ABSTRACT
Methicillin-resistant Staphylococcus aureus (MRSA) is among the top threats to global public health and biofilm production is one of the factors attributed to its pathogenesis. This study aims to investigate the biofilm forming ability of 89 clinical MRSA isolated from various sources in Malaysia and to investigate the presence of biofilm-associated and regulatory genes among these isolates. A total of 89.9% (80/89) isolates produced slime layer via Congo red assay while 57.3% (51/89) isolates produced strong biofilm via crystal violet assay. PCR assay revealed 97.8% (87/89) isolates carried at least one of the MSCRAMM with icaBC genes detected at the highest rate (92.1%, 82/89) and highest frequency of agr-I (68.5%, 61/89). Clinical isolates isolated from pus were significantly associated with strong biofilm (p = 0.0449). The obtained results revealed the varying ability of clinical MRSA isolates to form biofilms and highlighted the genetic background associated with biofilms from different sources and clinical presentations.
1. Introduction
Biofilms play a vital role in the pathogenesis of Staphylococcus aureus as bacteria embedded in biofilms are often difficult to eradicate with antibiotics as well as the host immune responses, as opposed to exposed planktonic cells [Citation1]. S. aureus also produces slime as an extracellular substance to facilitate the bacterial adhesion and biofilm formation [Citation2]. Polysaccharide matrix, cell surface proteins, extracellular DNA (e-DNA), and teichoic acids are the major components that construct the biofilm [Citation1].
Four major events are involved in the formation of biofilms: (i) initial attachment of the cells to biotic or abiotic surfaces, (ii) biofilm accumulation forming multiple bacterial layers, (iii) biofilm maturation with the production of polysaccharide-intercellular adhesins (PIA) and (iv) dispersal of cells in the planktonic state from mature biofilms to spread to new sites elsewhere mediated by quorum sensing, especially the accessory gene regulator, agr [Citation1].
MRSA biofilms are typically composed of extracellular proteins called microbial surface components recognizing adhesive matrix molecules (MSCRAMMs) and e-DNA [Citation3]. Genes involved in the production of MSCRAMMs include genes encoding intercellular adhesion (icaAD, icaBC), collagen-binding protein (cna), fibronectin-binding proteins A and B (fnbA, fnbB), fibrinogen-binding protein (fib), elastin-binding protein (ebps), clumping factors A and B (clfA, clfB), laminin-binding protein (eno), surface-anchored proteins C and G (sasC, sasG), biofilm-associated protein (bap), bone sialoprotein-binding protein (bbp) and plasmin-sensitive protein (pls). Different MSCRAMMs may have different properties and may adhere to different host-tissue components [Citation4].
Investigations on slime production and biofilm formation of MRSA isolates from different countries have been reported elsewhere [Citation5,Citation6]. The infections of MRSA in Malaysian hospitals continue to show resistance to certain antibiotics (e.g. gentamicin, tetracycline, erythromycin, ciprofloxacin, rifampin and chloramphenicol) and is difficult to treat due to their ability to form biofilms [Citation7]. Hence, the aims of the present study were: (i) to investigate slime production and biofilm formation of MRSA collected from various clinical sources from a tertiary hospital in Malaysia and (ii) to examine the potential association between the presence of MSCRAMM genes and agr groups with biofilm formation.
2. Materials and methods
2.1. Bacterial isolates
A total of 89 clinical MRSA consisting of pus (n = 55), blood (n = 27), respiratory secretions (n = 5) and eye (n = 2) isolates, and that were previously characterized were used in this study [Citation7,Citation8]. Skin and soft tissue infections is the most cases (43.8%, 39/89) associated with MRSA infections in this study. These isolates were obtained from main tertiary hospital with a total of 821 beds in Terengganu state, Malaysia, namely Hospital Sultanah Nur Zahirah, from July 2016 to June 2017. The study population consisted of 43% female and 57% male, and the age of patients ranged between 3 weeks to 82 years with a mean of 41 years.
2.2. Detection of slime production using Congo red assay
Using a spot inoculation method, 4 µL of bacterial suspension was inoculated on the Congo red agar (CRA) plate and incubated at 37°C for 24 and 48 h [Citation9,Citation10]. Results interpretation: (i) rough black spots (positive slime producer, score ++), (ii) a mixture of black and red spots (intermediate slime producer, score +), and (iii) smooth red spots (negative slime producer, score -) [Citation9].
2.3. Detection of biofilm forming ability using crystal violet staining
A total of 200 µL of the overnight diluted culture was inoculated into microtitre plate followed by incubation at 37°C for 24 h. Next, planktonic culture was measured at 600 nm. The adhered cells were fixed with absolute ethanol at room temperature followed by 200 µL of 0.5% (w/v) crystal violet staining. The acetic acid was added for solubilization and measured at 570 nm [Citation11]. Staphylococcus aureus ATCC 35556 and Staphylococcus epidermidis ATCC 12228 were used as positive and negative controls, respectively [Citation12]. Isolates were calculated using specific biofilm formation (SBF) index formula: SBF = (AB – CW)/G, where AB is the OD570nm of the adhered and stained bacteria, CW is the OD570nm of the stained control wells containing only sterile medium, and G is the OD600nm of cells growth in broth. The degree of biofilm formation was determined as follows; weak biofilm producer (SBF ≤ 0.5), intermediate biofilm producer (0.5 < SBF ≤ 1) and strong biofilm producer (SBF > 1) [Citation13].
2.4. DNA extraction
Total genomic DNA was extracted from overnight cultures using the in-house boiling method and subjected to quality and quantity measurement [Citation11]. Briefly, overnight culture was centrifuged and supernatant was discarded. The pellet was washed with sterile distilled water followed by centrifugation and supernatant was discarded. A total of 100 µL of sterile distilled water was added to resuspend the pellet and followed by boiling at 95°C for 10 min. The cell lysate was incubated on ice for another 10 min and followed by centrifugation. The supernatant was transferred to a new sterile microcentrifuge tube and stored in −20°C.
2.5. Detection of adhesin genes (MSCRAMMs)
DNA amplification of 15 MSCRAMM-associated genes (bap, bbp, clfA, clfB, cna, eno, ebps, fib, fnbA, fnbB, icaAD, icaBC, pls, sasC, and sasG) was performed by multiplex PCR using primers as described in [Citation14] and [Citation15] using the thermal cycling condition (Supplementary Table 1). The thermal cycling condition: one cycle at 95°C (3 min), 30 cycles at 95°C (1 min), 52°C (45 s) and 72°C (45 s), with a final extension at 72°C (10 min).
2.6. Accessory gene regulatory (agr) typing by PCR
The agr typing was carried out using primers as previously described [Citation16] and similar thermal cycling condition (Supplementary Table 1). The thermal cycling condition: one cycle at 95°C (3 min), 35 cycles at 95°C (1 min), 60°C (45 s) and 72°C (45 s), with a final extension at 72°C (10 min).
2.7. Data analysis
Data analysis was carried out using Grubbs’ test, dot plots, Fisher’s exact test (GraphPad Prism Version 9.1.2). The differences at p < 0.05 calculated with two tails were considered significant [Citation17].
3. Results
3.1. Phenotypic detection of slime production on Congo red agar
Of 89 isolates, 89.9% (n = 80) isolates exhibited the ability to produce slime (Table ). As shown in Table , a total of 10.1% (9/89) are categorized as positive slime producers (5 pus isolates, 4 blood isolates), 79.8% (71/89) are intermediate slime producers (45 pus isolates, 19 blood isolates, 5 respiratory isolates, 2 eye isolates) while 10.1% (9/89) are negative slime producers (5 pus isolates, 4 blood isolates).
Table 1. Slime phenotypes on Congo red agar.
Table 2. Association between patient’s characteristics, slime production and biofilm formation in MRSA isolates.
3.2. Detection of biofilm forming ability using crystal violet
All isolates tested were found to be adherent by varying degrees in the biofilm formation assay with SBF values that ranged from 0.173–5.478 (Figure ). Among all isolates, 57.3% (51/89) were categorized as strong biofilm producers (31 pus isolates, 17 blood isolates, 2 respiratory isolates, 1 eye isolate) with SBF range: 1.188–5.478. A total of 33.7% (30/89) showed SBF range: 0.574–0.851 were intermediate producers (16 pus isolates, 10 blood isolates, 3 respiratory isolates, 1 eye isolate) while 9.0% (8/89) showed SBF range: 0.173–0.430 were weak biofilm producers (8 pus isolates). Interestingly, clinical isolates isolated from pus were significantly associated with strong biofilm production (p = 0.0449). Of 51 strong biofilm-producing isolates, only 13.7% (7/51) MRSA showed a positive slime production on Congo red agar.
3.3. Prevalence of MSCRAMM genes
A total of 97.8% (87/89) clinical isolates harboured at least one of the MSCRAMM genes (Figure ). Three genes – i.e. bap, pls and bbp were not detected in all isolates. The most predominant genes present were icaBC (92.1%, 82/89) followed by sasG (88.8%, 79/89), eno (79.8%, 71/89), clfA (79.8%, 71/89), icaAD (78.7%, 70/89) and clfB (76.4%, 68/89). The frequency of other MSCRAMM genes (cna, fib, ebps, sasC, fnbA, and fnbB) ranged from 1.1% to 42.7%.
Figure 2. Representation of MSCRAMM genes amplified products on 1% (w/v) agarose gel electrophoresis. Lane L: 100 bp plus molecular weight marker; lane 1: Triplex 1 – cna (423 bp), eno (302 bp), ebps (186 bp); lane 2: Triplex 2 – sasC (936 bp), icaAD (520 bp), icaBC (182 bp); lane 3: Triplex 3 – fib (404 bp), clfA (292 bp), sasG (159 bp); lane 4: fnbA (643 bp); lane 5: fnbB (524 bp); lane 6: clfB (205 bp).
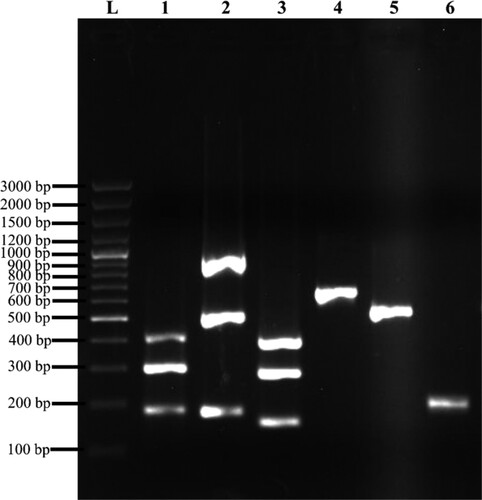
3.4. Detection of accessory gene regulator
Of 89 clinical isolates, 85.4% (76/89) were assigned to specific agr groups whereas 11.2% (10/89) isolates were untypable. The frequency of agr-typable isolates were: agr-I (68.5%, 61/89), agr-II (9.0%, 8/89) and agr-III (7.9%, 7/89). No agr-IV was detected. Three other isolates demonstrated the coexistence of two agr groups (agr-I and agr-III) (Table ).
Table 3. Association between slime production, biofilm formation, MSCRAMM genes distribution and accessory gene regulator in MRSA isolates.
3.5. Association of slime production, biofilm formation, MSCRAMM genes and agr among MRSA
The positive slime-producing isolates were significantly associated with carriage of the clfB (p = 0.0398), fib (p = 0.0176) and sasC (p = 0.0184) genes. Meanwhile, strong biofilm-producing isolates were significantly associated with the presence of the icaBC (p = 0.026) and cna (p = 0.0088) genes. With respect to agr groups, positive slime-producing isolates were significantly associated with agr-I (p = 0.0028) and agr-III (p = 0.0179). However, no statistically significant association was observed between biofilm formation and agr groups (Table ).
4. Discussion
Biofilm formation is a process where a community of microbial cells is attached to a surface and enclosed in a self-produced exopolysaccharide glycocalyx [Citation1]. Our results showed that the majority of the 89 MRSA (80/89, 89.9%) isolates were strong slime producers as they produced black-coloured colonies after 24 h on CRA. The intermediate slime producers (79.8%, 71/89) which exhibit variation of reddish black colonies after 24 h have developed black colonies after 48 h. As the phenotypic slime production analyses using CRA were reported to be highly subjective, crystal violet assay on microtitre plates has been used widely to examine biofilm production in bacterial isolates [Citation5,Citation18]. In this study, all clinical MRSA isolates isolated from various sources showed an ability to form biofilm ranging from weak, intermediate and strong (Table ). Our results showed that positive slime producers of MRSA do not necessarily imply that the isolates will be a biofilm producer in the microtitre plate biofilm assay and this finding was in agreement with previous studies [Citation19,Citation20]. The OD value measured in the biofilm assay is a reflection of the number of bacteria present and is not an indicator of slime production. Among nine positive slime producers, seven isolates were strong biofilm producers while two isolates were intermediate biofilm producers. The observation suggested that biofilm production depends on growth conditions and various sugar supplementation [Citation21,Citation22].
In this study, all 89 clinical MRSA isolates are biofilm producers at varying degrees (SBF: 0.173–5.478) and clinical MRSA isolates isolated from pus had a significantly greater ability to produce strong biofilm formation (p = 0.0449). This is in agreement with a previous study in which clinical isolates isolated from superficial or deep tissues showed significantly enhanced biofilm formation [Citation23]. Localized infections with thick fluid in deep solid tissues may favour the survival of the biofilm-producing organisms compared to blood and urine. Once the bacteria adhere firmly to the deep tissue with localized infection, it will become more difficult to be eradicated as it provides an environment conducive for their protection and longevity [Citation23]. Interestingly, one isolate isolated from the pus of patient with osteoarticular infections showed a weak biofilm formation but harboured most of the MSCRAMM genes (11/15). Yu et al. [Citation24] had reported no significant difference in biofilm formation between osteomyelitis and non-osteomyelitis isolates among orthopaedic S. aureus isolates. Thus, it is hypothesized that although S. aureus relies on protein-to-protein interactions for attachment, the presence of MSCRAMM genes was not directly related to the ability of isolates to form biofilms. Other environmental factors such as proteins, ions, stress factors, metabolic activity or host immunity could interfere as the biofilm development proceeds [Citation25].
Overall, 97.8% of the Terengganu MRSA isolates carried at least one MSCRAMM gene. The frequency of icaAD and icaBC were relatively high (78.7% and 92.1%, respectively) and these isolates were shown to be capable of forming strong to intermediate biofilms at 13 times higher than those weak biofilm producers. Recently, a study conducted by Niek et al. [Citation26] also reported high frequency of icaA (75.8%) and icaD (100%) in strong biofilm producers using MRSA isolates (n = 36) collected in 2014 from a teaching hospital (University Malaya Medical Centre) located in Kuala Lumpur. Moreover, the observation of a significant association of icaBC and strong biofilm producers is in agreement with previous studies that the polysaccharide matrix (PIA) encoded by the ica operon is required for biofilm formation [Citation4,Citation27]. However, our result also showed that 6 MRSA isolates that were negative for icaAD and icaBC were able to form biofilms (weak producers = 2, intermediate producers = 3, strong producers = 1). Similar observation was reported by previous study conducted in Iran by Ghaioumy et al. (2021) where negative icaADBC genes were seen in their biofilm producing isolates [Citation28]. This suggests that the absence or presence of icaADBC does not represent a clear discriminative marker in differentiating the biofilm-forming ability of S. aureus isolates. A minor proportion of S. aureus isolates continue to form biofilms in ica-independent pathways even after the deletion of the ica locus [Citation26,Citation29]. Therefore, it is suggested the role of extracellular polymeric substances other than PIA such as e-DNA and teichoic acids may be responsible for the biofilm matrix formation of these six isolates.
Apart from icaBC, 42.7% (38/89) of the clinical MRSA isolates in this study carried the cna gene and was significantly associated with strong biofilm producers. Similar findings were observed by Chen et al. [Citation30] who reported that 20% of cna-positive isolates were identified as moderate to strong biofilm producers. Furthermore, the cna gene was more common in invasive S. aureus isolates among other MSCRAMM genes which explained the robust biofilm formation in the presence of cna gene [Citation31]. Besides, fnbA and fnbB genes were reportedly essential in biofilm maturation and were also involved in PIA adhesion [Citation5]. However, among our Malaysian S. aureus isolates, only 9% (8/89) carried the fnbA gene while even a lower 1.1% (1/89) harboured the fnbB gene. A similarly low frequency of detection of the fnbA gene (7.2%) was reported in S. aureus isolates that were isolated from the nose swabs of healthy individuals working in Iranian hospitals [Citation32].
Another gene, bap, was known to encode the first protein to promote biofilm accumulation in S. aureus [Citation33]. However, it was not detected in any human clinical isolates in our study which is in accordance with previous studies [Citation6,Citation34]. The presence of the bap gene was first described in S. aureus isolates that caused bovine mastitis and was also observed in a few sheep mastitis isolates but rarely in human isolates [Citation5].
The clumping factors encoded by the clfA and clfB genes are responsible for the clumping of S. aureus to fibrinogen and fibrin under calcium-depleted conditions [Citation5,Citation35]. In this study, among the 89 S. aureus isolates, 79.8% (71/89) carried the clfA and 76.4% (68/89) harboured the clfB gene. The binding of ClfA to fibrinogen reduces the probability of S. aureus being eliminated by neutrophils [Citation36]. Furthermore, ClfB is capable of binding to keratin 10 which is the largest component of squamous cells and was reported to contribute to nasal colonization [Citation37].
Laminin is an important component of the blood vessel basement. The binding of MRSA to laminin of the blood vessel via the product of the eno gene enables the dissemination of MRSA in the host through the bloodstream to initiate tissue colonization at different sites. A high proportion on our MRSA isolates (79.8%, 71/89) harboured the eno gene. This is in agreement with an earlier study that reported an even higher occurrence (94.8%) of this gene among Palestinian MRSA isolates regardless of their clinical origins [Citation6]. Another gene that has shown a crucial role in binding to the extracellular cell matrix is the elastin binding protein (encoded by the ebps gene) which was found in 23.6% (21/89) of our MRSA isolates. The ebps gene was reported to facilitate the binding of S. aureus to elastin-rich tissues such as the lung, skin and blood vessels, thus promoting its colonization in mammalian tissues [Citation38].
In this study, the agr groups were detected in 79 out of 89 clinical isolates, but not in the remaining 10 isolates, which were thus referred to as non-typable. This may be due to a true agr deficiency or arise by mutations during infection [Citation39]. Shopsin et al. [Citation40] reported that 9% of healthy human subjects are colonized with agr-defective isolates, hence were unable to be detected by PCR assay. Apart from that, Soong et al. [Citation41] also reported 22% (29/133) of S. aureus expressed agr mutant-like phenotype from chronically infected patients with atopic dermatitis. The agr-I was the most dominant agr type in this study and in accordance with previous studies done in Malaysia and Middle East countries [Citation26,Citation42]. The agr-I isolates were usually associated with endocarditis, septicemia and necrotizing pneumonia [Citation43]. However, in this study, no significant association was observed between agr types with the clinical manifestation of the isolates. Three isolates showed co-existence of two agr types (agr-I and agr-III) and this could be due to inter-strain recombination events or co-infection by two distinct MRSA lineages. The presence of two agr types within a strain had occasionally been observed previously, but despite the co-existence, no report has shown the infectivity and increased virulence of the strain [Citation39,Citation44].
5. Conclusion
In summary, this study revealed that clinical MRSA isolates from Terengganu, Malaysia, produce biofilms by varying degrees regardless of their slime abilities. The clinical isolates isolated from pus showed a significant capacity for forming a strong biofilm. All isolates showed variation in the distribution of MSCRAMM genes and isolates carrying the icaBC and cna genes were significantly associated with strong biofilm producers. The heterogeneity in the genetic background may have played a role in the biofilm ability among clinical MRSA isolates.
Ethical approval
The study was approved by the Malaysian Ministry of Health, National Medical Research Registry (NMRR) and ethics committee with a reference number: NMRR-15-2369-28130 (IIR) for clinical isolates and data collection.
Supplemental Material
Download MS Word (38.7 KB)Acknowledgments
We are grateful to the Clinical Research Centre and the Microbiology Laboratory, Hospital Sultanah Nur Zahirah for providing clinical isolates.
Disclosure statement
No potential conflict of interest was reported by the author(s).
Correction Statement
This article has been republished with minor changes. These changes do not impact the academic content of the article.
Additional information
Funding
References
- Mukherji R, Patil A, Prabhune A. Role of extracellular proteases in biofilm disruption of gram-positive bacteria with special emphasis on staphylococcus aureus biofilms. Enzym Eng. 2015;4(1):1000126. doi:10.4172/2329-6674.1000126.
- Podbielska A, Galkowska H, Stelmach E, et al. Slime production by Staphylococcus aureus and Staphylococcus epidermidis strains isolated from patients with diabetic foot ulcers. Arch Immunol Ther Exp (Warsz). 2010;58(4):321–324. doi:10.1007/s00005-010-0079-9.
- Otto M. MRSA virulence and spread. Cell Microbiol. 2012;14(10):1513–1521. doi:10.1111/j.1462-5822.2012.01832.x.
- Foster TJ, Geoghegan JA, Ganesh VK, et al. Adhesion, invasion and evasion: the many functions of the surface proteins of Staphylococcus aureus. Nat Rev Microbiol. 2014;12(1):49–62. doi:10.1038/nrmicro3161.
- Achek R, Hotzel H, Nabi I, et al. Phenotypic and molecular detection of biofilm formation in Staphylococcus aureus isolated from different sources in Algeria. Pathogens. 2020;9(2):153. doi:10.3390/pathogens9020153.
- Azmi K, Qrei W, Abdeen Z. Screening of genes encoding adhesion factors and biofilm production in methicillin resistant strains of Staphylococcus aureus isolated from Palestinian patients. BMC Genomics. 2019;20(1):578. doi:10.1186/s12864-019-5929-1.
- Hamzah AM C, Yeo CC, Puah SM, et al. Staphylococcus aureus infections in Malaysia: A review of antimicrobial resistance and characteristics of the clinical isolates, 1990-2017. Antibiotics. 2019;8(3):128. doi:10.3390/antibiotics8030128.
- Jones SU, Chua KH, Chew CH, et al. spa diversity of methicillin-resistant and -susceptible staphylococcus aureus in clinical strains from Malaysia: a high prevalence of invasive European spa-type t032. PeerJ. 2021;9:e11195. doi:10.7717/peerj.11195.
- Osman KM, Amer AM, Badr JM, et al. Antimicrobial resistance, biofilm formation and mecA characterization of methicillin-susceptible S. aureus and non-S. aureus of beef meat origin in Egypt. Front Microbiol. 2016;7:222. doi:10.3389/fmicb.2016.00222.
- Kaiser TD, Pereira EM, Dos Santos KR, et al. Modification of the Congo red agar method to detect biofilm production by Staphylococcus epidermidis. Diagn Microbiol Infect Dis. 2013;75(3):235–239. doi:10.1016/j.diagmicrobio.2012.11.014.
- Puah SM, Tan JAMA, Chew CH, et al. Diverse profiles of biofilm and adhesion genes in Staphylococcus aureus food strains isolated from sushi and sashimi. J Food Sci. 2018;83(9):2337–2342. doi:10.1111/1750-3841.14300.
- Kwasny SM, Opperman TJ. Static biofilm cultures of gram-positive pathogens grown in a microtiter format used for anti-biofilm drug discovery. Curr Protoc Pharmacol. 2010;Chapter 13:13A.1–13A.8. doi:10.1002/0471141755.ph13a08s50.
- Naves P, del Prado G, Huelves L, et al. Measurement of biofilm formation by clinical isolates of Escherichia coli is method-dependent. J Appl Microbiol. 2008;105(2):585–590. doi:10.1111/j.1365-2672.2008.03791.x.
- Tristan A, Ying L, Bes M, et al. Use of multiplex PCR to identify Staphylococcus aureus adhesins involved in human hematogenous infections. J Clin Microbiol. 2003;41(9):4465–4467. doi:10.1128/JCM.41.9.4465-4467.2003.
- Sharma V, Sharma S, Dahiya DK, et al. Coagulase gene polymorphism, enterotoxigenecity, biofilm production, and antibiotic resistance in Staphylococcus aureus isolated from bovine raw milk in north west India. Ann Clin Microbiol Antimicrob. 2017;16(1):65. doi:10.1186/s12941-017-0242-9.
- Lina G, Boutite F, Tristan A, et al. Bacterial competition for human nasal cavity colonization: role of Staphylococcal agr alleles. Appl Environ Microbiol. 2003;69(1):18–23. doi:10.1128/AEM.69.1.18-23.2003.
- McDonald, J.H. Handbook of biological statistics. 3rd ed. MD: Sparky House Publishing, Baltimore, Maryland; 2014.
- Bissong MEA, Ateba CN. Genotypic and phenotypic evaluation of biofilm production and antimicrobial resistance in Staphylococcus aureus isolated from milk, north west province. South Africa. Antibiotics (Basel). 2020;9(4):156. doi:10.3390/antibiotics9040156.
- Oliveira M, Nunes SF, Carneiro C, et al. Time course of biofilm formation by Staphylococcus aureus and Staphylococcus epidermidis mastitis isolates. Vet Microbiol. 2007;124(1-2):187–191. doi:10.1016/j.vetmic.2007.04.016.
- Jain A, Agarwal A. Biofilm production, a marker of pathogenic potential of colonizing and commensal staphylococci. J Microbiol Methods. 2009;76(1):88–92. doi:10.1016/j.mimet.2008.09.017.
- Lade H, Park JH, Chung SH, et al. Biofilm formation by Staphylococcus aureus clinical isolates is differentially affected by glucose and sodium chloride supplemented culture media. J Clin Med. 2019;8(11):1853. doi:10.3390/jcm8111853.
- Manandhar S, Singh A, Varma A, et al. Biofilm producing clinical Staphylococcus aureus isolates augmented prevalence of antibiotic resistant cases in tertiary care hospitals of Nepal. Front Microbiol. 2018;9:2749. doi:10.3389/fmicb.2018.02749.
- Sanchez Jr CJ, Mende K, Beckius ML, et al. Biofilm formation by clinical isolates and the implications in chronic infections. BMC Infect Dis. 2013;13:47. doi:10.1186/1471-2334-13-47.
- Yu S, Jiang B, Jia C, et al. Investigation of biofilm production and its association with genetic and phenotypic characteristics of OM (osteomyelitis) and non-OM orthopedic Staphylococcus aureus. Ann Clin Microbiol Antimicrob. 2020;19(1):10. doi:10.1186/s12941-020-00352-4.
- Coraça-Huber DC, Kreidl L, Steixner S, et al. Identification and morphological characterization of biofilms formed by strains causing infection in orthopedic implants. Pathogens. 2020;9(8):649. doi:10.3390/pathogens9080649.
- Niek WK, Teh CSJ, Idris N, et al. Investigation of biofilm formation in methicillin-resistant Staphylococcus aureus associated with bacteraemia in a tertiary hospital. Folia Microbiol (Praha). 2021;66(5):741–749. doi:10.1007/s12223-021-00877-x.
- Nguyen HTT, Nguyen TH, Otto M. The staphylococcal exopolysaccharide PIA - biosynthesis and role in biofilm formation, colonization, and infection. Comput Struct Biotechnol J. 2020;18:3324–3334. doi:10.1016/j.csbj.2020.10.027.
- Ghaioumy R, Tabatabaeifar F, Mozafarinia K, et al. Biofilm formation and molecular analysis of intercellular adhesion gene cluster (icaABCD) among Staphylococcus aureus strains isolated from children with adenoiditis. Iran J Microbiol. 2021;13(4):458–463. doi:10.18502/ijm.v13i4.6969.
- Arciola CR, Campoccia D, Ravaioli S, et al. Polysaccharide intercellular adhesin in biofilm: structural and regulatory aspects. Front Cell Infect Microbiol. 2015;5:7. doi:10.3389/fcimb.2015.00007.
- Chen Q, Xie S, Lou X, et al. Biofilm formation and prevalence of adhesion genes among Staphylococcus aureus isolates from different food sources. Microbiologyopen. 2020;9(1):e00946. doi:10.1002/mbo3.946.
- Peacock SJ, Moore CE, Justice A, et al. Virulent combinations of adhesin and toxin genes in natural populations of Staphylococcus aureus. Infect Immun. 2002;70(9):4987–4996. doi:10.1128/iai.70.9.4987-4996.2002.
- Soltani E, Farrokhi E, Zamanzad B, et al. Prevalence and distribution of adhesins and the expression of fibronectin-binding protein (FnbA and FnbB) among Staphylococcus aureus isolates from Shahrekord hospitals. BMC Res Notes. 2019;12(1):49. doi:10.1186/s13104-019-4055-0.
- Latasa C, Solano C, Penadés JR, et al. Biofilm-associated proteins. C R Biol. 2006;329(11):849–857. doi:10.1016/j.crvi.2006.07.008.
- Parastan R, Kargar M, Solhjoo K, et al. A synergistic association between adhesion-related genes and multidrug resistance patterns of Staphylococcus aureus isolates from different patients and healthy individuals. J Glob Antimicrob Resist. 2020;22:379–385. doi:10.1016/j.jgar.2020.02.025.
- Ganesh VK, Rivera JJ, Smeds E, et al. A structural model of the Staphylococcus aureus ClfA-fibrinogen interaction opens new avenues for the design of anti-staphylococcal therapeutics. PLoS Pathog. 2008;4(11):e1000226. doi:10.1371/journal.ppat.1000226.
- Flick MJ, Du X, Prasad JM, et al. Genetic elimination of the binding motif on fibrinogen for the S. aureus virulence factor ClfA improves host survival in septicemia. Blood. 2013;121(10):1783–1794. doi:10.1182/blood-2012-09-453894.
- Mulcahy ME, Geoghegan JA, Monk IR, et al. Nasal colonisation by Staphylococcus aureus depends upon clumping factor B binding to the squamous epithelial cell envelope protein loricrin. PLoS Pathog. 2012;8(12):e1003092. doi:10.1371/journal.ppat.1003092.
- Downer R, Roche F, Park PW, et al. The elastin-binding protein of Staphylococcus aureus (EbpS) is expressed at the cell surface as an integral membrane protein and not as a cell wall-associated protein. J Biol Chem. 2002;277(1):243–250. doi:10.1074/jbc.M107621200.
- Traber KE, Lee E, Benson S, et al. Agr function in clinical Staphylococcus aureus isolates. Microbiology (Reading). 2008;154(Pt 8):2265–2274. doi:10.1099/mic.0.2007/011874-0.
- Shopsin B, Drlica-Wagner A, Mathema B, et al. Prevalence of agr dysfunction among colonizing Staphylococcus aureus strains. J Infect Dis. 2008;198(8):1171–1174. doi:10.1086/592051.
- Soong G, Paulino F, Wachtel S, et al. Methicillin-resistant Staphylococcus aureus adaptation to human keratinocytes. mBio. 2015;6(2):e00289–15. doi:10.1128/mBio.00289-15.
- Vali L, Dashti AA, Mathew F, et al. Characterization of heterogeneous MRSA and MSSA with reduced susceptibility to chlorhexidine in Kuwaiti hospitals. Front Microbiol. 2017;8:1359. doi:10.3389/fmicb.2017.01359.
- Jarraud S, Mougel C, Thioulouse J, et al. Relationships between Staphylococcus aureus genetic background, virulence factors, agr groups (alleles), and human disease. Infect Immun. 2002;70(2):631–641. doi:10.1128/IAI.70.2.631-641.2002.
- Ghasemzadeh-Moghaddam H, Ghaznavi-Rad E, Sekawi Z, et al. Methicillin-susceptible Staphylococcus aureus from clinical and community sources are genetically diverse. Int J Med Microbiol. 2011;301(4):347–353. doi:10.1016/j.ijmm.2010.10.004.