) With the assumptions above and the Boussinesq approximation, the flow model is [Citation4, Citation39]:
(5)
(5)
(6)
(6)
(7)
(7)
In equations (6) and (7), and
are respectively the heat source/sink and chemical reaction terms which assume a negative value for the heat source and generative chemical reaction, a positive value for heat sink and destructive chemical reaction, and zero for the absence of heat source/sink and chemical reaction.
The following are the flow conditions initially and at the boundary [Citation39]:
(8)
(8) Using the following quantities which are non-dimensional [Citation4]:
(9)
(9) (5)–(8) reduces to:
(10)
(10)
(11)
(11)
(12)
(12)
(13)
(13)
The above conditions indicate that the fluid flow is symmetrical about the axis of the tube.
The nomenclature defines all notations.
The initial conditions help achieve a simplified version of equations (10) – (12) by the Laplace transform as:
(14)
(14)
(15)
(15)
(16)
(16)
Using equation (16), equation (15) can be simplified further as:
(17)
(17) The Laplace transform of (8) is given as:
(18)
(18)
3. Solutions
By taking ,
, and
with
as the Laplace parameter, the solutions in the Laplace domain are thus given below as:
3.1. Concentration
From equation (16), the concentration is realized as:
(19)
(19)
3.2. Temperature
Using equation (19) in (17), the concentration is obtained as:
(20)
(20)
3.3. Velocity
By the use of (19) and (20) in (14), the velocity is thus obtained as
(21)
(21)
3.4. Rate of heat and mass transfer, and shear stress
From equations (19)-(21), the rate of heat and mass transfers expressed as Nusselt and Sherwood numbers and shear stress are respectively obtained on the inner surface of the tube as:
(22)
(22)
(23)
(23)
(24)
(24)
3.5. Inversion of the transforms
The Laplace inversion of equations (19) to (24) is complex and difficult to carry out, thus the Riemann-sum approximation which is a numerical procedure comes in handy for the inversion. The procedure is explicitly laid out by Khadrawi and Al-Nimr [Citation40] and Jha and Danjuma [Citation41]. The inversion is effected as follows:
(25)
(25) Here,
represents the velocity
, the temperature,
and the concentration
in equations (19), (20), and (21) respectively. Furthermore, it represents
,
and
in (22)-(24).
3.6. Validation
To confirm the accuracy of the method used in the present work, equations (10)-(12) are solved by the numerical programme of MATLAB PDEPE, and the results are found to be in good agreement with the results of the Riemann sum approximation method as revealed in Table . Furthermore, the steady-state version of the governing equations is derived and solved analytically. This is accomplished by setting in equations (6)-(8) to zero to produce governing equations that are independent of time as follows:
(26)
(26)
(27)
(27)
(28)
(28)
Table 1. Comparative values of velocity for the Riemann sum approximation method (RSA), PDEPE, and the exact solution (ES) .
Solving equations (26)-(28) give the steady-state concentration, temperature, and velocity respectively as:
(29)
(29)
(30)
(30)
(31)
(31) The steady-state solutions are presented in Table . A comparative view of the numerical solutions at a large value of time with the steady-state solutions reveals a good agreement. Finally, the results of a special case
of the work of Anurag and Singh [Citation39] are compared with the results of the present work when
in Table and are shown to agree. Figure .
4. Discussion of results
Analytical-cum-numerical solutions are presented to probe the influence of the diffusion thermo effect on the time-dependent natural convection of a chemically reactive binary fluid flow with a heat source/sink in a tube. The default parametric values of and
are chosen for this discussion. For other default values, time
varies from 0.2 to steady-state, Dufour number
varies from 0 to 0.8, chemical reaction
and heat source/sink
varies from −0.6–0.6 while the buoyancy ratio
varies from −4 to 4. For the entire discussion, the value chosen for
is 0.75 which Gebhart and Pera [Citation42] presented as the Schmidt number for a mixture of oxygen in air and Prandtl number of air
are used. Figures – capture a vivid behaviour of the concentration, temperature, and velocity as affected by time, chemical reaction, Dufour number, heat source/sink, and buoyancy ratio. Table exhibits the observable reactions of the shear stress, rate of heat, and mass transfers to the flow parameters.
Table 2. Numerical comparison of velocity with Anurag and Singh [Citation36].
Figures depict the effect of altering on
,
and
respectively. Both are raised to a steady state with time.
Figures and respectively show the consequence of varying and
on the temperature and velocity at a particular point in the tube
with other parameters fixed as displayed in the figures. Figure posits that for small values of the Dufour number, the temperature accumulates with time and then switches over to diminishing with time for large values of the Dufour number. The value of the Dufour number for the switching over varies as to whether the fluid is undergoing internal heating with either generative or destructive chemical reaction or internal cooling with either generative or destructive chemical reaction. Physically, a small Dufour number indicates the thermal buoyancy force to be lower than the solutal buoyancy force which gives the temperature the capacity to elevate as time is hiked while a large Dufour number specifies that the thermal buoyancy force is higher than the solutal buoyancy force making the temperature to diminish as more time is gained. Moreover, the temperature is observed to enhance with a rise in the Dufour number. In Figure , the switching over observed for the temperature in Figure does not appear, rather a progressive growth in the velocity is manifested as both time and Dufour number are raised. This unveils the fact that the diffusion thermo effect has an important role in the heating or the cooling of the fluid and can be used to raise or lower the fluid temperature without affecting the fluid velocity.
Figures present how chemical reaction affects concentration, temperature, and velocity respectively. The destructive chemical reaction diminishes the concentration (Figure ) leading to an elevation of the temperature (Figure ) which does not lead to a rise in the velocity but a decline (Figure ). The physical interpretation is that a hike in the destructive chemical reaction excites disturbances that motivate molecular motion, raising the temperature and lowering the concentration. Also, since the chemical reaction has an inverse proportion to the kinematic viscosity, an increase in the destructive chemical reaction indicates a decrease in the kinematic viscosity that decreases the velocity. The generative chemical reaction yields the reverse phenomenon.
Figures – unveil how the heat source/sink impacts the temperature and velocity respectively. Figure unveils that the heat source elevates the temperature leading to an enhancement of the convection current thereby accelerating the velocity as seen in Figure . Increasing heat sink reverses the phenomenon observed with the heat source. Physically speaking, intensifying the heat source generates heat in the fluid which raises the temperature that accelerates the fluid motion, whereas enhancing the heat sink discharges heat from the fluid which decelerates the fluid motion.
Figure explains how the buoyancy ratio impacts the velocity. It reveals that when temperature and concentration support each other with the temperature dominating the flow the velocity is advanced while the case of the temperature and velocity opposing each other with the concentration dominating the flow
ushers in a deceleration of the velocity.
Computed values of the Nusselt and Sherwood numbers and shear stress are displayed in Table . The Table reveals that as time increases, the slopes of the temperature and concentration on the wall of the tube are lowered hence a fall in the Nusselt and Sherwood numbers respectively while the slope of the velocity steepens thus enhancing the shear stress. Also, a rise in the Dufour number elevates the slopes of the temperature and velocity leading to a built-up of the Nusselt number and shear stress respectively. Additionally, a boost in heat sink dwindles the Nusselt number and shear stress whereas a boost in heat source rises the Nusselt number and shear stress. Furthermore, growth in destructive chemical reactions enhances the Nusselt number and diminishes the Sherwood number and shear stress with the reverse phenomenon observed for generative chemical reactions.
Table 3. Nusselt number, Sherwood number, and Skin-friction for the binary mixture of air and oxygen
.
5. Conclusion
The transient free convective fluid flow influenced by chemical reaction, having a heat source/sink and diffusion thermo effect has been solved semi-analytically by the use of the Laplace transform technique and Riemann-sum approximation method. The study has examined the influence of the flow parameters and reached the following important conclusions:
Temperature and concentration accumulate and velocity accelerates with time until they gain a steady state.
It is concluded that temperature heightens with time for small values of the Dufour number, and then switches over to decreasing with time for large values of the Dufour number. Also, temperature and velocity are observed to rise with the increase in the Dufour number.
A generative chemical reaction elevates the concentration and velocity but lowers the temperature while a destructive chemical reaction does the opposite due to a decrease in the kinematic viscosity.
The heat source enhances temperature and velocity while the heat sink inhibits the flow behaviour which is a direct consequence of the accumulation of heat due to the intensity of the heat source and the reduction of heat due to the intensity of the heat sink.
Nusselt number heightens as the heat source, Dufour number, and destructive chemical reaction are raised while it declines as time, heat sink, and generative chemical reaction are raised.
Sherwood number diminishes with time and destructive chemical reaction while increasing with generative chemical reaction.
Shear stress strengthens with time, Dufour number, heat source, and generative chemical reaction but weakens with time, heat sink, and destructive chemical reaction.
Disclosure statement
No potential conflict of interest was reported by the author(s).
References
- Ganesan P, Rani HP. On diffusion of chemically reactive species in convective flow along a vertical cylinder. Chem Eng Process. 2000;39:93–105.
- Rani HP, Reddy GJ, Kim CN. Transient analysis of diffusive chemical reactive species for couple stress fluid flow over vertical cylinder. Appl Math Mech -Engl Ed. 2013;34(8):985–1000.
- Paul A, Deka RK. Chemical Reaction Effect on Transient free convective flow past an infinite moving vertical cylinder. Int J Chem Eng. 2013;2013(2):1–9.
- Deka RK, Chaliha A. Effects of mass transfer and heat flux on convective flow past a Moving Vertical Cylinder with chemical reaction. Int J Appl Phys Math. 2012;2(4):273–275.
- Kumar G, Bansal A. Unsteady flow past on vertical cylinder in the presence of an inclined magnetic field and chemical reaction. An. univ., eftimie murgu resita. Fasc Ing. 2019;26(1):107–118.
- Kumar G, Rizvi SMK. Casson fluid flow past on vertical cylinder in the presence of chemical reaction and magnetic field. App Appl Math: An Int J (AAM). 2021;16(1):524–537.
- Chitra M, Kavitha V. Effect of chemical reaction on MHD flow of fluid through a vertical pipe filled with porous medium. AIP Conf Proc. 2020; 030019;2261(1):1–10.
- Arifuzzaman SM, Khan MS, Al-Mamun A, et al. Hydrodynamic stability and heat and mass transfer flow analysis of MHD radiative fourth-grade fluid through porous plate with chemical reaction. J King Saud Univ Sci. 2019;31:1388–1398.
- Al-Mamun A, Arifuzzaman SM, Reza-E-Rabbi S, et al. Numerical simulation of periodic MHD casson nanofluid flow through porous stretching sheet. SN Appl Sci. 2021;3(2):1–14.
- Rana BMJ, Arifuzzaman SM, Islam S, et al. Swimming of microbes in blood flow of nano-bioconvective Williamson fluid. Therm Sci Eng Prog. 2021;25(101018):1–17.
- Ferdows M, Al-Mdallal QM. Effects of order of chemical reaction on a Boundary Layer flow with heat and mass transfer over a Linearly stretching sheet. Am J Fluid Dyn. 2012;2(6):89–94.
- Barik RN, Dash GC, Rath PK. Chemical reaction and heat source effects on MHD flow of viscoelastic fluid past an exponentially accelerated vertical plate embedded in a porous medium. AMSE J Model B. 2015;84(2):80–104.
- Rehman KU, Al-Mdallalb QM, Malik MY. Symmetry analysis on thermally magnetized fluid flow regime with heat source/sink. Case Studies Therm Eng. 2019;14(100452):1–10.
- Dadheech PK, Agrawal P, Sharma A, et al. Entropy analysis for radiative inclined MHD slip flow with heat source in porous medium for two different fluids. Case Studies Therm Eng. 2021;28(101491):1–13.
- Gharami PP, Reza-E-Rabbi S, Arifuzzaman SM, et al. MHD effect on unsteady flow of tangent hyperbolic nano-fluid past a moving cylinder with chemical reaction. SN Appl Sci. 2020;2(7):1–16.
- Marin M, Othman MIA, Seadawy AR, et al. A domain of influence in the moore–gibson–thompson theory of dipolar bodies. J Taibah Univ Sci. 2020;14(1):653–660. doi:10.1080/16583655.2020.1763664.
- Idowu AS, Akolade MT, Abubakar JU, et al. MHD free convective heat and mass transfer flow of dissipative Casson fluid with variable viscosity and thermal conductivity effects. J Taibah Univ Sci. 2020;14(1):851–862. doi:10.1080/16583655.2020.1781431.
- Shakir I, Haider S, Agboola PO, et al. Fabrication of NiO/SnO2 heterojunction based photocatalyst for efficient sunlight degradation of organic dyes. J Taibah Univ Sci. 2021;15(1):656–665. doi:10.1080/16583655.2021.1996135.
- Sravanthi CS, Gorla RSR. Effects of heat source/sink and chemical reaction on MHD Maxwell nanofluid flow over a convectively heated exponentially stretching sheet using homotopy analysis method Int. J Appl Mech Eng. 2018;23(1):137–159.
- Thiagarajan M, Kumar MD. Heat source/sink and chemical reaction Effects on MHD and heat transfer flow of radiative nanofluid over a porous exponentially stretching sheet with viscous dissipation and ohmic heating. Int J Basic Sci Appl Comp (IJBSAC). 2019;2(7):5–12.
- Akram J, Akbarb NS, Marajc E. Chemical reaction and heat source/sink effect on magnetonano prandtl-eyring fluid peristaltic propulsion in an inclined symmetric channel. Chin J Phy. 2020;65:300–313.
- Dawar A, Shah Z, Kumam P, et al. Chemically reactive MHD micropolar nanofluid flow with velocity slips and variable heat source/sink. Sci Rep. 2020;10(20926):1–23.
- Irfan M, Khan M, Khan WA. Heat sink/source and chemical reaction in stagnation point flow of Maxwell nanofluid. Appl Phys A. 2020;126(11);892:1–8.
- Appidi L, Pramod Kumar P. Chemical reaction and heat source Effects on MHD free convective flow over A Linearly accelerated Moving Vertical porous plate. Indian J Sci Tech. 2021;14(13):1044–1055.
- El-Kabeir SMM, Chamkha AJ, Rashad AM, et al. Soret and Dufour effects on heat and mass transfer by non-darcy natural convection from a permeable sphere embedded in a high porosity medium with chemically-reactive species. Int J Energy Tech. 2010;2:1–10.
- Salleh SNA, Bachok N, Md Arifin N, et al. Influence of Soret and Dufour on forced convection flow towards a moving thin needle considering buongiorno’s nanofluid model. Alexandria Eng J. 2020;59:3897–3906.
- Waini I, Ishak A, Pop I. Dufour and Soret effects on Al2O3-water nanofluid flow over a moving thin needle: tiwari and Das model. Int J Num Meth Heat Fluid Flow. 2020;31(3):766–782.
- Eckert ERG, Jurewicz LS, Tewfik OE. Diffusion-Thermo Effects on Heat Transfer from a cylinder in cross flow. AIAA J. 1963;1(7):1537–1543.
- Sparrow EM, Scott CJ, Forstrom RJ, et al. Experiments on the diffusion thermo effect in a binary boundary Layer With injection of various gases. ASME J Heat Transf. 1965;87(3):321–327.
- Kumar RVMSSK, Kumar AGV, Raju MC, et al. Dufour effect On MHD Free Convection flow of chemically reactive and Radiation absorption fluid past a vertical permeable moving plate with variable suction. Math Theory Mod. 2015;5(6):143–160.
- Vedavathi N, Dharmaiah G, Balamurugan KS, et al. Chemical reaction, Radiation and Dufour Effects on Casson magneto Hydro dynamics fluid flow over A vertical plate with heat source/sink. Global J Pure Appl Math. 2016;12(1):191–200.
- Sharmilaa KP, Kaleeswari SP. Dufour effects on unsteady MHD free convection and mass transfer flow past through a porous medium in a slip regime with heat source/sink. Int J Sci Eng Appl Sci (IJSEAS). 2015;1(6):307–320.
- Ganesh NV, Kalaivanan R, Al-Mdallal QM, et al. Buoyancy driven second-grade nano boundary layers over a catalytic surface with reaction rate, heat of reaction and activation energy at boundary. Case Studies Therm Eng. 2021;28(101346):1–14.
- Reza-E-Rabbi S, Arifuzzaman SM, Sarkar T, et al. Explicit finite-difference analysis of an unsteady MHD flow of a chemically reacting Casson fluid past a stretching sheet with Brownian motion and thermophoresis effects. J King Saud Univ Sci. 2020;32:690–701.
- Reza-E-Rabbi S, Ahmmed SF, Arifuzzaman SM, et al. Computational modeling of multiphase fluid flow behavior over a stretching sheet in the presence of nanoparticles. Eng Sci Tech An Int J. 2020;23:605–617.
- Rani HP, Kim CN. A numerical study of the Dufour and Soret effects on unsteady natural convection flow past an isothermal vertical cylinder. Korean J Chem Eng. 2009;26(4):946–954.
- Rani HP, Reddy GJ. Soret and Dufour Effects on Transient double diffusive free convection of couple-stress fluid past a vertical cylinder. J Appl Fluid Mech. 2013;6(4):545–554.
- El-Kabeir SMM, Chamkha AJ. Heat and mass transfer by mixed convection from a vertical slender cylinder with chemical reaction and Soret and Dufour effects. Heat Transf Asian Res. 2013;42(7):618–629.
- Singh AK. Role of heat source/sink in transient free convective flow through a vertical cylinder filled with a permeable medium: An analytical approach. Heat Transf. 2021;50(4):3154–3175.
- Khadrawi AF, Al-Nimr MA. Unsteady natural convection fluid flow in a vertical microchannel under the effect of the dual-phase-Lag heat-conduction model. Int J Thermophys. 2007;28(4):1387–1400.
- Jha BK, Danjuma YJ. Transient generalized taylor-couette flow: a semi-analytical approach. J Taibah Univ Sci. 2020;14(1):445–453. doi:10.1080/16583655.2020.1745477.
- Gebhart B, Pera L. The nature of vertical natural convection flows resulting from the combined buoyancy effects of thermal and mass diffusion. Int J Heat Mass Transf. 1971;14:2025–2050.