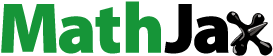
Abstract
This investigation peruses the features of temperature and mass transport of the non-Newtonian third-order liquid over an absorbent convective temperature shrinking sheet. The sheet is packed with silver nanoparticles. The Buongiornos modelling uses a particular non-Newtonian third-order liquid with the Brownian movement and the thermophoresis consequences using the non-linear radiation. The non-linear partial differential equations are changed to the ordinary differential equations with similarity transformations. The changed system of equations is then resolved using the numerical Shooting method and the sixth-order Runge-Kutta’s method. The numerically obtained solutions of the velocity profiles, temperature distributions, and concentrations of nanoparticles are discussed graphically. Also, the non-Newtonian parameter reduced the velocity of the liquid, increased the temperature and the concentration profiles throughout the fluid. Ultimately, the qualified systematic analysis is built by the preceding study in restrictive cases and displaingy the best correlation.
1. Introduction
The flows of the non-Newtonian fluids are increasing considered due to their various applications in manufacturing and industrial procedures. Examples of non-Newtonian fluids are molten plastic, blood, ketchup, grease, artificial fibre, paint certain oily liquids etc. These fluids violate Newton’s law of viscosity. They are extremely glutinous and expose their elasticity. They are essential in composite process, polymer depolarization, absorptions of bubbles, boiling points, etc. The second-grade fluids exhibit the effects of the natural stress and cannot predict the shear thin and thick phenomenon. But a model of the third-order liquids can predict together the natural stresses and the shear thin and thick phenomenon evenly, and the constitutive governing equations have more complexity.
In the taxonomy, the third-grade fluid, a secondary type of non-Newtonian fluid, is confined to the non-Newtonian impacts. These fluids are shear thin and thick and natural stress components and they have rigid boundaries. They exhibit glutinous elastic features. The second-order fluid exhibits normal stress effects and does not show the shear thinning and thickening phenomenon. Furthermore, the equations of flow in the third-grade fluids are more difficult than the consequent equations in the second-grade fluids. The non-Newtonian fluid along with temperature and mass transport is significantly very important for paper production and lubrication with the grease industry, in addition, due to those considerable practical applications.
The solutions of the specific third-grade liquid towards the stagnation points of the unstable absorbent stretched and/or shrinking surfaces were obtained by Naganthran et al. [Citation1]. The increment of the thermally conductance of the liquid through scattering nanoparticles was studied by Masuda et al. [Citation2]. Buongiorno [Citation3] found that the Brownian movement and thermophoresis impacts of nanofluids incremented fluid’s thermal conductivity. The computational resolutions of nanofluids over the stretched sheets were obtained by Khan and Pop [Citation4] using Buongiornos modelling and analysing the Brownian movement along with the thermophoretic impacts on the temperature transportation rates at the surface. Makinde and Aziz [Citation5] studied the temperature transportation in the nanofluids past the stretched sheets to represent convection frontier conditions. Makinde and Aziz [Citation6] inspected MHD combined convective frontier layered movement and warm transport past the warmed perpendicular plate engrossed in the permeable media. The frontier layered movements by the temperature transportations towards the warmed absorbent stretched surfaces were explored computationally by Ishak [Citation7]. Yao et al. [Citation8] examined a systematic resolution for the frontier layered movement over the convective warmed porous stretched and/or shrinking walls. Rahman et al. [Citation9] deliberated the combined convection flows over the perpendicular smooth plate by representing convective frontier conditions. Mustafa et al. [Citation10] explored the Maxwells fluids past the warmed exponential stretched surfaces engrossed in the nanofluids. Khan et al. [Citation11] considered the effects of non-linearly radiation on MHD flows of Carreau’s liquids over the non-linearly stretched surfaces by the convection frontier conditions.
Zaib et al. [Citation12] studied the impacts of the nanoparticle on the flows of the specially non-Newtonians third-grade liquids past the absorbent warmed shrinking sheets by the non-linear radiation. More recently, Krishna and Chamkha [Citation13] explored the diffusion thermo, radiating absorption and, Hall and ion slips impacts on MHD free convection rotating flow of nanoliquids over the semi-infinite porous stirring plate by the invariable temperature sources. Krishna and Chamkha [Citation14] considered the MHD squeezing flows of the H2O-supported nanoliquid during the saturated permeable media concerning two equivalent discs, appying the Hall currents to descriptions. Hall and ion slip consequences on uneven MHD convective revolving flows of the nanoliquids were investigated by Krishna and Chamkha [Citation15]. Krishna [Citation16] researched the stable MHD convection flows of the gelatinous nanoliquid due to the porous exponential stretched absorbent surfaces. Krishna et al. [Citation17] conferred the radiation and absorption on the MHD convection flows of nanoliquids over a vertically travelling porous plate. Krishna et al. [Citation18] explored unsteady radiating MHD flow of the Casson hybrid nanoliquid past an infinite exponentially accelerated vertical absorbent surface. Ahammad and Krishna [Citation19] discussed the chemically reacting, Soret and Dufour consequences on MHD natural convective revolving flow through a perpendicular permeable channel.
Prakash et al. [Citation20] conducted theoretical study of laminar, stable, noncompressible bio-convection flows of tangential hyperbolically non-Newtonian nanofluids from the two path-stretching surfaces underneath a reciprocally, orthogonally and electrically magnetic field. Tripathi et al. [Citation21] explored the computational modelling approaches to describe the peristaltic pumping of the couple stresses hybrid nanofluid standardized by the electro-osmosis system in the micro-channel. The consequences of the boron-nitride nanotube suspensions on the heat transfer performances of ethylene-glycol based nanofluid flow across the micro-channel/duct driven by peristaltically pumping and electro-osmotic pumping has been researched by Akram et al. [Citation22]. Akram et al. [Citation23] explored the peristaltic synchronized electro-osmotic pumping of H2O-supported hybrid Silver–Gold nanofluid in an inclined asymmetrical micro-fluidic duct/channel into an absorbent medium. Akram et al. [Citation24] examined hybrid Ag-Al2O3 nanofluids and Al2O3 mono-nanofluids in the convection temperature transport processes determined by electro-osmotis and peristaltic pumping. Prakash et al. [Citation25] explored the electro-osmotic flows of hybrid nanoliquids in an asymmetrically micro-channel. This moved sinusoidally by invariable wave velocity underneath axial electrical fields and Joule heating. The consequences of the dissimilar categories of supporting fluid on carbon nanotube nanofluid flow past a spherical stretching sheet have been explored by Akbar et al. [Citation26]. The influences of Coriolis body forces, electro-magneto hydro-dynamics and thermally radiative temperature transport on the Cassons hybrid-assorted convective nanofluids determined by an exponential accelerating plate adjoining to an absorbent media in the revolving structure have been explored by Tripathi et al. [Citation27].
Khan et al. [Citation28] explored a three-dimensional revolving flow of H2O-supported nanofluid originated from infinitely revolving discs. Turkyilmazoglu [Citation29] examined the interactions of overhanging particles by the fluid past a stretchable gyratory disc. The visible consequences of Buongiornos nanofluids modelling on the pace of temperature and accumulation transport in dissimilar fluids movement geometry have been conferred by Turkyilmazoglu [Citation30]. Anuar et al. [Citation31] examined the upshots of MHD on the sturdy 2D combined convective movement induced with non-linear surfaces in carbon nanotubes. Turkyilmazoglu [Citation32] researched the frontier layered flows of movement due to a revolution with stretchable and/or shrinkable bendable cone in an immobile fluid.
The mutual consequences of MHD temperature transport flow underneath the influences of slips past an affecting smooth plate with the effect of entropy have been scrutinized by Ellahi et al. [Citation34]. Bhatti et al. [Citation35] explored the electro-osmotic MHD movement of non-Newtonian Jeffrey fluids in petite particles affecting the sinusoidal format in a Darcy Brinkmans Forchheimers medium.
The frontier layered movement of the particular non-Newtonian third-order liquid over the convection heated shrinking sheets by the non-linear heat radiating due to solar energies and magnetic effect with the numerical solutions, generated by the Mathematica 10.0 computational software, has not yet been investigated. Therefore, the present investigation was to consider the frontier layered movement of the particular non-Newtonian third-order liquid over the convection heated shrinking sheets by the non-linear heat radiating due to solar energies and magnetic effect. The Buongiornos modelling includes the Brownian movement and the thermophoretic effects for the third-grade liquid. The renovated non-linear equations were resolved computationally utilizing the Shooting methodology. To the best of our knowledge, this specific problem on the third-grad fluids has not been measured before; therefore, the description results are innovative and original.
2. Formulation and solution of the problem
The present investigation scrutinizes the features of temperature and mass transport of the particular non-Newtonian third-order liquid over an absorbent convective temperature shrinking sheet packed with the nanoparticles. The Buongiornos modelling is used for the particular non-Newtonian third-order liquid. This included the Brownian movement and the thermophoretic consequences of non-linear radiating. The physical configuration of the problem is displayed in Figure .
It is assumed that the -direction is taken by the side of the shrinking sheet and the
-direction vertical to it. The shrinking surface velocity is
for
. The lower surfaces of the sheet are warmed convectively by the temperature
, which delivers the temperature transport coefficient hf. An invariable magnetic domain by the strength
is applied on the transversal flow path.
and
are the heat and nanoparticle concentration at a large distance from the surface, respectively. The physical calculations , governing the steady flow were written by (Naganthran et al. [Citation1] and Mustafa et al. [Citation10]):
(1)
(1)
(2)
(2)
(3)
(3)
(4)
(4)
The relevant frontier stipulations were
(5)
(5) where u and v are the velocity constituents in the x and y axis, respectively.
is the non-Newtonian constraint,
is the magnetic parameter,
is the free stream temperature,
is the convective fluid, temperature,
is the concentration near the surfaces,
is the concentration of nanoparticle,
is the heat diffusivity,
is the kinematic viscosity,
is the density of the liquid,
is the electrical conductivity,
is the ratio of effectively heat capacity of the nanoparticle materials to the heat capacity of the fluid,
is the thermal conductivity,
is the Brownian diffusion coefficient and
is the thermophoresis diffusion coefficient,
is the specific heat capacitance of nanofluid.
is radiative temperature discharge. Radiative temperature discharge
via Rosselands approximations might be set into the following format
(6)
(6) where
is the Stefan–Boltzmann’s invariable and
is the coefficient of the mean absorption.
Using the following transformation
(7)
(7) Here
is the temperature ratio parameter. Later, using equation (7), equation (1) was satisfied identically and equations (2), (3), in addition to (4) consider the subsequent form as
(9)
(9)
(10)
(10)
(11)
(11)
Revised boundary conditions
(12)
(12) where
is the magnetic parameter,
is the thermal convective parameter,
is the suction parameter,
is the dimensionless Non-Newtonian parameter,
is the Prandtl number,
is the thermal radiation parameters
is the Lewis number,
is the Brownian motion parameter,
is the thermophoresis parameter.
The significant physical variables of interest were the local skin frictions coefficient, the locally Nusselts number and the locally Sherwoods number were designated as
(13)
(13) where
was the shear stress in the x-axis ((Naganthran et al. [Citation1]),
was the temperature flux and
is the mass discharge specified by
(14)
(14)
Using (7), we obtained as
(15)
(15) where
the Reynolds quantity.
3. Numerical solution of the problem
Yang and Zhang [Citation33] explored the existence and multiple resolutions for a non-linear system with the pertinent parameters involved in the boundary value problem. Now the reduced sets of non-linear ordinary differential equations from (9) to (11) mutually by the frontier conditions (12) are resolved computationally by the Nachtsheim-Swigert Shooting iterations methodology simultaneously by the Runge-Kutta’s sixth-order iteration technique. The numerical solutions to the non-dimensional boundary value problem are generated by the Mathematica 10.0 computational software. The relative error tolerance to was deemed for the convergence, and the step size was
.
For computation, the thermo-physical characteristics of the supporting fluid (water) and Silver utilized for program code validation, are given in Table .
Table 1. Thermophysical properties of water and Ag nanoparticles.
Table 2. Skin frictions, Nusselts number and Sherwoods number.
4. Results and discussion
The current research systematically found the frontier layered movement by the temperature and accumulation transport of the third-order liquid by the non-linear heat radiation and magnetic domain effect. The renovated ordinary differential equalities are computationally resolved by the Shooting technique for various quantities of the significant constraints.
The analysis of computational results for dissimilar physically restrictions connecting into a problem were explored by the graphs. The velocities, temperature distributions, and concentrations of nanoparticles for the distinct quantities of are depicted in Figures . Figure displays that the velocity profiles are decreased by the enhancing quantities of
. Therefore, the momentum boundary layer thickness decreased. The magnetic domain is vertical towards the movement paths. An inclination on or attraction to produce the resistances is called Lorentzs force. It shows the propensity to refuses to go together with the flow field throughout the fluids media. In contrast, the temperatures distributions and concentrations of nanoparticles are increased by the magnetic parameters
, as displayed in Figures and . As a result, the thermal and concentration of frontier layers are increased.
The velocities, temperature delivery, and the concentrations of nanoparticles for dissimilar quantities of the dimensionless non-Newtonians parameters are shown in Figures . Figure displays that the velocity profiles are decreased by increasing the quantities of
. Therefore, the momentum frontier layered thickness increased. In contrast, the temperature distributions and the concentrations of nanoparticles are increased by the
, as represented in Figures and , and therefore, the heat and concentrations frontier layers are augmented throughout the fluid region. This was also explored from those figures that the velocities, temperature delivery, and concentrations of nanoparticles were the largest for the particular third-grad liquid, as evaluated by the Newtonian fluid (
= 0).
Figure displays due to the growth of quantities of the Brownian movement parameter, the temperature profiles are increased. But, the contradictory behaviour was detected for the concentrations profile, as shown in Figure . Hence, the thermally frontier layered thicknesses increase, whereas the concentrations of frontier layered thicknesses decrease. The kinetic energy of nanoparticles increases because the strength of the chaotic movement and the temperature of the fluid increase. The Brownians movement at the nanoscales and the molecular level are significant mechanisms of the nanoscale levels that govern the thermal behaviour. In systems using nanofluid, the Brownian movement has occurred since the sizes of nanoparticle might change the characteristics of the warm transport. As the scale sizes of the particles approached the scales of the nano-meters, the particle Brownian movement and this result on the neighbouring liquids play very important roles in the temperature transport features.
The effects of the convective parameter on the temperatures delivery and concentrations of nanoparticles are inspected in Figures and , respectively. Figure shows that by increasing the values of
due to the stronger convection warming near the surfaces, the temperature gradients near the surfaces of the sheets are increasing. It allowed the temperature effects to penetrate cavernous into the sluggish fluids. Hence, the temperatures delivery and the thermally frontier stratum thicknesses increase by an increase in in the value of
. Figure displays that the concentrations of nanoparticles and the concentrations frontier stratum are increased by the largest quantities of
.
Figure displays the cross-flow of the temperature with the increase of . Figure shows that the concentration profiles are decreased by an augment in the Lewis number
. Hence, the thermal and concentration frontier layer thickness became thin for the numerical solution. Figures and depict the outcomes of the thermophoretic restriction
on the temperatures delivery and the concentrations of nanoparticles. These figures show that the temperatures profiles and the concentrations of nanoparticles are increased by an enhancement in
. It is since the diffusion penetrated deeply in the fluid due to an increasing quantity of
which reasons the thickness of the thermal boundary layer and the concentration boundary layer stratum.
The Skin frictions and Nusselts numbers, in addition, to the Sherwoods numbers, are evaluated with reference to the governing parameters and are tabulated in Table . The skin frictions are increased by an enhancement in Hartmann number and retard with non-Newtonian fluid parameters. The local Nusselts quantity and Sherwoods numbers are increased with the growth in thermophoresis parameter, thermal convective parameter, Lewis number and thermal radiation parameter. These are also reduced with escalating in Hartmann number, non-Newtonian parameter, Brownian motion parameter and Prandtl number. Consequently, the third-order liquid accelerated the frontier layered partitions with increasing Non-Newtonian parameter.
Table compares the outcomes. Here, the heat and mass transport of the third-order liquid over an absorbent convective temperature shrinking sheet packed with the Ag nanoparticles together with the Brownian movement and the thermophoresis impacts the non-linear radiating have been discussed comfortably. These results are the best concurrence by the existing outcomes of Makinde and Aziz [Citation5] and Zaib et al. [Citation12]. The current developments describe the features and the applications of non-Newtonian liquids in tribology and automotive industries, and so on. Lubricating oil in the machines is recurrently tested for gumminess since it could affect the performance of oils. Therefore, it influence the lifetime of the equipment. As oil utilized for elongated duration and still being utilize that factors together with defect particles as well as soot from unfinished combustion can cause them to takes on further non-Newtonian attributes even at the lowest shear rate. Thus, connected parameters engaged in addition suitable situations needed to be apply and handle to controls the non-Newtonian behaviour of the oils.
Table 3. Comparisons of outcomes for and
(
).
5. Conclusions
The boundary layered flows with the heat and mass transportation of the third-order liquid by non-linear heat radiating and magnetic field impacts have been explored. The subsequent terminations are preserved as follows.
The velocity deliveries are decreased , but temperature and concentration distributions are increased for magnetic field parameters.
The temperature distributions and concentration distributions are increased by an enhancement in Brownian motion and convective parameters.
The temperature distributions and concentration distributions are increased for the thermophoresis parameter.
The temperature distribution increases and concentration distributions are decreased by an increase in the Lewis parameter.
The current consequences might be utilized for displaying the non-Newtonian features of second- and third grade-fluid in addition to the relevance in the engineering field, automobile industries, etc.
Therefore, the implicated physical constraints and appropriate circumstances, for instance, shrinking , stretching sheets, etc. required to be handled and useful to organize the non-Newtonian behaviours of the oils.
Disclosure statement
No potential conflict of interest was reported by the author(s).
References
- Naganthran K, Nazar R, Pop I. Unsteady stagnation-point flow and heat transfer of a special third-grade fluid past a permeable stretching/shrinking sheet. Sci Rep. 2016;6:1–13.
- Masuda H, Teramae AEK, Hishinuma N. Alteration of thermal conductivity and viscosity of liquid by dispersing ultrane particles. Netsu Bussei. 1993;7:227–233.
- Buongiorno J. Convective transport in nanofluids. ASME J Heat Transfer. 2006;128:240–250.
- Khan WA, Pop I. Boundary-layer flow of a nanofluid past a stretching sheet. Int J Heat Mass Transfer. 2010;53:2477–2483.
- Makinde OD, Aziz A. Boundary layer flow of a nanofluid pasta stretching sheet with a convective boundary condition. Int J Therm Sci. 2011;50:1326–1332.
- Makinde OD, Aziz A. MHD mixed convection from a vertical plate embedded in a porous medium with a convective boundary condition. Int J Therm Sci. 2010;49:1813–1820.
- Ishak A. Similarity solutions for flow and heat transfer over apermeable surface with convective boundary condition. Appl Math Comput. 2014;217:837–842.
- Yao S, Fang T, Zhong Y. Heat transfer of a generalized stretching/shrinking wall problem with convective boundary conditions. Commun Nonlinear Sci Numer Simul. 2011;16:752–760.
- Rahman MM, Merkin JH, Pop I. Mixed convection boundary layer flow past a vertical flat plate with a convective boundary condition. Acta Mech. 2015;226:2441–2460.
- Mustafa M, Khan JA, Hayat T, et al. Simulations for Maxwell fluid flow past a convectively heated exponentially stretching sheet with nanoparticles. AIP Adv. 2015;5:037133.
- Khan M, Hashim M, Hussain M, et al. Magnetohydrodynamic flow of Carreau fluid over a convectively heated surface in the presence of non-linear radiation. J. Magn. Mater. 2016;412:63–68.
- Zaib A, Chamkha AJ, Rashidi MM, et al. Impact of nanoparticles on the flow of a special non-Newtonian third-grade fluid over a porous heated shrinking sheet with nonlinear radiation. Nonlinear Eng. 2018;7(2):103–111.
- Krishna MV, Chamkha AJ. Hall and ion slip effects on MHD rotating boundary layer flow of nanofluid past an infinite vertical plate embedded in a porous medium. Results Phys. 2019;15:102652.
- Krishna MV, Chamkha AJ. Hall effects on MHD squeezing flow of a water based nano fluid between two parallel disks. J Porous Media. 2019;22(2):209–223.
- Krishna MV, Chamkha AJ. Hall and ion slip effects on Unsteady MHD convective rotating flow of nanofluids – application in biomedical engineering. J Egyptian Math Soc. 2020;28(1):1–14.
- Krishna MV. Heat transport on steady MHD flow of copper and alumina nanofluids past a stretching porous surface. Heat Transfer. 2020;49(3):1374–1385.
- Krishna MV, Ahamad NA, Chamkha AJ. Radiation absorption on MHD convective flow of nanofluids through vertically travelling absorbent plate. Ain Shams Eng J. 2021;12:3043–3056.
- Krishna MV, Ahammad NA, Chamkha AJ. Radiative MHD flow of Casson hybrid nanofluid over an infinite exponentially accelerated vertical porous surface. Case Stud Thermal Eng. 2021;27:101229.
- Ahammad NA, Krishna MV. Numerical investigation of chemical reaction. Soret and dufour impacts on MHD free convective gyrating flow through a vertical porous channel. Case Stud Thermal Eng. 2021;28:101571.
- Prakash J, Tripathi D, Akkurt N, et al. Tangent hyperbolic non-Newtonian radiative bioconvection nanofluid flow from a bi-directional stretching surface with electro-magneto-hydrodynamic Joule heating and modified diffusion effects. Eur Phys J Plus. 2022;137:472.
- Tripathi D, Prakash J, Reddy MG, et al. Numerical study of electro-osmosis induced alterations in peristaltic pumping of couple stress hybrid nanofluids through microchannel. Ind J Phys. 2021;95:2411–2421.
- Akram J, Akbar NS, Tripathi D. Thermal analysis on MHD flow of ethylene glycol-based BNNTs nanofluids via peristaltically induced electroosmotic pumping in a curved microchannel. Arab J Sci Eng. 2022;47:7487–7503. doi:10.1007/s13369-021-06173-7.
- Akram J, Akbar NS, Tripathi DA. Theoretical investigation on the heat transfer ability of water-based hybrid (Ag–Au) nanofluids and Ag nanofluids flow driven by electroosmotic pumping through a microchannel. Arab J Sci Eng. 2021;46:2911–2927.
- Akram J, Akbar NS, Tripathi DA. Comparative study on ethylene glycol based Ag-Al2O3 and Al2O3 nanofluids flow driven by electroosmotic and peristaltic pumping: a nano-coolant for radiators. Phys Scr. 2020;99(11):115208.
- Prakash J, Tripathi D, Bég OA. Comparative study of hybrid nanofluids in microchannel slip flow induced by electroosmosis and peristalsis. Appl Nanosci. 2020;10:1693–1706.
- Akbar NS, Tripathi DA, Khan ZH. Numerical simulation of nanoparticles with variable viscosity over a stretching sheet. Numer Simul Eng Sci. 2018;1:1–22.
- Tripathi D, Prakash J, Beg OA, et al. EMHD Casson hybrid nanofluid flow over an exponentially accelerated rotating porous surface. J Porous Media. 2022;25:1–16. doi:10.1615/JPorMedia.2022041050.
- Khan JA, Mustafa M, Hayat T, et al. Numerical study of nanofluid flow and heat transfer over a rotating disk using Buongiorno's model. Int J Numer Methods Heat Fluid Flow. 2017;27(1):221–234.
- Turkyilmazoglu M. Suspension of dust particles over a stretchable rotating disk and two-phase heat transfer. Int J Multiphase Flow. 2020;127:103260.
- Turkyilmazoglu M. On the transparent effects of Buongiorno nanofluid model on heat and mass transfer. Eur Phys J Plus. 2021;136:376.
- Anuar NS, Bachok N, Turkyilmazoglu M, et al. Analytical and stability analysis of MHD flow past a nonlinearly deforming vertical surface in carbon nanotubes. Alexandria Eng J. 2020;59(1):497–507.
- Turkyilmazoglu M. A note on the induced flow and heat transfer due to a deforming cone rotating in a quiescent fluid. J Heat Transfer. 2018;140(12):124502.
- Yang Y, Zhang J. Existence and multiple solutions for a nonlinear system with a parameter. Nonlinear Anal. 2008;70:2542–2548.
- Ellahi R, Alamri SZ, Basit A, et al. Effects of MHD and slip on heat transfer boundary layer flow over a moving plate based on specific entropy generation. Journal of Taibah University for Science. 2018;12(4):476–482.
- Bhatti MM, Zeeshan A, Bashir F, et al. Sinusoidal motion of small particles through a Darcy-Brinkman-Forchheimer microchannel filled with non-Newtonian fluid under electro-osmotic forces. J Taibah Univ Sci. 2021;15(1):514–529.