Abstract
This investigation aimed to assess the anti-Parkinson's effect of butin in rotenone-treated rats. A total of 24 albino Wistar rats were segregated into four groups equally as follows: Group I (normal) and Group II (rotenone control); Group III and Group IV; were butin 25 and 50 mg/kg/p.o. Daily, 1 h post offer mentioned treatments, Group I animals were injected with sunflower oil (s.c.), and Group II-IV were treated subcutaneously with 2 mg/kg rotenone emulsion in sunflower oil for 28 days. On day 29, i.e. 24 h after the rotenone dose, animals were evaluated for behavioural and biochemical parameters i.e. acetylcholine-esterase, paraoxonase-1, butyrylcholinesterase, endogenous antioxidants (Glutathione, catalase, superoxide-dismutase), oxidative, nitrative stress and proinflammatory markers (Interleukin-6, IL-1β, and TNF-α). Butin attenuated the rotenone-induced catalepsy, akinesia, memory loss and decreased learning ability. Butin treatment shows rotenone-induced neurobehavioral impairments, oxidative and nitrative stress, and neuroinflammatory markers reversed the enzyme activities towards normal.
GRAPHICAL ABSTRACT
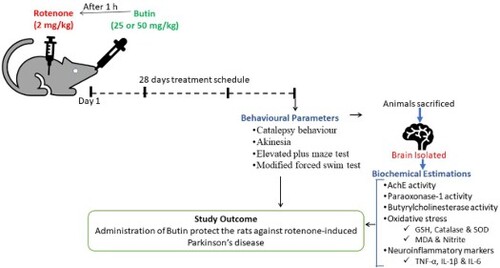
1. Introduction
The commonly seen neurodegenerative disease, Parkinson’s disease (PD) causes progressive deterioration that can be postponed but not entirely stopped by a cure [Citation1]. Weakness in upper and lower extremities tremors, slow movements, and a loss of balance are all symptoms. It is caused by dopaminergic nigrostriatal system neurodegeneration, resulting in striatum dopamine (DA) insufficiency [Citation2]. The latter’s pathophysiology is connected to drug-induced alterations in the motor circuit of basal ganglia as a result of dopaminergic neuron death [Citation3]. The tyrosine hydroxylase containing neuronal loss in the striatum reduces dopamine synthesis. Tremor bradykinesia, stiffness, and postural instability result as a result of this condition [Citation4].
PD etiology is thought to be linked to numerous environmental variables as well as genetic abnormalities [Citation5]. Consumption of herbicides and pesticides polluted water, and neurotoxins such as rotenone are all suggested environmental variables linked to the etiology of PD. In both sporadic and hereditary instances of PD, oxidative in sulthas been proposed as a similar underlying fundamental cause. Cellular dysfunction as a result of this [Citation6]. The oxidative stress degenerate neurons which secrets dopamine in substantia nigra pars compacta. Inflammation of neurons synthesizes reactive oxygen species (ROS), mitochondrial malfunction, and dopamine metabolism are considered to be the initiators of these cascades of events involved with the formation of oxidative stress in dopamine synthesizing neuronal cells [Citation7]. Dopamine replacement medication is now used to treat Parkinson’s disease (PD), although long-term usage can produce motor fluctuations and dyskinesias, as well as increased free radical production, which can hasten neuronal degeneration in certain PD patients. Levodopa/carbidopa does not provide the same level of neuroprotection in rodents. Several treatments have been tested for their beneficial effect on the onset of PD or for delaying the degradation of dopaminergic neurons, but none has proven to be effective [Citation8].
Despite advancements in PD management such as its prevention, diagnosis, and treatment, a lack of community knowledge and attitudes may play a major role in restricting access to proper management options. Despite significant research, the processes of sporadic PD development remain unclear. Elevated oxidative stress, inflammatory response, and dysfunction of mitochondria are all neurochemicals implicated in the pathophysiology of PD [Citation9,Citation10]. Many medicines are now used to treat Parkinson’s disease, but their usage is restricted due to serious adverse effects [Citation8]. Hence, harmonizing and alternative therapy is critical in the management of PD. Clinical methods to natural chemicals are being investigated in this context for the creation of a new medication that can prevent nigrostriatal system degeneration in adults.
Butin (7,3′,4′-trihydroxydihydro flavone) was discovered in a plant. A number of therapeutic plants, including Dalbergia odorifera, Adenanthera pavanina, and Vernonia anthelmintica Willd [Citation11–13]. Butin was evaluated for its protective action in H2O2-induced damage to cells [Citation14], DNA oxidative damage [Citation15,Citation16], mitochondrial dysfunction, and apoptosis [Citation16,Citation17] caused by oxidative stress were decreased, according to research. More recently butin was reported to possess a protective effect against 3-nitropropionic acid induced Huntington’s disease in rats [Citation18] and complete Freund’s adjuvant-induced arthritic rats [Citation19]. To our knowledge, only a few researchers have looked into the mechanism behind butin’s protective qualities in mitochondria.
2. Material and methods
2.1. Chemicals and reagents
Butin (≥99% purity) was provided a gift sample by SRL, India. Rotenone and thiobarbituric acid (TBA), and reduced glutathione (GSH) (Sigma, USA) were used in the study. The analytical kits for tumor-necrosis-factor-α (TNF-α) Interleukin 1β (IL-1 β), and Interleukin 6 (IL-6) were analyzed by use of a commercially available rat enzyme-linked immunosorbent assay kit (Sigma, USA). All used chemicals were of standard quality.
2.2. Animals
Wistar rats (150–200 g) used in the study were housed at standard controlled conditions according to Institutional Research Board guidelines. The rats had full access to the normal pellets and ad libitum water. The experimental procedure was conducted after the official permission of the Institutional Animal Ethical Committee (IAEC/TRS/PT/021/005).
2.3. Experimental design
Butin was diluted with 0.5% sodium carboxymethyl cellulose (SCMC) solution and given to experimental animals orally for 28 days. Two doses of butin (25 and 50 mg/kg) were selected from acute oral toxicity studies and previously published reports [Citation18,Citation19]. Rotenone was emulsified in sunflower oil and administered to rats (2 mg/kg) subcutaneously to induce neurotoxicity [Citation20].
A total of 24 Wistar rats were segregated equally into four different groups and considered as: Group I and Group II; normal (positive) and rotenone control (negative) were orally given 1 ml of 0.5% SCMC solution; Group III and Group IV; were served as test groups and orally administered with butin (25 and 50 mg/kg).
Daily 1 h after the offer mentioned treatments, Group I animals were injected with sunflower oil (s.c.), and animals from group II–IV were treated with 2 mg/kg of rotenone (s.c.) emulsion in sunflower oil. This treatment schedule was followed daily for 28 days.
On day 29, i.e. 24 h after the last dose of rotenone treatment, rats were evaluated for catalepsy, akinesia, elevated-plus-maze-test (EPM), and modified-forced-swim-test (FST). Then, rats were euthanized, brain was removed for biochemical analysis such as acetylcholine esterase (AChE), paraoxonase-1 (PON-1), butyrylcholinesterase (BChE), oxidative (malondialdehyde-MDA), endogenous antioxidants (reduced-glutathione-GSH, catalase, and superoxide-dismutase-SOD), and nitrative (nitrite) stress markers and neuroinflammatory indicators, i.e. IL 1β, TNF α, and IL 6.
2.4. Behavioural parameters
2.4.1. Catalepsy
The bar test was used to measure catalepsy. The catalepsy test was performed as per the protocol reported in a published protocol [Citation8]. In this test, three consecutive trials were conducted to calculate the length of time it took the animals to withdraw one of the forelimbs from a high bar (9 cm) and in a half-raising position. For the bar test, the maximum cut-off time was set at 180 s.
2.4.2. Akinesia
The difficulty in beginning movement in PD is replicated in this test. Akinesia in the animals was measured by watching their latency in seconds while they moved all four limbs, and the test was completed within 180 s. Each rat was acclimatized for 5 min on a hardwood raised (100 cm) platform (100 cm 150 cm) before the akinesia test. The time taken by rat to move limbs was measured with a stopwatch [Citation21].
2.4.3. Elevated-plus-maze-test
The abilities of memory and learning were tested by EPM. The equipment is made up of equal dimensions (50 cm × 10 cm) of two closed and two open arms crossing over each other. The closed arm is surrounded by a high wall of 40 cm. The open and closed arms are joined with 10 cm2 Central Square. On the 28th day, acquisition of memory was measured by placing the animal (facing the centre square) at the terminal part of one open arm. Initial transfer latency (ITL) was measured as the time required for rats to enter one of the closed arms. If the animal fails to enter in closed arms within 120 s, the animal was assisted to enter into one closed arm, allowing them to expose closed arm for 10 s and the ITL was assigned as 120 s. The retention transfer latency (RTL) was measured on day 29 using the same approach as the ITL [Citation21–23].
2.4.4. Modified-forced-swim-test
When rodents were exposed to an inescapable situation, such as a forced swim test, they developed immobile posture, similar to depression in humans. On day 28, the training session was conducted, and rats were allowed to swim in a water-filled tank (25 cm wide × 40 cm long × 16 cm high) for 15 min. On day 29, i.e. 24 h post-training session, animals were again exposed to swimming for 5 min to determine immobility time (the time required to achieve a lack of movement of an entire body with only mobility required to remain the head on the surface of the water). During the test, to avoid the impact of odour, water from the tank was replaced after every animal [Citation21].
3. Biochemical estimations
3.1. Brain tissue homogenate preparation
The animals were decapitated, and the brain was separated and homogenized in phosphate buffer (pH 7.4, 0.1 M, ice-cold). For 15 min, the tissue suspension was centrifuged at 10,000 × g and biochemical analyses were carried out in the supernatant [Citation24,Citation25].
3.2. Total protein
The total protein content of the brain homogenate was determined as described by Lowry et al. [Citation27] using bovine serum albumin as standard [Citation26,Citation27].
3.3. AChE activity
The activity of AChE was estimated as per Ellman et al. [Citation28]. The absorbance changes were measured at 412 nm immediately. Data were calculated as µ mole/mg-tissue [Citation28].
3.4. PON-1 activity
PON-1 was evaluated for its aryl esterase activity by utilizing phenyl-acetate as a substrate. The phenol generation was spectrophotometrically measured by determining the absorption changes at 270 nm and 25°C. The enzyme activity was calculated in Kilo IU/L (kU/L) [Citation29].
3.5. BChE activity
Commercially available kits were used to determine BChE activity. The formation of butyrate and thiocholine by hydrolysis of butyrylthiocholine by cholinesterase is the basis for colourimetric assay. The absorbance changes at 405 nm in unit time are proportional to the cholinesterase activity in the test [Citation30].
3.6. Endogenous antioxidant levels
GSH levels: GSH content in the brain tissue homogenate were estimated as per Ellman [Citation31] method. The GSH content was represented as mg/g tissue at 412 nm [Citation31].
Catalase: 0.1 ml of tissue supernatant and 1.9 ml of phosphate-buffer-solution (pH 7, 50-mM) were added to the cuvette. 1.0 ml of freshly prepared H2O2 (30 mM) was added to the cuvette to initiate the reaction. The H2O2 breakdown rate was determined at 240 nm spectrophotometrically. The catalase activity result was reported as µ moles ofH2O2 used/min/mg protein [Citation32].
SOD levels: 0.2-ml homogenate supernatant of the brain and 0.8 ml of the buffer of glycine (50 mM, pH 10.4) were mixed together. 0.02 ml of epinephrine was added for initiation of the reaction and kept aside for 5 min. Then at 480 nm, the absorbance was recorded. SOD activity was represented as units/mg protein [Citation33].
3.7. Oxidative and nitrative stress markers
MDA levels: MDA was quantified as per the Wills method (1966). The MDA content was measured at 532 nm after its reaction with TBA. The MDA was expressed as ng/g tissue [Citation34].
Nitrite levels: Griess reagent was used to estimate nitrite. At 546 nm wavelength, the absorbance was recorded. The amount of nitrite was measured using a standard-curve of sodium-nitrite and represented in mg/g tissue [Citation35,Citation36].
3.8. Proinflammatory markers
The IL-6, IL-1βand TNF-α were quantified by immunological assay kit (Modern Lab, India) using a microplate reader (Meril Life Science, India) and expressed in pg/ml.
3.9. Statistical analysis
Findings were analyzed statistically by software Graph pad prism-5. Values are expressed in mean ± S.E.M. (n = 6). One-way ANOVA was followed by Tukey’s post hoc test. P-value <.05 is considered as statistically significant.
4. Results
4.1. Behavioural parameters
4.1.1. Catalepsy
In the bar test, normal control animals corrected their posture from the elevated bar quickly. Animals administered with rotenone increased latency to take away paws with P < .05 significance from the elevated surface when compared to normal animals. Butin treatment (25 and 50 mg/kg) improved muscular rigidity in the animals and significantly reduced time with significant value P < .01 and P < .001 to remove the paw from the elevated surface. The result of the catalepsy test is represented in .
4.1.2. Akinesia
Initiation of the moment was found normal in normal control animals as they moved all four limbson a hardwood raised platform. Animals treated with rotenone took significantly (P < .05) more time to remove all four paws indicating the difficulty in movement initiation in animals. Administration of both the tested doses of butin to rotenone treated animals improved the rotenone-made difficulties in the initiation of movement in the rats. The values were statistically significant (P < .01; .001) as compared to rotenone-control animals. The result of the effect of butin on rotenone-induced akinesia is shown in Figure .
4.1.3. Elevated-plus-maze-test
Rotenone decreased memory retention significantly (p < .05) in the animals. Butin (25 and 50 mg/kg) treatment, restored the memory in the animals. The significance value of 25 mg/kg dose was P < .01 while P < .001 was at 50 mg/kg dose when correlated to rotenone control animals. , represents the results of the learning abilities and memory of animals estimated using the EPM test.
4.1.4. Modified-forced-swim-test
The normal control animals spent less time in an immobile state. Administration of rotenone-induced depression in the animals and spending significantly (p < .05) more time in an immobile state in FST. Administration of both doses of butin to rotenone treated rats improved the depression and they spent less (P < .05; P < .001) period in an immobile state as compared to rotenone-control animals. The dose dependent manner of butin with a high dose decreases the FST immobility time than average rotenone. The result of FST is shown in .
5. Biochemical estimations
5.1. Ache activity
The significantly (P < .05) elevated levels of AchE were in rotenone control rats as compared to normal control. Butin treatment attenuated levels of AchE by 15.81% (P < .01) by 25 mg/kg and 31.87% (P < .001) by 50 mg/kg, when correlated to the rotenone control group. The result of AchE is represented in .
5.2. BChE activity
The level BChE, nonspecific cholinesterase activity was significantly increased in the rotenone control group. Administration of butin to rotenone treated rats restored BChE activity towards normal when correlated with rotenone induced rats (P < .05 and P < .01). The result of BChE is shown in .
5.3. PON-1 activity
The hydrolytic enzyme PON-1 was considerably (P < .05) less in rotenone administered animals compared to normal control. Treatment with butin restored the PON-1 activity towards normal compared with rotenone treated control animals. The results of PON-1 activity are depicted in .
5.4. Endogenous antioxidant
Administration of rotenone disturbed endogenous antioxidant levels-GSH, catalase, and SOD. A significantly (P < .05) less catalase, GSH, and SOD levels were seen in rotenone induced animals when correlated with a normal control group. Treatment with a lower and higher dose of butin restored the levels of catalase, SOD (P < .01), and GSH (P < .05) towards normal when correlated with rotenone control animals. Results of GSH, catalase, and SOD levels are shown in Figures .
Figure 8. Effect of butin on GSH levels in rotenone treated rats. Values are expressed in mean ± S.E.M. (n = 6). #P < .05 when compared to normal control rats and *P < .01 and **P < .001 when compared to rotenone control rats. One-way ANOVA followed by Tukey’s post hoc test.
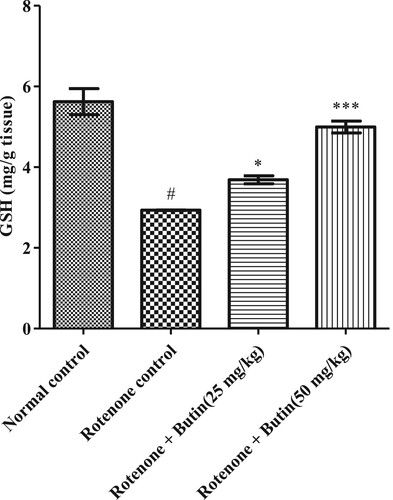
5.5. Oxidative and nitrative stress markers
Administration of rotenone-induced oxidative and nitrative stress markers in the animal’s brain. Significantly (P < .05) high amounts of MDA and nitrite were observed in rotenone treated animals. Treatment with butin at lower and higher doses attenuated the increased levels of MDA (P < .01) and nitrite (P < .001) towards normal when correlated to rotenone control animals. The results of oxidative and nitrative stress are shown in Figures and .
5.6. Proinflammatory markers
The inflammatory markers IL-6, TNF-α, and IL-1β were considerably (P < .05) high in rotenone induced animals when correlated to normal control animals. Butin at the lower and higher dose to rotenone treated animals attenuated the level of IL-6, TNF-α, and IL-1β significantly (P < .01). The IL-6, TNF-α, and IL-1β results are represented in Figures , respectively.
Figure 13. Effect of butin on TNF-α levels in rotenone treated rats. Values are expressed in mean ± S.E.M. (n = 6). #P < .05 when compared to normal control rats and *P < .01 and **P < .001 when compared to rotenone control rats. One-way ANOVA followed by Tukey’s post hoc test.
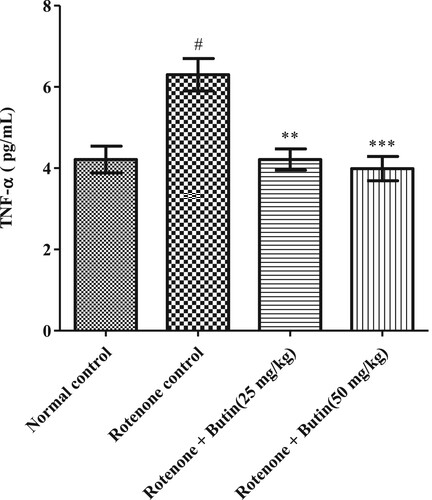
6. Discussion
In the present study, administration butin treated rats attenuated the rotenone-induced catalepsy, akinesia, memory loss, and decreased ability to learn in the rats. In addition to the behavioural alteration, treatment butin also corrected the disturbing levels of AchE, PON-1, and BChE in rotenone treated rat brains. Moreover, treatment with butin reversed the rotenone-induced depleted GSH, catalase, and SOD as well as increased oxidative stress, nitrite, and IL-6, IL-1β as well as TNF-α, levels towards normal when correlated to rotenone control rats.
Rotenone, a toxin present in the roots of several plants and that is poisonous to insects, animals, and humans [Citation37]. Rotenone inhibits NADH oxidation by suppressing the mitochondrial respiratory chain complex-I enzyme and induces neurotoxicity [Citation37]. The dopaminergic neurons are highly susceptible to oxidative insults in the substantia nigra, hence exposure to the rotenone affects these neurons selectively and induces PD like symptoms [Citation37].
In PD, neuronal degeneration begins in the movement-controlling region of the brain, which causes early symptoms such as muscle rigidity, a shuffling step, difficulty initiating movement, and so on [Citation2,Citation38]. The study data well supports the above findings, administration of rotenone-induced muscular rigidity, loss of muscle control, and reduced body movement and it was evident by significant catatonia and akinesia in rotenone treated animals. Treatment with butin to rotenone treated animals decreased the catatonic behaviour and increased the moment of the animals. These results indicated the beneficial actions of butininrotenone-treated muscular rigidity and ultimately reduced body movement in the animals.
As PD progresses, difficulties with cognitive function, such as memory loss, focus problems, and learning abilities also emerge [Citation2,Citation39]. Rotenone-decreased memory and learning abilities in the animals and were evident by the EPM test. Administration of butin to rotenone treated animals improved the memory and learning ability of the animals. This indicates the protective action of butin against rotenone-induced loss of memory and decreased learning abilities in the animals.
Depression is common in people with PD and may begin years before any other symptoms appear [Citation40]. Depressive disorders not only result in inherent emotional distress, but they also negatively affect quality of life, motor and cognitive dysfunction, functional disability, and other psychiatric comorbidities among people with PD [Citation40]. In the present study, rats treated with rotenone spent more time in the immobile posture when they were exposed to force swim test. The immobile posture of rats, when exposed to an inescapable situation, is similar to depression in humans [Citation21]. Administration with butin to rotenone treated rats decreased the time spent in immobile posture when forced to swim in the water. This indicates the anti-depressive effect of butin against rotenone-induced depression in rats.
According to laboratory studies, rats exposed to neurotoxins have increased AChE, the key enzyme involved in acetylcholine hydrolysis. Reduced activity of acetylcholine is responsible for cognitive deficits [Citation41]. Treatment with butin ameliorated the rotenone-induced increased AChE and BChE activity, which is primarily responsible for cognitive deficits. This observation well supports the outcome of EPM test which is evaluated the cognitive performance of the animals such as memory and learning ability.
The mechanism underlying rotenone neurotoxicity is dependent on oxidative stress and ROS production [Citation37]. Administration of rotenone to the rat decreased the endogenous antioxidant levels and increased the oxidative and nitrative stress in the animal’s brain. Treatment with butin to rotenone treated rats attenuated the rotenone-induced depletion of endogenous antioxidant levels and oxidative and nitrative stress in the animals indicating the antioxidant property of butin against rotenone.
The etiology of PD is multifactorial and inflammation may play role in neurodegeneration [Citation42]. Researchers have found that patients with Parkinson’s disease display higher concentrations of inflammatory markers, IL-6, TNF-α, and IL-1β [Citation42]. The findings of the study well support the above findings, in rotenone-induced levels of inflammatory markers. Administration of butin attenuated rotenone-induced proinflammatory markers. This indicates the anti-inflammatory properties of butin against rotenone-induced neuroinflammation in rats. Administration of butin attenuated the rotenone-induced TNF-α, IL-1β, and IL-6. This study shows that butin reduces neuroinflammation induced by rotenone in rats.
PON1 is a hydrolytic enzyme with a broad substrate range and can protect from lipid oxidation and has antioxidant as well as anti-inflammatory actions [Citation43,Citation44]. Rotenone depleted PON1 activity, which may be a factor to increase the oxidative stress and inflammatory response associated with neuronal degeneration in the animals. Butin treatment restored the rotenone-induced depletion of PON1 enzyme activity. These results evidenced the role of PON1 in protecting the animals against rotenone-induced PD like symptoms. The current study results strongly validate the protective effect of butin in PD. Findings of the current study results strongly validate that butin (25 and 50 mg/kg), attenuated the level of oxidative stress, nitrative stress, and neuroinflammatory markers, and reversed the enzyme activities towards normal rats treated with rotenone.
7. Conclusion
The present study demonstrates that butin mitigates behavioural and biochemical abnormalities caused by rotenone in rats. Butin attenuates inflammatory response and free radical damage in rotenone treated rats. The actions of the PON-1 enzyme may be responsible for the observed beneficial effect of butin as an anti-inflammatory and antioxidant activity in PD. Future research is warranted to evaluate butin in the management of Parkinson’s disease in humans.
Disclosure statement
No potential conflict of interest was reported by the author(s).
References
- Alyamani AM, Alarifi J, Alfadhel A, et al. Public knowledge and awareness about Parkinson’s disease in Saudi Arabia. J Family Med Prim Care. 2018;7(6):1216.
- Geibl FF, Henrich MT, Oertel WH. Mesencephalic and extramesencephalic dopaminergic systems in Parkinson’s disease. J Neural Transm. 2019;126(4):377–396.
- Mbiydzenyuy NE, Ninsiima HI, Valladares MB, et al. Zinc and linoleic acid pre-treatment attenuates biochemical and histological changes in the midbrain of rats with rotenone-induced Parkinsonism. BMC Neurosci. 2018;19(1):1–11.
- Hallett M. Tremor: pathophysiology. Parkinsonism Relat Disord. 2014;20:S118–SS22.
- Elbaz A, Tranchant C. Epidemiologic studies of environmental exposures in Parkinson’s disease. J Neurol Sci. 2007;262(1–2):37–44.
- Henchcliffe C, Beal MF. Mitochondrial biology and oxidative stress in Parkinson disease pathogenesis. Nature Clinical Practice Neurology. 2008;4(11):600–609.
- Hwang O. Role of oxidative stress in Parkinson’s disease. Exp Neurobiol. 2013;22(1):11.
- Garabadu D, Agrawal N. Naringin exhibits neuroprotection against rotenone-induced neurotoxicity in experimental rodents. NeuroMol Med. 202022(2):314–330.
- Fischer R, Maier O. Interrelation of oxidative stress and inflammation in neurodegenerative disease: role of TNF. Oxid Med Cell Longevity. 2015;2015:1–18.
- Hassanzadeh K, Rahimmi A. Oxidative stress and neuroinflammation in the story of Parkinson’s disease: could targeting these pathways write a good ending? J Cell Physiol. 2019;234(1):23–32.
- Tian G, Zhang U, Zhang T, et al. Separation of flavonoids from the seeds of Vernonia anthelmintica Willd by high-speed counter-current chromatography. J Chromatogr, A. 2004;1049(1–2):219–222.
- Liu R-X, Wang Q, Guo H-Z, et al. Simultaneous determination of 10 major flavonoids in Dalbergia odorifera by high performance liquid chromatography. J Pharm Biomed Anal. 2005;39(3-4):469–476.
- Su E-N, Yu S-S, Pei Y-H. Studies on chemical constituents from stems and leaves of Adenanthera pavanina. Zhongguo Zhong yao za zhi = Zhongguo zhongyao zazhi = China Journal of Chinese materia medica. 2007;32(20):2135–2138.
- Zhang R, Chae S, Kang KA, et al. Protective effect of butin against hydrogen peroxide-induced apoptosis by scavenging reactive oxygen species and activating antioxidant enzymes. Mol Cell Biochem. 2008;318(1):33–42.
- Kang KA, Lee JH, Chae S, et al. Butin decreases oxidative stress-induced 8-hydroxy-2′-deoxyguanosine levels via activation of oxoguanine glycosylase 1. Chem-Biol Interact. 2009;181(3):338–342.
- Zhang R, Kang KA, Piao MJ, et al. Butin reduces oxidative stress-induced mitochondrial dysfunction via scavenging of reactive oxygen species. Food Chem Toxicol. 2010;48(3):922–927.
- Zhang R, Lee IK, Piao MJ, et al. Butin (7,3′,4′-trihydroxydihydroflavone) reduces oxidative stress-induced cell death via inhibition of the mitochondria-dependent apoptotic pathway. Int J Mol Sci. 2011;12(6):3871–3887.
- Alshehri S, Al-Abbasi FA, Ghoneim MM, et al. Anti-Huntington’s effect of butin in 3-nitropropionic acid-treated rats: possible mechanism of action. Neurotox Res. 2022;40(1):66–77.
- Alzarea SI, Alasmari AF, Alanazi AS, et al. Butin attenuates arthritis in complete Freund’s adjuvant-treated arthritic rats: possibly mediated by its antioxidant and anti-inflammatory actions. Front Pharmacol. 2022;13:810052.
- Sharma N, Bafna P. Effect of Cynodon dactylon on rotenone induced Parkinson’s disease. Orient Pharm Exp Med. 2012;12(3):167–175.
- Venkateshgobi V, Rajasankar S, Johnson W, et al. Neuroprotective effect of Agaricus blazei extract against rotenone-induced motor and nonmotor symptoms in experimental model of Parkinson’s disease. Int J Nutr, Pharmacol, Neurol Dis. 2018;8(2):59–65.
- Kumar P, Kumar A. Protective effect of rivastigmine against 3-nitropropionic acid-induced Huntington’s disease like symptoms: possible behavioural, biochemical and cellular alterations. Eur J Pharmacol. 2009;615(1–3):91–101.
- Dhadde SB, Nagakannan P, Roopesh M, et al. Effect of embelin against 3-nitropropionic acid-induced Huntington’s disease in rats. Biomed Pharmacother. 2016;77:52–58.
- Shaikh A, Dhadde SB, Durg S, et al. Effect of Embelin against lipopolysaccharide-induced sickness behaviour in mice. Phytother Res. 2016;30(5):815–822.
- Durg S BNK, Vandal R, Dhadde SB, et al. Antipsychotic activity of embelin isolated from Embelia ribes: a preliminary study. Biomed Pharmacother. 2017;90:328–331.
- Dhadde SB, Durg S, Potadar PP, et al. Piroxicam attenuates 3-nitropropionic acid-induced brain oxidative stress and behavioral alteration in mice. Toxicol Mech Methods. 2014;24(9):672–678.
- Lowry OH, Rosebrough NJ, Farr AL, et al. Protein measurement with the Folin phenol reagent. J Biol Chem. 1951;193(1):265–275.
- Ellman GL, Courtney KD, Andres V, et al. A new and rapid colorimetric determination of acetylcholinesterase activity. Biochem Pharmacol. 1961;7(2):88–95.
- Wysocka A, Cybulski M, Berbeć H, et al. Dynamic changes of paraoxonase 1 activity towards paroxon and phenyl acetate during coronary artery surgery. BMC Cardiovasc Disord. 2017;17(1):92-.
- Abdel-Salam OME, Youness ER, Khadrawy YA, et al. Acetylcholinesterase, butyrylcholinesterase and paraoxonase 1 activities in rats treated with cannabis, tramadol or both. Asian Pac J Trop Med. 2016;9(11):1089–1094.
- Ellman GL. Tissue sulfhydryl groups. Arch Biochem Biophys. 1959;82(1):70–77.
- Aebi H, Wyss SR, Scherz B, et al. Heterogeneity of erythrocyte catalase II. Isolation and characterization of normal and variant erythrocyte catalase and their subunits. Eur J Biochem. 1974;48(1):137–145.
- Misra HP, Fridovich I. The role of superoxide anion in the autoxidation of epinephrine and a simple assay for superoxide dismutase. J Biol Chem. 1972;247(10):3170–3175.
- Wills ED. Mechanisms of lipid peroxide formation in animal tissues. Biochem J. 1966;99(3):667–676.
- Green LC, Wagner DA, Glogowski J, et al. Analysis of nitrate, nitrite, and [15N]nitrate in biological fluids. Anal Biochem. 1982;126(1):131–138.
- Nagakannan P, Shivasharan BD, Thippeswamy BS, et al. Restoration of brain antioxidant status by hydroalcoholic extract of Mimusops elengi flowers in rats treated with monosodium glutamate. J Environ Pathol Toxicol Oncol. 2012;31(3):213–221.
- Lawana V, Cannon JR. Chapter five – rotenone neurotoxicity: relevance to Parkinson’s disease. In: Aschner M, Costa LG, editor. Advances in neurotoxicology. 4. Cambridge (MA): Academic Press; 2020. p. 209–254.
- Klein MO, Battagello DS, Cardoso AR, et al. Dopamine: functions, signaling, and association with neurological diseases. Cell Mol Neurobiol. 2019;39(1):31–59.
- Liu C, Kaeser PS. Mechanisms and regulation of dopamine release. Curr Opin Neurobiol. 2019;57:46–53.
- Marsh L. Depression and Parkinson's disease: current knowledge. Curr Neurol Neurosci Rep. 2013;13(12):409-.
- Binawade Y, Jagtap A. Neuroprotective effect of lutein against 3-nitropropionic acid-induced Huntington’s disease-like symptoms: possible behavioral, biochemical, and cellular alterations. J Med Food. 2013;16(10):934–943.
- Caggiu E, Arru G, Hosseini S, et al. Inflammation, infectious triggers, and Parkinson’s disease. Front Neurol. 2019;10:122.
- Litvinov D, Mahini H, Garelnabi M. Antioxidant and anti-inflammatory role of paraoxonase 1: implication in arteriosclerosis diseases. N Am J Med Sci. 2012;4(11):523–532.
- Shunmoogam N, Naidoo P, Chilton R. Paraoxonase (PON)-1: a brief overview on genetics, structure, polymorphisms and clinical relevance. Vasc Health Risk Manag. 2018;14:137–143.