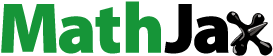
ABSTRACT
The present study was designed to explore the biological potential of aerial parts of Berberis baluchistanica. HPLC analysis revealed the highest berberine content in leaves (19453.15 ppm) followed by roots (3225.12ppm) and stem (29.11ppm). Phenolics, flavonoids, tannins, DPPH inhibition activity and total antioxidant capacity are reported in root, leaf and stem extracts. Antihaemolytic assay displayed the highest (97%) inhibition potential of extracts against H2O2-induced haemolysis than heat-induced haemolysis (75%). The highest DNA protection activity was observed in root and leaves extracts while the antimicrobial potential was recorded highest in the leaves extract against Staphylococcus aureus (23.00 ± 1.0 mm) and Klebsiella pneumonia (19.67 ± 0.2 mm). Similarly, brine shrimp cytotoxicity potential was observed highest in root extract (LC50 – 10.04 ppm) and the allelopathic potential was confirmed by radish seed phytotoxicity assay. Overall, results suggest a remarkable nutritional and biological potential B. baluchistanica, especially areal parts can help to preserve the endemic plants by discouraging the use of roots.
1. Introduction
Since the Neolithic era, medicinal plants have been used to cure diseases ranging from the common cold to cancer [Citation1]. Even today, approximately 80% of the world’s population uses herbal medicines as a source of primary healthcare. These estimates are higher in developing and underdeveloped countries of the world [Citation2]. The demand for herbal drugs is increasing continuously, as these are safer and more economical than clinical drug therapy which has side effects on human health and the environment [Citation3]. Natural plant-based products possess biological active compounds like flavonoids which contribute to the therapeutic properties [Citation4].
The genus Berberis is the largest genus of the family Berberidaceae, with ∼17 genera and 650 species, including shrubs that are widely distributed in Asia, Europe and America [Citation5]. In Pakistan, 29 Berberis species have been reported in the mountainous ranges of the country [Citation6]. Baluchistan is the largest province of Pakistan with versatile ecological conditions. Several diverse flora and fauna are restricted to this province due to its spatial topography and climate [Citation7]. Local communities use medicinal plants against numerous diseases viz. typhoid, oedema, kidney pain, purification of blood, joints pain, hair fall, chest infections, jaundice, pimples, toothache, high blood pressure, diabetes and snake bites [Citation8].
Berberis baluchistanica is an endemic species of Baluchistan province, distributed mainly in Kalat, Hanna Urak and their allied areas, locally known as Zralga in Pashto and Zarchin in Brahui [Citation9]. Its root extracts are a rich source of secondary metabolites and are used as beneficial herbal agents to cure cough, microbial infection and internal injuries of human beings and livestock [Citation10]. Many antioxidant compounds present in this endemic plant are very useful in the reduction of cancer mortality and coronary heart diseases [Citation8]. Similarly, roots of B. baluchistanica have also been explored for various other purposes such as gentamicin-induced renal toxicity [Citation11], food preservatives [Citation12], and antimicrobial and insecticidal activity [Citation9].
Due to their multiple biological properties, such plants are misused for clinical and nutritional purposes and thus face survival challenges, resulting in the diminution of natural reservoirs [Citation13]. According to the International Union for Conservation of Nature and the World Wildlife Fund, more than 15,000 plant species are going to become extinct due to over-exploitation [Citation14]. These issues can be addressed by adopting strategies like identification and development of conservation areas, discouraging of uprooting of medicinal plants and encouraging the usage of substitutional approaches by utilizing the renewable and alternative aerial parts of the plants for medical purposes [Citation15].
Keeping in mind the excessive traditional use of B. baluchistanica roots in treating various disorders, the present study was designed to ascertain the biological potential of stem and leaves along with the roots so that the aerial parts could also be utilized as an alternative approach by substituting the roots. This work also deciphers the nutritional and phytochemical composition of the selected extracts by performing various spectroscopy and chromatography techniques.
2. Materials and methods
2.1. Collection of plant material
The plant material was collected from the mountainous regions of Hanna Urak, Quetta, Baluchistan (Pakistan) and then the sample was identified by comparing it with the already present herbarium specimens and flora of Pakistan. A voucher specimen (RAW100268) was deposited at the National Herbarium, Islamabad, Pakistan.
2.2. Preparation of plant extracts
Roots, stems and leaves of B. baluchistanica were washed thoroughly under running tap water and placed at room temperature for one week followed by drying in an oven for 24 h at 40°C. About 20 g of each powdered plant material was added into 200 mL of methanol and placed on a stirrer for 12–18 h. Afterwards, samples were filtered using Whatman filter paper to remove all types of course materials and the whole process was repeated three times. Finally, the obtained extracts were evaporated using a rotary evaporator (BUCHI Rotavapor R-220).
2.3. Elemental analysis
A total of six elements, namely, sodium (Na), calcium (Ca), magnesium (Mg), potassium (K), zinc (Zn) and iron (Fe), were estimated in selected species using atomic absorption spectrophotometry (Spectra AA240 FS, Varian, NJ, USA). For the sample digestion, 0.5 g of each dried root, stem and leaves sample was added in an 8 mL digestion mixture of HNO3:HClO4 (3:1 v/v) and left overnight. Then, the samples were placed on a hot plate and heated until brown fumes turned white. After some time, the digested mixtures were diluted with 25 mL distilled water followed by filtration using Whatman filter paper. The collected filtrates were analysed using a spectrophotometer which was operated at respective wavelengths ranging from 213 nm to 283 nm. The atomization temperature (°C) was set at 1400 and 2300 using an HCl lamp and the detection limits ranged from 0.1 to 5.0 ppm [Citation16].
2.4. UV-Vis and FTIR analysis
For UV-Vis spectroscopic analysis, the plant samples were centrifuged at 3000 rpm for 10 min and then filtered and diluted (1:10) using deionized water. Subsequently, every individual sample was scanned by UV-Vis spectrophotometer (Emclab, EMC-11-UV, Germany) at 200–800 nm. For FTIR analysis, the samples were prepared by encapsulating 10 mg of plant powder in 100 mg of dried potassium bromide (KBr) pellet and were subjected to a pressure of about 5 × 106 Pa in an evacuated dye. Subsequently, Perkin Elmer-Spectrum 65 (FTIR model) was used to record the spectra at 5000–500 cm−1 frequency [Citation17].
2.5. HPLC analysis of berberine
For HPLC analysis, the plant extracts (10 µL) were added in 5 mL of 10% methanol and then filtered using 0.45 nm membrane filters. An Agilent 1260 HPLC series (Agilent Technologies, USA) equipped with a quaternary pump and Zorbax Eclipse XDB-C18 column (100 mm × 4.6 mm) was operated at 30°C. Methanol, 0.2% H3PO4 and acetonitrile were used as mobile phase with a flow rate of 1 mL/min. The injected volume was 10 µL and the wavelength was set at 340 nm to identify berberine content. In the end, quantification was done by using the peak area of the standard [Citation18].
2.6. Total phenolic, flavonoid and tannin content
For the phenolic contents, the Folin–Ciocalteu (FC) method of Sumczynski et al. [Citation19] was followed with slight modifications. The calibration curve was made by mixing gallic acid with 90 µL of FC reagent and 90 µL of NaCO3 solution. Plant extracts (20 µL) were mixed with the same reagent and then the mixtures were incubated at room temperature for 60 min and the absorbance was measured. Total phenolic contents were expressed as gallic acid equivalents (mg GAE/g sample). However, flavonoid contents were measured using the aluminium chloride colourimetric method [Citation20]. Briefly, 20 µL of each extract was added in 10 µL of potassium acetate, 10 µL of aluminium chloride and 160 µL of distilled water. The absorbance was measured at 510 nm and results were expressed as mg of quercetin equivalent per gram.
Total tannin content (TTC) was determined by Folin–Denis method as described by Ram and Mehrotra [Citation21]. Briefly, 0.5 mL of Folin–Denis reagent was added to 5 mL of extract. 1 mL of sodium carbonate (7%) and 3.5 mL of distilled water were added to the mixture and placed at room temperature for 20 min. The absorbance was measured at 700 nm and the standard curve of tannic acid was prepared in 80% methanol. Total tannin content was determined as milligrams of tannic acid equivalent per 100 g of dry weight (mg TAE/100 g DW).
2.7. Antioxidant assays
For the DPPH assay, the reagent solution was prepared by adding 2.4 mg of DPPH into 25 mL of methanol. Then 20 µL of the test sample was added into 180 µL of reagent solution followed by the incubation in dark for 30 min. The absorbance was measured at 517 nm using a microplate reader and scavenging activity was calculated using the following formula [Citation22]:
Total antioxidant capacity was estimated by following the phosphomolybdenum method [Citation23]. The tubes containing the extract and reagent solution (0.6 mol L−1 sulphuric acid, 28 mmol L−1 sodium phosphate and 4 mmol L−1 ammonium molybdate) were incubated at 95°C for 90 min and then cooled at room temperature. The absorbance was measured at 695 nm and the ascorbic acid was measured as a standard.
2.8. Antihaemolytic activity
Six millilitres of human blood were collected in EDTA vials from healthy humans and the study approval (#BEC-FBS-QAU2019-143) for the use of blood was taken from the Bioethical Committee of Quaid-i-Azam University, Islamabad (Pakistan). For the H2O2-induced haemolytic assay, the human blood was diluted with PBS and then centrifuged at 1000 rpm for 12 min. Subsequently, the pellet was collected and washed three times with 0.2 M phosphate buffer and then diluted (4%) with phosphate buffer (pH 7.4). 1 mL of diluted erythrocyte suspension along with 0.5 mL PBS and 1 mL of each extract (1000, 500 and 250 µg/mL) were incubated and then centrifuged for 10 min to detect the absorbance [Citation24].
In heat-induced haemolysis, the red blood cells (3 mL) were taken as a 40% suspension in 15 M sodium phosphate buffer and then incubated for 20 min at 55°C followed by the centrifugation (Model 2-6E, Sigma Laborzentrifugen, D-37520 Osterode am Harz, Germany) at 5000 rpm for 5 min at 4°C. The absorbance of the supernatant was estimated at 540 nm and percentage inhibition was calculated using the following formula [Citation25]:
2.9. DNA damage protection assay
For DNA damaging assay, p1391Z plasmid and wheat genomic DNA were used to study the protection capacity of tested plant extracts against oxidative damage caused by H2O2. Briefly, 1 µL of plasmid DNA and 3 µL of wheat genomic DNA were incubated with 1 µL of 1 mM FeSO4, 1 µL of 10% H2O2 and 3 µL of each (5, 2.5, 1 and 0.5 mg mL−1) plant extract. The final volume of the reaction mixture was made up to 20 µL with phosphate buffer (pH 7.0). DNA were exposed to the Fenton’s reagent alone and with different concentrations of plant extracts. After 1-hour incubation (at 37°C), the samples were loaded on 1% agarose gel containing 3 µL ethidium bromide and were allowed to run in the gel electrophoresis apparatus. The gel-containing DNA bands were photographed under UV light by using a gel documentation system [Citation26].
2.10. Antimicrobial assay
Four bacterial strains namely Staphylococcus aureus (ATCC 48755), Pseudomonas aeruginosa (ATCC 23451), Klebsiella pneumonia (ATCC 78501) and Escherichia coli (ATCC 30155) and three fungal strains including Alternaria alternata, Aspergillus niger (FCBP 0198) and Fusarium oxysporum were collected from the Department of Microbiology, Quaid-i-Azam University, Islamabad. The activity was assessed using the well diffusion method using autoclaved Müller-Hinton agar and Sabouraud dextrose agar media (Oxoid – CMO147) were used with different concentrations of plant extracts and kanamycin and fluconazole drugs were taken as standards. After incubation, the zones of inhibition were recorded with the help of a ruler by measuring the diameter of the clear zone around each well [Citation27].
2.11. Brine shrimp’s cytotoxicity assay
To confirm the biocompatible nature of B. baluchistanica plant extracts, the cytotoxic assay was performed using Artemia salina larvae (Ocean Star, UT, USA). Eggs of A. salina were incubated for 48 h at 30°C in seawater (3.8% sea salt in 1000 mL of distilled water). Six concentrations (10, 100, 500, 1000, 2500 and 5000 µg mL−1) of plant extracts were added in different vials and their final volume was brought to 5 mL using saline solution. After 24 h, 10 shrimps were transferred to each vial and were incubated at 32°C for the next 24 h and then the number of alive shrimps were counted. In the end, percentage mortality and LC50 values were calculated [Citation28].
2.12. Radish seed phytotoxicity assay
For phytotoxic assessment, three concentrations (100, 250 and 500 µg mL−1) of plant extracts were added to each Petri plate inside a laminar hood (Model SC2-41 Changi South, Singapore) and sterilized Whatman filter papers were placed in Petri plates. After the evaporation of all solutions, distilled water (5 mL) was added. 15 sterilized seeds were placed in each Petri plate and then incubated at 25°C in dim light. On the fifth day, seed germination indices were calculated along with root length by using the following formula [Citation29]:
2.13. Statistical analysis
All experiments were performed three times (n = 3) and then mean values and standard deviation were determined using Microsoft Excel. The obtained data were subjected to ANOVA using Statistix 8.1 software and a comparison among mean values was made by LSD. Graphpad Prism was used to determine IC50 values and a probit analysis programme was used to calculate LC50 values and 95% confidence interval [Citation30].
3. Results
3.1. Elemental analysis
The concentration of six elements determined in different parts of B. baluchistanica is presented in Table . Comparatively, root extract showed the highest concentration of Ca (2840 ± 2.75 mg kg−1) followed by Na (345.78 ± 2.98 mg kg−1) and Zn (90.11 ± 1.05 mg kg−1) whereas stem extract showed the highest concentration of K (1037.04 ± 2.48 mg kg−1) than roots and leaves. However, the concentration of Mg (595.10 ±2.76 mg kg−1) and Fe (524.29 ± 1.78 mg kg−1) was detected significantly in higher concentration in B. baluchistanica leaves extract as compared to its stem and root extracts.
Table 1. Concentrations of six elements observed in roots, stem and leaves extracts of B. baluchistanica.
3.2. UV-Vis and FTIR spectroscopy
The UV-VIS profiles of B. baluchistanica roots, stems and leaves were characterized in the range of 200–800 nm as peaks baseline and sharpness. The absorption was recorded in the range of 224.5–274 and 338.5–429 in roots, 210.5–278.5 in stems and 221–294 and 331 in leaves of selected species (Figure (a–c)) These peaks represented the presence of glycosides, phenolic acids, flavones and chlorophyll contents. Additionally, the multiple peaks in the range of 200–400 nm showed the presence of unsaturated heteroatoms.
Figure 1. Spectral analysis of B. baluchistanica extracts recorded at different wavelengths by using UV-Vis and FTIR spectroscopy:(a) UV-Vis spectroscopy of B. baluchistanica root extract. (b) UV-Vis spectroscopy of B. baluchistanica stem extract. (c) UV-Vis spectroscopy of B. baluchistanica leaves extract. (d) FTIR spectrum of B. baluchistanica root extract. (e) FTIR spectrum of B. baluchistanica stem extract and (f) FTIR spectrum of B. baluchistanica leaves extract.
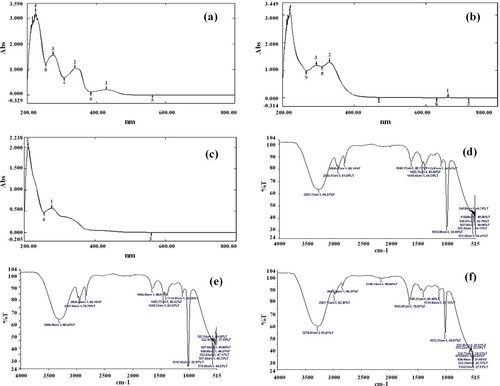
In the FTIR profile, various peaks were detected at 3306.95–3278.91 cm−1 (N–H or OH stretch), 40.56–2834.58 cm−1 (C–H stretch), 1655.05–1396.31 cm−1 (C=C stretch), 1114.57–1013.15 cm−1 (C–O bend) and 587.04–518.67 cm−1 (C–Cl /C–Br stretch) frequencies which indicated the presence of phenols, alcohols, amines, amides, alkane, alkene, carboxylic acid, alkyl halides and aromatic compounds in selected extracts (Figure (d–f)). Another peak was observed at2146.14 cm−1 frequency which indicated the presence of alkynes (C≡C stretch) in leaves only (Figure (f)). However, all other functional groups were found in all parts of B. baluchistanica (Table ).
Table 2. Functional groups observed in the root, stem and leaves of B. baluchistanica using the FTIR technique.
3.3. HPLC analysis
The HPLC analysis was performed to ascertain berberine content in different organs of selected specie and the quantification was done by using the peak area of the standard. Results revealed the highest berberine concentration in the leaves (19,453.15 ppm) and roots (3225.12 ppm) of B. balucistanica species (Table ). However, the stem displayed the lowest berberine content (29.11 ppm) as shown in Figure .
Figure 2. HPLC chromatogram indicating the presence of berberine content in B. baluchistanica. (a) Roots, (b) stem, (c) leaves and (d) standard Berberine.
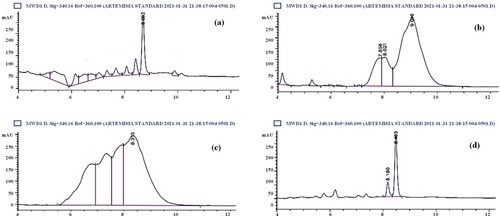
Table 3. The concentration of berberine detected in Berberis baluchistanica species.
3.4. Phytochemical analysis and antioxidant assays
Results revealed that root extracts possess the highest phenolic (136.54 ± 0.07 mg GAE/g) and flavonoid contents (40.62 ± 0.37 mg QE/g) followed by the stem extract (119.77 ± 0.04 mg GAE/g and 30.48 ± 0.35 mg QE/g). However, leaf extract displayed the lowest phenolic (118.44 ± 0.06 mg GAE/g) and flavonoid (37.39 ±0.57 mg QE/g) contents. Tannins were found in descending order of 100.49 ± 1.11 mg TAE/g (root extract) > 98.40 ± 6.11 mg TAE/g (stem extract) > 79.72 ± 8.99 mg TAE/g (leaves extract) (Figure (a)).
Figure 3. Antioxidant assays and their correlation analysis with phytochemicals as determined in roots, stem and leaves extracts of B. baluchistanica (a) Total phenolic, flavonoid and tannin contents (b) DPPH scavenging activity (c) Total antioxidant capacity(d) Pearson correlation analysis between phytochemicals and DPPH assay (e) Pearson correlation analysis between phytochemicals and total antioxidant capacity. Data represent the mean of three replicates (n = 3) and error bars represent standard deviation. Pearson correlation analysis was determined using Microsoft Excel. TPC: Total phenolic content; TFC: Total flavonoid content; TTC: Total tannin content; r: Correlation coefficient.
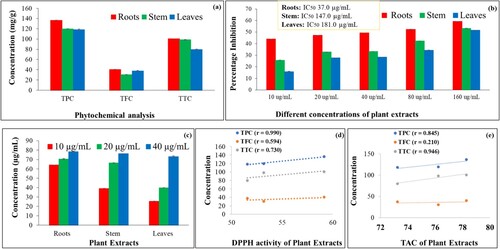
The antioxidant activity was found to be dose-dependent and the highest DPPH activity was observed in root extract which displayed an IC50 value of 37.04 µg mL−1, followed by the stem extract (147.00 µg mL−1) and leaves extract (181.00 µg mL−1) (Figure (b)). Similarly, total antioxidant capacity (TAC) was also observed highest in root extract i.e. 78.284 ± 0.26 µg mL−1 as compared to the stem (76.218 ± 0.17 µg mL−1) and leaves (73.23 ± 0.57 µg mL−1) extracts (Figure (c)). Comparatively, root extract showed the highest antioxidant activity in comparison with the aerial parts of B. baluchistanica. Pearson correlation analysis showed a strongly positive correlation between phytochemicals and antioxidant assays (Figure (d,e)).
3.5. Antihaemolytic assay
In the present study, all parts of B. baluchistanica were examined at three different concentrations i.e. 250, 500 and 1000 µg mL−1 and a comparison between the H2O2- and heat-induced antihaemolytic potential of plant extracts was determined. Results revealed that root, stem and leaves extracts exhibited almost similar antihaemolytic potential and the highest percentage inhibition (97%) was observed at 1000 µg mL−1. Comparatively, selected extracts were highly effective in inhibiting H2O2-induced haemolysis (97%) as compared to the heat-induced haemolysis which showed up to 75% inhibition only (Figure ).
Figure 4. Effect of different concentrations of B. baluchistanica extracts on H2O2- and heat-induced haemolysis as compared with the quercetin (standard). Data indicate the mean of three replicates (n = 3) and the standard deviation is indicated by error bars. Each alphabetical letter (a–c) represents significance at P < 0.05 as determined by LSD.
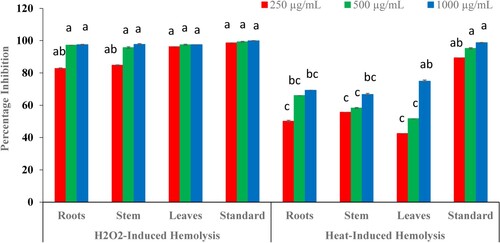
3.6. DNA protection assay
To assess the oxidative DNA damage protection ability of plant extracts, two different plasmids (p1391Z plasmid and wheat genomic DNA) were used. The agarose gel shows that negative control (C−), which was untreated with Fenton’s reagent had bright bands in both types of DNA, while in the positive control (C+), DNA bands were not found on the gel due to DNA degradation by Fenton’s reagent. As the plant extracts were added along with Fenton’s reagent, the DNA protection on the gel appeared with bright bands. DNA treated with different concentrations of B. baluchistanica roots and leaves extracts were highly effective in preventing DNA from oxidative damage; however, DNA treated with stem extract showed protection only at higher concentrations (5.0 mg mL−1) (Figure ).
Figure 5. Effects of different concentrations of B. baluchistanica extracts against oxidative damage to p1391Z plasmid DNA and wheat genomic DNA. Lanes – A: Plasmid DNA; B: Wheat DNA; C−: Negative control (untreated DNA); C+: Positive control (Treated DNA with Fenton reagent); and “C+” show negative control (Untreated DNA) and positive control (Treated DNA with Fenton reagent); R1–R4: 0.5, 1.0, 2.5 and 5.0 mg mL−1 concentration of root extracts with DNA and Fenton reagent; S1–S4: 0.5, 1.0, 2.5 and 5.0 mg mL−1 concentration of stem extracts with plasmid DNA and Fenton reagent; L1–L4: 0.5, 1.0, 2.5 and 5.0 mg mL−1 concentration of leaves extracts with plasmid DNA and Fenton reagent.
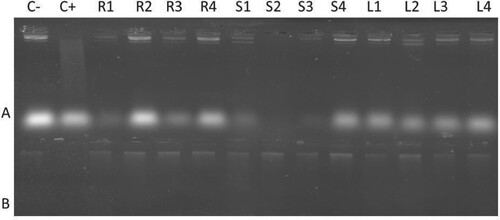
3.7. Antibacterial and antifungal assays
Results of antibacterial activity revealed that root extracts exhibited the highest inhibition potential against all strains while leaves extracts showed the lowest activity against tested bacterial species. However, stem extract only displayed inhibition zones against S. aureus and remain inactive against P. aeruginosa, K. pneumonia and E. coli. Among all extracts, maximum zones were recorded in leaves extract against S. aureus (23.0 ± 1.0 mm) and K. pneumonia (19.6 ± 0.2 mm) while root extract against E. coli (19.6 ± 0.5 mm) as shown in Figure (a).
Figure 6. Inhibition zones showing antimicrobial activity of B. baluchistanica extracts against seven pathogens using Kanamycin and Fluconazole as standard drugs. Data indicate the mean of three replicates and each alphabetical letter (a–d) represents significance at P < 0.05 as determined by LSD: (a) antibacterial activity and (b) antifungal activity.
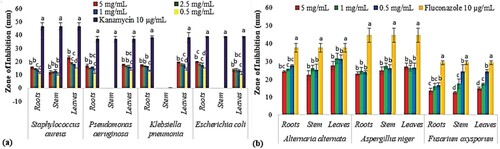
The antifungal activity revealed that all the selected extracts were more effective at the lowest concentration (0.5 and 1.0 mg mL−1) in inhibiting mycelial growth. The highest activity was displayed by the leaves extract against A. alternata i.e. 31.6 ± 2.8 mm inhibition zone (1.0 mg mL−1) followed by the leaves and stem extract against F. oxysporum (24.3 ± 3.7 mm and 24.3 ± 1.1 mm) when examined using 0.5 mg mL−1 concentration. In general, all fungal species were regarded as sensitive strains when tested against B. baluchistanica extracts (Figure (b)).
3.8. Toxicological analysis
Both aerial and underground parts of B. baluchistanica showed significant cytotoxic activity against brine shrimps. Results showed a dose-dependent response and root extract showed the highest cytotoxicity as it displayed an LC50 value of 10.04 ppm followed by the stem extract (10. 86 ppm). However, leaves extract showed the lowest toxicity potential with an 18.32 ppm LC50 value (Table ).
Table 4. Percentage mortality of brine shrimps observed by applying different concentrations of Berberis baluchistanica extracts and their LC50 values.
In the radish seed phytotoxicity assay, the seed germination and inhibition percentages were found to be less sensitive to the different concentrations of all extracts; however, germination speed and T50 (days required for 50% germination) were retarded and delayed after applying different concentrations of plant extracts. Overall, root extracts showed 16.67% toxicity at higher concentrations, while stem and leaf extracts showed only 10.00% inhibitory effects (Table ). Similarly, root extracts also showed the highest root and shoot length inhibition was also observed in root extracts (85.35 ± 0.12% and 41.30 ± 0.21%) as compared to the stem (50.40 ± 2.52% and 28.43 ± 1.51%) and leaves extract (52.02 ± 1.11% and 23.08 ± 0.47%) respectively (Figure ).
Figure 7. Phytotoxic potential of B. baluchistanica roots, stem and leaves extracts. Data show the mean of three replicates and each alphabetical letter (a–e) indicates significance at P < 0.05 as determined by LSD: (a) root length inhibition percentage and (b) shoot length inhibition percentage.
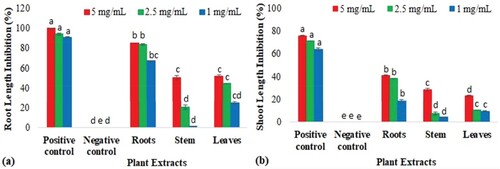
Table 5. Germination indices of radish seeds measured against methanolic extracts of Berberis baluchistanica.
4. Discussion
Medicinal plants are widely used in pharmacological practices providing nutritional supplements and health safety drugs, especially the roots of medicinal plants, which puts them under threat of extinction [Citation31]. The present study describes the nutritional and biological potentials of the aerial parts of B. baluchistanica for the first time and presents a comparison with roots so that they can be used as a substitute for roots for medicinal purposes.
Elements play a vital role in plant metabolism, the structure of biomolecules and participate in various biochemical reactions [Citation32]. However, these elements can be lethal if used beyond their safe daily consumption limits. The recommended dietary allowances of Na, K, Mg and Ca are 3000, 3700, 350 and 1500 mg day−1, respectively [Citation33]. Similarly, the permissible limit of Zn and Fe in edible plants is 27.4 and 20.0 ppm [Citation34]. Apart from B. baluchistanica root extract, our results confirmed that stem and leaves extracts also comprise of significant concentration of elements and thus can be utilized equally as a potent source of elements. Previously, many other Berberis species have been studied for their nutritional composition [Citation35,Citation36]; however, this is the first report on B. baluchistanica showing that the stem and leaves of the plant are also nutrient-rich and safe and hence could be exploited in synthetic foods and drugs.
The secondary metabolites in plants have phenolic hydroxyl groups that express antioxidant activities and play protective roles against various stresses [Citation37]. In the present study, B. baluchistanica roots and leaves showed two absorption bands, while the stem showed one absorption maxima, which indicates that all plant parts had flavonoid compounds. These findings are also further supported by the phytochemical analysis and the antioxidant data. The UV-Vis and FTIR results revealed the existence of N–H, OH, C–H, C=C, C≡C, C–O and C–Cl /C–Br functional groups showing the presence of phenols, alcohols, amines, amides, alkane, alkene, alkyne, carboxylic acid, alkyl halides and aromatic compounds.
The present results are coherent with the earlier studies of B. aristata leaves which demonstrated the presence of various functional groups [Citation38]. These are involved in the structural and natural defence system of plants, like amines and amides are elementary parts of proteins, while phenols, aldehydes, aliphatic compounds and alkyl halides aid in providing defence by acting as fungicides, insecticides and refrigerants [Citation39,Citation40]. Moreover, the OH groups possess the ability to form hydrogen bonds with peptides or proteins and impair the activities of microorganisms and predators [Citation41], again indicating the defensive potential of the selected plant. As berberine is the major alkaloid present in the Berberis genus, hence its concentration was detected in different organs using the HPLC method. Our study is coherent with the earlier findings of Abdykerimova et al. [Citation42] and Ahmad et al. [Citation43] who determined significant berberine content in the leaves of different species of genus Berberis i.e. Berberis iliensis and roots of Berberis vulgaris.
The phenolic compounds contribute directly to the antioxidant activity of plant extracts [Citation44]. Our results revealed that root extracts possess the highest TPC, TFC, TTC and antioxidant potential and the highest activity is directly correlated with the presence of phenolic, flavonoid and tannin contents. Besides this, stem and leaves extracts also displayed significant concentrations of phytochemicals and antioxidant potential. Our study also correlates with the previous studies of Batool et al. [Citation45] who reported 230.2.9 µg mL−1 IC50 value of DPPH scavenging activity in the methanol extracts of B. baluchistanica roots. Present results are also consistent with the study of Bhatt et al. [Citation46] in which different parts of B. aristata and B. thomsoniana showed concentration-dependent antioxidant activity ranging from 19.38% to 98.47%, respectively. It can be concluded that stem and leaves extracts are also medicinally valuable and could be consumed as antioxidants in the food and drug industries.
Oxidative stress and many other factors like heat, oxidative drugs and UV radiations may cause haemolysis of human red blood cells (RBCs) [Citation47]. Moreover, hydroxyl radicals have the ability to react with the nucleotides and whole DNA molecule causing breakage in DNA and ultimately leading to mutagenesis, carcinogenesis and other disorders [Citation48]. Medicinal plants are well known for their abilities to protect genetic material by scavenging reactive oxygen species [Citation47]. In the current study, all the tested plant extracts showed high protection of RBCs against stress induced by H2O2 as compared to heat-induced haemolysis. Similarly, DNA damage protection was observed in descending order of roots extract > leaves extracts > stem extract. The high antihaemolytic and DNA protection capacity of B. baluchistanica extracts could be attributed to the presence of phenolic and flavonoid contents in these extracts.
The increasing multi-drug resistant pathogens have threatened the use of synthetic drugs and put demands on the need for novel antibacterial agents. From this perspective, plant-based antimicrobials are highly effective in curing diseases [Citation49]. The present study revealed that the B. baluchistanica roots and leaves extracts exhibited inhibitory effects against all bacterial strains, but gram-negative strains showed resistance against stem extract. Our study is parallel with the earlier findings of Kakar et al. [Citation9], Batool et al. [Citation45] and Idrees et al. [Citation50] who reported antibacterial potential in the methanol root extract of B. baluchistanica. This activity can be accredited to the TPC and TFC as these are associated with the growth inhibition of microbial pathogens. Hence, the present study supports the idea of using the aerial plant parts for industrial use, which will help in protecting this invaluable plant from becoming endangered by using roots only. Moreover, the present study also revealed that few species were more effective at the lowest concentration and their activity was reduced at higher concentrations. Previously, Parekh and Chanda [Citation51], also reported that methanolic plant extracts exhibit antimicrobial activity against pathogenic yeasts and moulds and it was not concentration dependent. This might be due to the fact that the concentration of some specific compounds that are associated with microbial growth inhibition remains static even at higher concentrations, contrarily concentration of other compounds might get increased. Hence, their antimicrobial potential was decreased at higher concentration.
The brine shrimp lethality bioassay is a simple and inexpensive method for determining the cytotoxicity potential of the plant extracts [Citation44]. Many researchers have reported that the plants showing LC50 value below 20 µg mL−1 possess antitumour and anticancer potential [Citation52]. In the present study, root extract displayed the highest cytotoxicity potential (10.04 ppm) as compared to the stem and leaves extracts (10.86 and 18.32 ppm). Previously, Pai et al. [Citation53] also reported brine shrimp cytotoxicity effects in B. aristata which then expressed the anticancer activity. Thus, it can be suggested that the aerial parts of B. baluchistanica could also be examined against various cancer cell lines to ascertain their potential use.
Many studies have reported that different germination indices and plant root length help evaluate the allelopathic effects of plant extracts [Citation54]. Plants contain phytotoxins which affect seed coat permeability or seed size and seed structure [Citation55]. In the present study, the highest phytotoxicity potential was found in the root extract as compared to the stem and leaves extracts. Previously, several studies [Citation54,Citation55] have reported phenolic and flavonoid compounds as potential candidates for phytotoxicity due to their solubility in water. The present study also indicates that root length is more sensitive (85.35% inhibition) than shoot length (41.30% inhibition), which correlates with the previous findings of Rinez et al. [Citation56] who also determined that roots are more sensitive to the phytotoxic agents. All-inclusive, all parts of B. baluchistanica exhibit potent biological properties and thus can be exploited for pharmaceutical purposes.
5. Conclusion
The present study showed the multitude of elements, functional groups, phenolics and alkaloid compounds in B. baluchistanica extracts. The relative efficacy and toxicity potential of different organs have been assessed using standard protocols. Comparatively, root extracts displayed the highest DPPH activity and TAC while leaves extracts showed the highest antimicrobial activity against S. aureus, K. pneumonia and A. alternata. The root extracts also exhibited the highest brine shrimp cytotoxicity and the radish seed phytotoxicity potential. Besides root extracts, leaves and stem extracts also revealed significant phytochemicals and biological activities. Thus, the selected plant can be preserved by discouraging its uprooting and encouraging the use of stem and leaves for medicinal purposes. However, detailed in vivo studies are recommended to authenticate their safe use as dietary or medicinal products for humans on a larger scale. Novel approaches of extraction and isolation of various compounds need to be established to enhance their potential use.
Ethics approval and consent to participate
This study makes use of human blood and consent was obtained from the person. Study approval for the use of blood was taken (#BEC-FBS-QAU2019-143) from the Bioethical Committee (BEC) of Quaid-i-Azam University, Islamabad (Pakistan).
Disclosure statement
No potential conflict of interest was reported by the author(s).
References
- Fierascu RC, Georgiev MI, Fierascu I, et al. Mitodepressive, antioxidant, antifungal and anti-inflammatory effects of wild-growing Romanian native Arctium lappa L.(Asteraceae) and Veronica persica Poiret (Plantaginaceae). Food Chem Toxicol. 2018;111:44–52.
- Ekor M. The growing use of herbal medicines: issues relating to adverse reactions and challenges in monitoring safety. Front Pharmacol. 2014;4(177):1–10.
- Bodeker G, Ong CK. WHO global atlas of traditional, complementary and alternative medicine. Geneva: World Health Organization. 2005;1.
- Sultan MT, Butt MS, Karim R, et al. Nigella sativa fixed and essential oil supplementation modulates hyperglycemia and allied complications in streptozotocin-induced diabetes mellitus. Evid-Based Complement Alternat Med. 2014;2014:1–8.
- Ahrendt LWA. Berberis and Mahonia: a taxonomic revision. Bot J Linn Soc. 1961;57(369):1–410.
- Khan T, Khan IA, Rehman A. Evaluation and detailing of taxonomic and historical perspectives on genus Berberis from Pakistan. J Bio Env Sci. 2015;6:361–367.
- Tareen RB, Bibi T, Khan MA, et al. Indigenous knowledge of folk medicine by the women of Kalat and Khuzdar regions of Balochistan, Pakistan. Pak J Bot. 2010;42(3):1465–1485.
- Baloch N, Nabi S, Yasser MSA, et al. In vitro antileishmanial, cytotoxic, anti-oxidant activities and phytochemical analysis of Berberis baluchistanica roots extracts and its fractions. J Phytopharmacol. 2013;4:282–287.
- Kakar SA, Tareen RB, Kakar MA, et al. Screening of antibacterial activity of four medicinal plants of Balochistan-Pakistan. Pak J Bot. 2012;44:245–250.
- Zaidi SF, Muhammad JS, Shahryar S, et al. Anti-inflammatory and cytoprotective effects of selected Pakistani medicinal plants in Helicobacter pylori-infected gastric epithelial cells. J Ethnopharmacol. 2012;141(1):403–410.
- Pervez S, Saeed M, Khan H, et al. Nephroprotective effect of Berberis baluchistanica against gentamicin-induced nephrotoxicity in rabbit. Bangladesh J Pharmacol. 2018;13(3):222–230.
- Abbasi MA, Naqvi SSH, Rehman A, et al. Berberis baluchistanica assessment of natural antioxidant to reprieve from oxidative stress. Int Res J Pharm. 2013;4(5):101–105.
- Ahn K. The worldwide trend of using botanical drugs and strategies for developing global drugs. BMB Rep. 2017;50(3):111–116.
- Raj A, Jhariya MK, Yadav DK, et al. Conservation issues, challenges, and management of medicinal plantresources: a new dimension toward sustainable natural resource management. In: Waisundara VY, editor. Agroforestry and climate change. Apple Academic Press; 2019. p. 123–153.
- Zschocke S, Rabe T, Taylor JL, et al. Plant part substitution–a way to conserve endangered medicinal plants? J Ethnopharmacol. 2000;71(1-2):281–292.
- Hseu ZY. Evaluating heavy metal contents in nine composts using four digestion methods. Bioresour Technol. 2004;95(1):53–59.
- Meenambal M, Pughalendy K, Vasantharaja C, et al. Phytochemical information from FTIR and GC-MS studies of methol extract of Delonix elat leaves. Int J Chem Anal Sci. 2012;3(6):1446–1448.
- Abdelkhalek A, Salem MZ, Kordy AM, et al. Antiviral, antifungal, and insecticidal activities of Eucalyptus bark extract: HPLC analysis of polyphenolic compounds. Microb. Pathog. 2020;147:104383.
- Sumczynski D, Bubelova Z, Sneyd J, et al. Total phenolics, flavonoids, antioxidant activity, crude fibre and digestibility in non-traditional wheat flakes and muesli. Food Chem. 2015;174:319–325.
- Stankovic MS, Niciforovic N, Topuzovic M, et al. Total phenolic content, flavonoid concentrations and antioxidant activity, of the whole plant and plant parts extracts from Teucrium montanum L. var. montanum, f. supinum (L.) Reichenb. Biotechnol Biotec Eq. 2011;25(1):2222–2227.
- Ram PR, Mehrotra BN. A compendium of Indian medicinal plants (drug research preparative: A CDRI series). Vol. 1, New Delhi: Lucknow and Publication and Information Directorate; 1993. p. 390.
- Jain A, Soni M, Deb L, et al. Antioxidant and hepatoprotective activity of ethanolic and aqueous extracts of Momordica dioica Roxb. leaves. J Ethnopharmacol. 2008;115:61–66.
- Prieto P, Pineda M, Aguilar M. Spectrophotometric quantitation of antioxidant capacity through the formation of a phosphomolybdenum complex: specific application to the determination of vitamin E. Anal Biochem. 1999;269:337–341.
- Yang ZG, Sun HX, Fang WH. Haemolytic activities and adjuvant effect of Astragalus membranaceus saponins (Ams) on the immune responses to ovalb Sermakkani umin in mice. Vaccine. 2005;23:5196–5203.
- Okoli CO, Akah PA, Onuoha NJ, et al. Acanthus montanus: an experimental evaluation of the antimicrobial, anti-inflammatory and immunological properties of a traditional remedy for furuncles. BMC Complement Altern Med. 2008;8(1):1–11.
- Lee JC, Kim HR, Kim J, et al. Antioxidant property of an ethanol extract of the stem of Opuntia ficus-indica var. saboten. J Agr Food Chem. 2002;50(22):6490–6496.
- Jo C, Park BJ, Chung SH, et al. Antibacterial and antifungal activity of citrus (Citrus unshiu) essential oil extracted from peel by-products. Food Sci Biotechnol. 2004;13:384–386.
- Moshi MJ, Mbwambo ZH. Some pharmacological properties of extracts of Terminalia sericea roots. J Ethnopharmacol. 2005;97:43–47.
- Arzu UT, Camper ND. Biological activity of common Mullein, a medicinal plant. J Ethnopharmacol. 2002;82:117–125.
- Finney DJ. Probit analysis (2nd ed.). J Instrum. 1952;78:388–390.
- Meena AK, Bansal P, Kumar S. Plants-herbal wealth as a potential source of ayurvedic drugs. Asian J Tradit Med. 2009;4(4):152–170.
- Arif N, Yadav V, Singh S, et al. Influence of high and low levels of plant-beneficial heavy metal ions on plant growth and development. Front Environ Sci. 2016;4:69.
- Leśniewicz A, Jaworska K, Żyrnicki W. Macro-and micro-nutrients and their bioavailability in Polish herbal medicaments. Food Chem. 2006;99(4):670–679.
- Bogden JD, Klevay LM. Clinical nutrition of the essential trace elements and minerals: the guide for health professionals. New York: Springer Science & Business Media. 2000.
- Damascos MA, Arribere M, Svriz M, et al. Fruit mineral contents of six wild species of the North Andean Patagonia, Argentina. Biol Trace Elem Res. 2008;125(1):72–80.
- Kumar M, Puri S, Pundir A, et al. Evaluation of nutritional, phytochemical, and mineral composition of selected medicinal plants for therapeutic uses from cold desert of Western Himalaya. Plants. 2021;10(7):1429.
- Akhi MZ, Haque MM, Biswas MS. Role of secondary metabolites to attenuate stress damages in plants. In: Waisundara VY, editor. Antioxidants. London: IntechOpen; 2021. p. 1–16.
- Thusa R, Mulmi S. Analysis of phytoconstituents and biological activities of different parts of Mahonia nepalensis and Berberis aristata. Nepal J Biotechnol. 2017;5(1):5–13.
- Gál B, Bucher C, Burns NZ. Chiral alkyl halides: Underexplored motifs in medicine. Mar Drugs. 2016;14(11):206.
- Chandra S. Fourier transform infrared (Ft-Ir) spectroscopic analysis of Nicotiana plumbaginifolia (Solanaceae). J Med Plants. 2019;7(1):82–85.
- Ashokkumar R, Ramaswamy M. Comparative study on the antimicrobial activity of leaf extracts of four selected Indian medicinal plants against Pseudomonas aeruginosa, Pseudomonas fluorescens, Penicillium chrysogenum and Penicillium restrictum. J Chem Biol Phys Sci. 2013;3(2):1279.
- Abdykerimova S, Sakipova Z, Nakonieczna S, et al. Superior antioxidant capacity of berberis iliensis—HPLC-Q-TOF-MS based phytochemical studies and spectrophotometric determinations. Antioxidants. 2020;9(6):504.
- Ahmad S, Hussain A, Hussain A, et al. Quantification of berberine in Berberis vulgaris L. root extract and its curative and prophylactic role in cisplatin-induced in vivo toxicity and in vitro cytotoxicity. Antioxidants. 2019;8(6):185.
- Fatima I, Akhtar W, Bangash NK, et al. Volatile profiling, elemental composition and biological activities of aerial parts of seven Poaceae species. Plant Biosyst. 2022;156(4):908–925.
- Batool F, Saadullah M, Asif M, et al. Phytochemical and biological screening of root extracts of Berberis baluchistanica. BioCell. 2019;43(2):1–7.
- Bhatt LR, Wagle B, Adhikari M, et al. Antioxidant activity, total phenolic and flavonoid content of Berberis aristata DC. and Berberis thomsoniana CK Schneid. from Sagarmatha National Park, Nepal. Pharmacog J. 2018;10(6s):167–171.
- Ebrahimzadeh MA, Nabavi SF, Nabavi SM, et al. Anti-hemolytic and antioxidant activities of allium paradoxum. Cent Eur J Biol. 2010;5:338–345.
- Moskovitz J, Yim KA, Choke PB. Free radicals and disease. Arch Biochem Biophys. 2002;397:354–359.
- Manandhar S, Luitel S, Dahal RK. In vitro antimicrobial activity of some medicinal plants against human pathogenic bacteria. J Trop Med. 2019;2019 1–5.
- Idrees M, Rehman S, Leghari SK, et al. Antimicrobial activity of three medicinally important plants (Berberis balochistanica, Thymus serpyllum and Salvia bucharica) of Shudden, Mazhoo Tehsil Nana Sab area, District Pishin, Balochistan-Pakistan. Pure Appl Biol. 2021;11(2):570–576.
- Parekh J, Chanda S. In vitro antifungal activity of methanol extracts of some Indian medicinal plants against pathogenic yeast and moulds. Afr J Biotechnol. 2008;7(23):4349–4353.
- Ali A, Haider MS, Hanif S, et al. Assessment of the antibacterial activity of Cuscuta pedicellata Ledeb. Afr J Biotechnol. 2014;13(3):430–433.
- Pai KSR, Srilatha P, Suryakant K, et al. Anticancer activity of Berberis aristata in Ehrlich ascites carcinoma-bearing mice: a preliminary study. Pharm Biol. 2012;50(3):270–277.
- Kiran F, Khan MA, Batool R, et al. Biological evaluation of some important medicinal plants from Poonch valley, Azad Kashmir, Pakistan. J Tradit Chin Med. 2019;39(6):753–763.
- Batish DR, Singh HP, Setia N, et al. 2-Benzoxazolinone (BOA) induced oxidative stress, lipid peroxidation and changes in some antioxidant enzyme activities in mung bean (Phaseolus aureus). Plant Physiol Biochem. 2006;44(11-12):819–827.
- Rinez A, Ladhari A, Omezzine F, et al. Phytotoxicity of Nicotiana glauca Graham aqueous extracts, a Tunisian invasive plant. In 3rd International Symposium on Weeds and Invasive Plants, 2011; 2–7.