Abstract
Obesity is a global public health concern brought on by a combination of excessive dietary intake, inactivity and genetic predisposition. Pyridopyrimidines have received considerable interest in the development of obesity and diabetes. Mannich reaction was applied on 2-thioxo-pyridopyrimidinone derivative with a variety of aromatic and heterocyclic aromatic amines and formaldehyde by grinding at 25°C in the presence of HCl to obtain 18 fused thiadiazinones and two bis-fused thiadiazinone derivatives. Additionally, in silico docking was performed to investigate the mode of actions of the 20 synthesized compounds against fat mass and obesity-associated (FTO) protein. The ligand molecules are nicely docked to the target FTO with binding energies (ΔGbind) ranging from −11.6 to −8.0 kcal/mol. Electrostatic potential map of semiempirical optimized compound 16 was performed using Gaussian 03. Interestingly, two bis-fused thiadiazinone derivatives showed marked amelioration of adiposity in the high-fat diet model especially in hepatic and adipose tissues.
1. Introduction
Obesity is an international public health threat which caused by a combination of excess of food intake with lack of genetic susceptibility and physical activity [Citation1–3]. In addition, the expression fat mass and obesity-associated (FTO) gene is correlated with regulation of food intake. By altering the m6A level of hormones associated to eating, FTO protein contributes to the development of obesity [Citation2,Citation3]. Therefore, FTO protein was chosen as a therapeutic target for identification of anti-obesity agents using molecular docking approach.
The wide range of biological and pharmacological activities of pyridopyrimidines, including their application as anti-inflammatory, anti-folate, antiviral, antibacterial and anticancer agents, has garnered considerable attention in recent years [Citation4–9]. Also, pyridopyrimidines are used as inhibitors for hepatitis B virus, PDE IV and tyrosine kinase [Citation9–11]. Furthermore, they also play a crucial role in the metabolism of fatty acids, carbohydrates and amino acids [Citation12–16]. As a result, they are implicated in the treatment of diseases such as type 2 diabetes and obesity [Citation17]. They have received considerable interest in the development of therapeutics for a variety of human diseases, including obesity, diabetes, metabolic syndrome, microbial infections and cancer [Citation18,Citation19], as shown in .
The Mannich reaction is a crucial step in the synthesis of numerous bioactive compounds and is frequently utilized in the production of secondary and tertiary amine derivatives [Citation6,Citation20,Citation21]. A variety of prospective pharmacological properties, such as antifungal, antitubercular, anticancer, neuroprotection and antibacterial activity, are displayed by N-Mannich bases generated from NH-heterocycles and related compounds [Citation22–25].
Bioactive chemicals have been created via multicomponent reactions (MCRs), which combine several reactants in a single vessel [Citation26–33]. They are crucial for creating structural diversity and libraries of drug-like compounds in industrial, synthetic, green and medicinal chemical applications [Citation34]. Therefore, synthetic organic chemists are paying close attention to the discovery of new MCRs and/or the improvement of established MCRs for the synthesis of novel molecules [Citation35]. An increasing consensus is focused at carrying out organic reactions in solvent-free reaction conditions due to rigorous environmental issues and green chemistry [Citation36]. These reactions are growing in popularity as a result of their numerous benefits, including cost savings, decreased energy usage, sped-up reaction times, significant reactor size reductions and reduced capital expenditure, making the reactions cleaner, safer and simpler in this way. The explicit statement made by Sheldon [Citation37] that “The ideal solvent (diluent) is water, while the best solvent is none at all” is significant. Additionally, solvent-free processes’ environmental factor (E Factor) makes them excellent candidates for clean technology [Citation38].
In this vein, we have been working on a programme for the past 10 years that aims to multicomponent one pot synthesize some new heterocyclic structures of biological interest [Citation28–32,Citation39–47] to identify new drug candidates which can be used as anti-obesity inhibitors, through interaction with the target protein, as represented in . Additionally, the molecular docking studies and biological activity of the tested compounds were performed. Finally, the physiochemical and pharmacokinetics parameters of the best docked derivatives are calculated using free website tools.
2. Results and discussion
2.1. Chemistry
2-Thioxo-pyridopyrimidinone derivative 1 [Citation48] reacts with the aromatic amines 2a-m and two equivalents of formaldehyde 3 via the Mannich reaction conditions; grinding at 25°C in the presence of HCl to afford fused thiadiazinone derivatives 4a-m, respectively (Scheme 1).
Likewise thione 1 and two equivalents of formaldehyde 3 were allowed to react with the different heterocyclic amines 5, 7, 9, 11 and 13 under the same reaction conditions to give the fused thiadiazinones 6, 8, 10, 12 and 14, respectively (Scheme 2).
Finally, compounds 1 (2 equivalents) and 3 (4 equivalents) were allowed to react with one equivalent of p-phenylenediamine 15 or p,p`-diaminodiphenylmethane 17 under the same Mannich reaction conditions to afford the bis-pyrido[2′,3′:4,5]pyrimido[2,1-b]thiadiazinones derivatives 16 and 18 (Scheme 3).
2.2. Docking study
In the present study, FTO protein is associated reproducibly with human body mass. Therefore, FTO protein (PDB ID: 3LFM) was chosen as a therapeutic target for identification of anti-obesity agents. Herein, molecular docking approach [Citation49–58] of the newly synthesized compounds 4–18 was performed for identifying new obesity inhibitors. The molecular docking software PyRx was used to calculate the binding affinity of the prepared compounds to the active site pocket of the target. The 3D binding modes of intermolecular interactions between the compounds with highest affinities 16 and 18 with the target are represented in . showed the docking affinities (ΔGbind) of the best docked complexes. The ligand molecules were successfully docked to the active site residues of the target through a network of non-covalent interactions such as H-bonding and/or π stacking as shown in . Upon the docking simulation, it has been shown that all compounds are nicely docked to the target FTO, with binding energies (ΔGbind) ranging from −11.6 to −8.0 kcal/mol.
Figure 3. Three-dimensional interactions of the best docked compounds 16 and 18 with the target. The ligand molecules and the residues are shown in grey stick models. H-bonds and pi-stacking are represented in green dotted lines and orange lines, respectively.
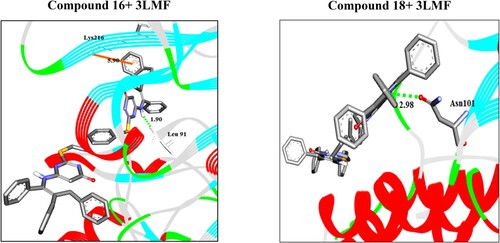
Table 1. Energy-based interactions involving the protein and the high ranked docked molecules. B.E., or calculated free binding energy.
The ligand molecule 16 with binding energy (−11.6 kcal/mol), docked to the target protein through one arene-cation interactions and one hydrogen bonds with the residues Lys216 and Leu91 at 5.90 and 1.90 Å, respectively. In addition, compound 18 docked to the target through one hydrogen bond with the residue Asn101 at 2.98 Å.
Redocking of 3-methylthymidine (co-crystalized ligand) was performed to validate the docking process (RMSD < 2 Å) and the obtained results showed similar fitness to the docked compounds. In addition, the electrostatic potential map of semiempirical was performed to investigate the molecular interactions of the best docked compound 16 to the target. The 3D electrostatic potential map reflects the arene-cation interaction between phenyl moiety of compound 16 and the lysine residue, as shown in . Further, by applying Lipinski rule of five (Ro5) to these ligand molecules, the best compounds 16 and 18 obeyed Ro5, as tabulated in . Furthermore, the absorption rates of these compounds exceeded 99%, indicating that these compounds possess excellent absorption and distribution properties. Moreover, the results have presented that they are non-toxic and non-carcinogenic. These results shed light on bis-fused thiadiazinones 16 and 18 as potent anti-obesity drug candidates.
Figure 4. Electrostatic potential (ESP) map of compound 16. The high electron density charges (electronegative) represented by the red colored region, while pale yellow represents electropositive part.
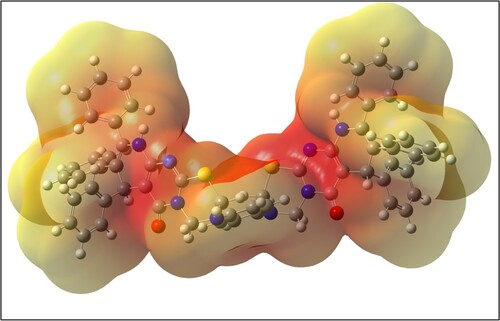
Table 2. List of physicochemical properties and ADME of the best docked compounds.
2.3. Biological study
2.3.1. Histopathological findings of specific organs (liver and adipose tissue)
The liver of control animal showed normal hepatic parenchyma. The hepatocytes were arranged in cords-like manner around the central vein and with normal portal structures. Each hepatocyte was separated with hepatic sinusoids with few Kuffer’s cells. The liver of the adiposity group showed marked granular hepatic cell swelling with marked intrahepatic fatty changes associated with clear and round vacuoles mostly. The sinusoids showed moderate congestion with prominent Kuffer’s cell. The diseased groups treated with bis-fused thiadiazinones 16 and 18 demonstrated marked decrease in vascular congestion and hepatic steatosis (Figures and ).
Figure 5. Liver sections of different groups. (A) control group, (B) adiposity group (arrows indicate fatty change within the hepatocytes), (C) bis-fused thiadiazinones 16 group (arrow indicate marked decrease the fatty change within the hepatocytes) and (D) bis-fused thiadiazinones 18 group (arrow indicate marked decrease the fatty change within the hepatocytes), CV mean central vein. H&E stain, bar = 50 µm.
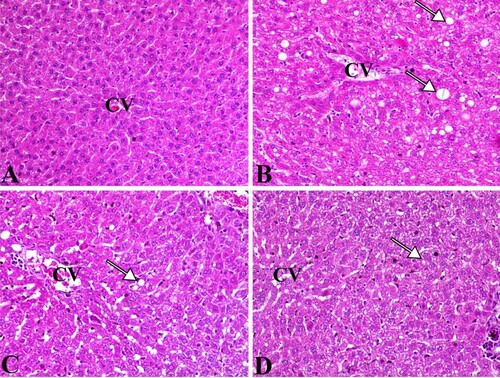
Figure 6. Hepatic steatosis lesion score of different groups expressed mean ± SD, * indicates significance in comparison with control group G1 and # indicates significance in comparison with diseased group G2, P < 0.05.
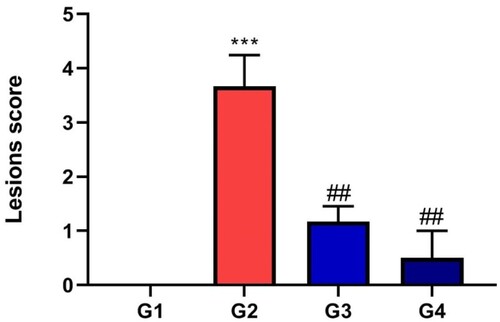
The adipose connective tissue of control animal showed normal small adipocytes separated with marked connective tissue septa. The diseased groups showed marked enlarged distend adipocytes with fat and most of them showed loss of their membrane. The diseased groups treated with bis-fused thiadiazinones 16 and 18 showed marked decrease in the fat cells of the adipocytes with decrease in their diameter. Quantitative estimation of the diameter of the fat cells showed marked increase in their diameter in adiposity group in comparison with control groups (P < 0.05). While the treated groups with bis-fused thiadiazinones 16 and 18 revealed marked decrease in the diameter of the fat cells in comparison with adiposity group (P < 0.05) (Figures and ).
Figure 7. White adipose connective tissue sections of different groups. (A) control group (white arrowhead indicates normal fat cells and black arrowhead indicates normal fibrous septa), (B) adiposity group (white arrowheads indicate large fat cells with disrupted cell membrane), (C) bis-fused thiadiazinones 16 Group (arrowhead indicates decrease the size of the fat cells) and (D) bis-fused thiadiazinones 18 group (arrowhead indicates marked decrease the size of the fat cells). H&E stain, bar = 50 µm.
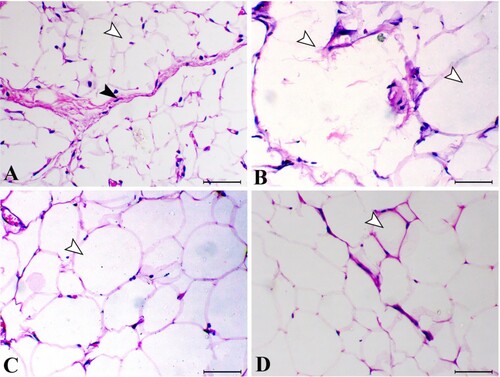
3. Experimental and characterization
3.1. Chemistry
On an electrothermal device, all melting points were calculated without being adjusted. GCMS-Q1000-EX Shimadzu spectrometers were conducted to record the mass spectra of the samples on the ionizing voltage at 70 eV. On the BRUKER 400 FT-NMR system spectrometer, 1H- and 13C-NMR spectra (500 MHz for 1H-NMR) were captured in DMSO solutions. On KBr discs, IR spectra were captured using a Shimadzu FT-IR 8201 PC spectrophotometer.
3.1.1. General procedure for synthesis of fused thiadiazinone derivatives 4a-m, 6, 8, 10, 12 and 14
A mixture of thione 1 (0.409 g, 1 mmol), the proper aromatic amines 2a-m or heterocyclic amines 5, 7, 9, 11 and 13 (1 mmol) and formaldehyde 3 (0.06 g 2 mmol) with one drop of hydrochloric acid under grinding technique at 25°C for 20 min. The products formed were crystalized from DMF or DMF\ EtOH (1:1) to give compounds 4a-m, 6, 8, 10, 12 and 14, respectively. The products’ spectrum data and physical attributes are listed below.
3.1.2. 3,7,8,9-Tetraphenyl-3,4,7,10-tetrahydropyrido[2’,3’:4,5]pyrimido[2,1-b][1,3,5]thiadiazin-6(2H)-one (4a)
Yield 79%; orange microcrystals; mp > 350°C; Anal. Calcd for C33H26N4OS (526.65): C, 75.26; H, 4.98; N, 10.64, Found: C, 75.11; H, 4.86; N, 10.53%; 1H-NMR: δ 4.11 (s, CH, 1H), 5.01 (s,, CH2 2H), 5.81 (s, CH2, 2H), 6.76–7.89 (m, Ar-H, 20H), 11.39 (br s, NH, 1H) ppm; 13C-NMR: δ 31.7(CH), 53.2, 69.8 (2CH2), 104.8, 12.2, 112.5, 115.6, 118.5, 120.9, 121.8, 122.1, 122.7, 122.9, 123.2, 124.9, 125.8, 128.9, 134.7, 137.1, 137.7, 143.7, 143.8, 150.6, 159.9 (Ar-C and C=N), 162.7(C=O) ppm; MS m/z (%): 526 (M+, 20), 495 (12), 391 (21), 313 (49), 265 (44), 106 (58), 71 (70), 57 (100); IR (KBr): v 1607 (C=N), 1702 (C=O), 2933, 3023 (CH), 3392 (NH) cm−1.
3.1.3. 7,8,9-Triphenyl-3-(p-tolyl)-3,4,7,10-tetrahydropyrido[2’,3’:4,5]pyrimido[2,1-b][1,3,5] thiadiazin-6(2H)-one (4b)
Yield 84%; beige microcrystals; mp 249–251°C; Anal. Calcd for C34H28N4OS (540.68): C, 75.53; H, 5.22; N, 10.36. Found: C, 75.41; H, 5.08; N, 10.23%; 1H-NMR: δ 2.22 (s, CH3, 3H), 4.20 (s, CH, 1H), 4.97 (s, CH2, 2H), 5.04 (s, CH2, 2H), 6.75–7.25 (m, Ar-H, 9H), 11.80 (s, NH, 1H) ppm; 13C-NMR: δ 21.0 (CH3), 31.9 (CH), 53.1, 70.2 (2CH2), 104.8, 112.3, 116.4, 116.9,120.9, 121.5, 125.0, 125.3, 129.5, 129.8, 133.2, 136.4, 136.8, 137.1, 140.7, 143.8, 146.5, 147.4, 147.5, 155.6, 158.8 (Ar-C and C=N), 163.0 (C=O) ppm; MS m/z (%): 540 (M+, 22), 529 (30), 398 (70), 322 (43), 271 (59), 206 (29), 119 (39), 91 (100), 77 (38); IR (KBr): v 1611 (C=N), 1694 (C=O), 2958, 3035 (CH), 3355 (NH), cm−1.
3.1.4. 7,8,9-triphenyl-3-(4-methoxyphenyl)-3,4,7,10-tetrahydropyrido[2’,3’:4,5]pyrimido[2,1-b][1,3,5] thiadiazin-6(2H)- one (4c)
Yield 77%; white microcrystals; mp 275–277°C; Anal. Calcd for C34H28N4O2S (556.68): C, 73.36; H, 5.07; N, 10.06. Found: C, 73.24; H, 4.93; N, 9.96%; 1H-NMR: δ 3.78 (s, OCH3, 3H), 4.18 (s, CH, 1H), 4.89 (s, CH2, 2H), 5.65 (s, CH2, 2H), 6.58–7.30 (m, Ar-H, 19H), 11.14 (br s, NH, 1H) ppm; 13C-NMR: δ 31.6 (CH), 53.3, 57.9 (OCH3), 70.0 (CH2), 104.3, 112.5, 116.6, 117.4, 121.7, 121.9, 124.6, 125.2, 128.4, 129.3, 132.5, 133.8, 135.9, 137.5, 140.0, 141.3, 143.6, 145.0, 147.2, 151.7, 156.3 (Ar-C and C=N), 163.2 (C=O) ppm; MS m/z (%): 556 (M+, 22), 474 (78), 370 (64), 313 (18), 230 (15), 170 (21), 88 (43), 57 (100); IR (KBr): v 1630 (C=N), 1703 (C=O), 2955, 3051 (CH), 3337 (NH) cm−1.
3.1.5. 3-(2-Ethoxyphenyl)-7,8,9-triphenyl-3,4,7,10-tetrahydropyrido[2’,3’:4,5]pyrimido[2,1-b][1,3,5]thiadiazin-6(2H)-one (4d)
Yield 88%; white microcrystals; mp 234–236°C; Anal. Calcd for C35H30N4O2S (570.70): C, 73.66; H, 5.30; N, 9.82. Found: C, 73.52; H, 5.19; N, 9.71%; 1H-NMR: δ 1.36 (t, CH3, 3H), 4.14 (s, CH, 1H), 4.25 (q, OCH2, 2H), 4.42 (s, CH2, 2H), 5.59 (s, CH2, 2H), 6.97–7.67 (m, Ar-H, 19 H), 11.30 (br s, NH, 1H) ppm; MS m/z (%): 556 (M+, 22), 552 (30), 525 (39), 474 (70), 370 (64), 313 (18), 230 (15), 170 (21), 88 (43), 55 (100); IR (KBr): v 1631 (C=N), 1705 (C=O), 2972, 3059 (CH), 3379 (NH) cm−1.
3.1.6. 3-(4-Chlorophenyl)-7,8,9-triphenyl-3,4,7,10-tetrahydropyrido[2’,3’:4,5]pyrimido[2,1-b][1,3,5]thiadiazin-6(2H)-one (4e)
Yield 81%; white microcrystals; mp 231–233°C; Anal. Calcd for C33H25ClN4OS (561.10): C, 70.64; H, 4.49; N, 9.99. Found C, 70.52; H, 4.37; N, 9.87%; 1H-NMR: δ 4.25 (s, CH, 1H), 4.63 (s, CH2, 2H), 4.99 (s, CH2, 2H), 7.07–7.68 (m, Ar-H, 19H), 11.33 (br s, NH, 1H) ppm; MS m/z (%): 561 (M+,15), 552 (27), 474 (23), 363 (37), 316 (46), 236 (32), 170 (32), 63 (71), 57 (100); IR (KBr): v 1622 (C=N), 1702 (C=O), 2962, 3066, (CH), 3365 (NH) cm−1.
3.1.7. 3-(3-Chlorophenyl)-7,8,9-triphenyl-3,4,7,10-tetrahydropyrido[2’,3’:4,5]pyrimido[2,1-b][1,3,5]thiadiazin-6(2H)-one (4f)
Yield 87%; beige microcrystals; mp 260–262°C; Anal. Calcd for C33H25ClN4OS (561.10): C, 70.64; H, 4.49; N, 9.99. Found: C, 70.52; H, 4.39; N, 9.88%; 1H-NMR: δ 4.20 (s, CH, 1H), 4.95 (s, CH2, 2H), 5.51 (s, CH2, 2H), 6.65–7.20 (m, Ar-H, 19H), 11.40 (br s, NH, 1H) ppm; MS m/z (%): 561 (M+, 15), 552 (27), 474 (23), 363 (37), 316 (46), 239 (32), 170 (32), 57 (100); IR (KBr): v 1602 (C=N), 1701 (C=O), 2961, 3066 (CH), 3363 (NH) cm−1.
3.1.8. 3-(4-Bromophenyl)-7,8,9-triphenyl-3,4,7,10-tetrahydropyrido[2’,3’:4,5]pyrimido[2,1-b][1,3,5]thiadiazin-6(2H)-one (4 g)
Yield 83%; white microcrystals; mp 240–242°C; Anal. Calcd for C33H25BrN4OS (605.55): C, 65.45; H, 4.16; N, 9.25. Found: C, 65.33; H, 4.06; N, 9.12%; 1H-NMR: δ 4.18 (s, CH, 1H), 4.98 (s, CH2, 2H), 5.38 (s, CH2, 2H), 6.89–7.63 (m, Ar-H, 19H), 11.58 (br s, NH, 1H) ppm; 13C-NMR: δ 32.2(CH), 53.9, 70.3 (2CH2), 104.9, 112.9, 114.8, 118.2, 118.8, 121.3, 122.0, 122.8, 124.6, 124.9, 125.3, 125.9, 127.2, 128.2, 131.6, 133.4, 137.0, 140.8, 143.1, 152.6, 159.3 (Ar-C and C=N), 163.4(C=O) ppm; MS m/z (%): 605 (M+, 15), 595 (31), 571 (28), 513 (29), 428 (19), 365 (98), 337 (82), 259 (32), 185 (64), 78 (100); IR (KBr): v 1635 (C=N), 1695 (C=O), 2971, 3064 (CH), 3352 (NH) cm−1.
3.1.9. 3-(4-Nitrophenyl)-7,8,9-triphenyl-3,4,7,10-tetrahydropyrido[2’,3’:4,5]pyrimido[2,1-b][1,3,5]thiadiazin-6(2H)-one (4 h)
Yield 89%; yellow microcrystals; mp 255–257°C; Anal. Calcd for C33H25N5O3S (571.65): C, 69.34; H, 4.41; N, 12.25. Found: C, 69.22; H, 4.31; N, 12.13%; 1H-NMR: δ 4.19 (s, CH, 1H), 4.91 (s, CH2, 2H), 5.56 (s, CH2, 2H), 6.73–7.73 (m, Ar-H, 19H), 11.39 (br s, NH, 1H) ppm; MS m/z (%): 571 (M+, 15), 560 (33), 524 (25), 437 (18), 325 (35), 283 (15), 150 (47), 77 (100); IR (KBr): v 1636 (C=N), 1701 (C=O), 2962, 3031 (CH), 3360 (NH) cm−1.
3.1.10. 3-(2,4-Dichlorophenyl)-7,8,9-triphenyl-3,4,7,10-tetrahydropyrido[2’,3’:4,5]pyrimido[2,1-b][1,3,5]thiadiazin-6(2H)-one (4i)
Yield 79%; white microcrystals; mp 230–232°C; Anal. Calcd for C33H24Cl2N4OS (594.10): C, 66.55; H, 4.06; N, 9.41. Found: C, 66.41; H, 3.95; N, 9.28%; 1H-NMR: δ 4.16 (s, CH,1H,), 5.15 (s, CH2, 2H), 5.77 (s, CH2, 2H), 6.81-8..09 (m, Ar-H, 18H), 11.29 (br s, NH, 1H) ppm; MS m/z (%): 594 (M+, 19), 552 (31), 538 (49), 466 (19), 367 (38), 313 (71), 213 (41), 172 (82), 161 (38), 84 (68), 57 (100); IR (KBr): v 1629 (C=N), 1694 (C=O), 2957, 3032 (CH), 3365 (NH) cm−1.
3.1.11. 3-(2,5-Dichlorophenyl)-7,8,9-triphenyl-3,4,7,10-tetrahydropyrido[2’,3’:4,5]pyrimido[2,1-b][1,3,5]thiadiazin-6(2H)-one (4j)
Yield 89%; white microcrystals; mp 139–141°C; Anal. Calcd for C33H24Cl2N4OS (594.10): C, 66.55; H, 4.06; N, 9.41. Found: C, 66.43; H, 3.98; N, 9.31%; 1H-NMR: δ 4.18 (s, CH, 1H), 4.91 (s, CH2, 2H), 5.75 (s, CH2, 2H), 6.80–7.69 (m, Ar-H, 18H), 11.61 (br s, NH, 1H) ppm; MS m/z (%): 594 (M+, 15), 580 (14), 509 (12), 467 (18), 359 (42), 272 (20), 230 (98), 202 (100), 164 (45), 57 (67); IR (KBr): v 1630 (C=N), 1697 (C=O), 2955, 3034 (CH), 3366 (NH) cm−1.
3.1.12. 3-(3-Chloro-2-methylphenyl)-7,8,9-triphenyl-3,4,7,10-tetrahydropyrido[2’,3’:4,5]pyrimid- [2,1-b][1,3,5]thiadiazin-6(2H)-one (4k)
Yield 75%; white microcrystals; mp = 245–247°C; Anal. Calcd for C34H27ClN4OS (575.12): C, 71.00; H, 4.73; N, 9.74. Found: C, 70.89; H, 4.63; N, 9.62%; 1H-NMR: δ 2.29 (s, CH3, 3H), 4.18 (s, CH, 1H), 5.17 (s, CH2, 2H), 5.87 (s, CH2, 2H), 6.82–7.85 (m, Ar-H, 18H), 11.51 (br s, NH, 1H) ppm; MS m/z (%): 575 (M+, 15), 565 (23), 551 (46), 474 (37), 367 (22), 313 (36), 230 (27), 152 (43), 77 (69), 55 (100); IR (KBr): v 1624 (C=N), 1697 (C=O), 2964, 3022 (CH), 3371 (NH) cm−1.
3.1.13. 7,8,9-Triphenyl-3-(2,4,6-trichlorophenyl)-3,4,7,10-tetrahydropyrido[2’,3’:4,5]pyrimido-[2,1-b][1,3,5]thiadiazin-6(2H)-one (4 l)
Yield 77%; white microcrystals; mp = 258–260°C; Anal. Calcd for C33H23Cl3N4OS (629.99): C, 62.91; H, 3.68; N, 8.89. Found: C, 62.80; H, 3.59; N, 8.79%; 1H-NMR: δ 4.16 (s, CH, 1H), 5.03 (s, CH2, 2H), 5.56 (s, CH2, 2H), 6.97–7.74 (m, Ar-H, 17H), 11.64 (br s, NH, 1H) ppm; MS m/z (%): 630 (M+, 15), 619 (25), 578 (21), 503 (19), 463 (15), 398 (53), 239 (43), 195 (97), 108 (47), 59 (100); IR (KBr): v 1629 (C=N), 1706 (C=O), 2965, 3071 (CH), 3353 (NH) cm−1.
3.1.14. 7,8,9-Triphenyl-3-(2,4,6-tribromophenyl)-3,4,7,10-tetrahydropyrido[2’,3’:4,5]pyrimido-[2,1-b][1,3,5]thiadiazin-6(2H)-one (4 m)
Yield 77%; white microcrystals; mp = 240–242°C; Anal. Calcd for C33H23Br3N4OS (763.34): C, 51.92; H, 3.04; N, 7.34. Found: C, 51.81; H, 2.90; N, 7.21%; 1H-NMR: δ 4.06 (s, CH, 1H), 4.75 (s, CH2, 2H), 5.37 (s, CH2, 2H), 6.96–7.64 (m, Ar-H, 17H), 11.48 (br s, NH, 1H) ppm; MS m/z (%): 764 (M+, 11), 740 (27), 690 (32), 565 (12), 398 (45), 329 (66), 230 (33), 171 (38), 71 (73), 57 (100); IR (KBr): v 1631 (C=N), 1704 (C=O), 2970, 3069 (CH), 3355 (NH) cm−1.
3.1.15. 7,8,9-Triphenyl-3-(thiazol-2-yl)-3,4,7,10-tetrahydropyrido[2’,3’:4,5]pyrimido[2,1-b] [1,3,5]thiadiazin-6(2H)-one (6)
Yield 89%; white microcrystals; mp = 268–270°C; Anal. Calcd for C30H23N5OS2 (533.13): C, 67.52; H, 4.34; N, 13.12. Found: C, 67.43; H, 4.20; N, 13.01%; 1H-NMR: δ 4.25 (s, CH, 1H), 4.69 (s, CH2, 2H), 5.39 (s, CH2, 2H), 6.99–7.66 (m, Ar-H, 17H), 11.12 (br s, NH, 1H) ppm; 13C-NMR: δ 31.7 (CH), 53.9, 70.0 (2CH2), 104.6, 113.5, 116.5, 117.9, 119.2, 124.6, 25.2, 125.8, 127.0, 129.6, 129.8, 133.0, 135.7, 136.2, 141.6, 142.0, 143.4, 146.4, 154.0, 159.5 (Ar-C and C=N), 164.4 (C=O) ppm; MS m/z (%): 533 (M+, 11), 480 (13), 409 (16), 313 (29), 264 (34), 167 (32), 105 (55), 77 (88), 57 (100); IR (KBr): v 1612 (C=N), 1693 (C=O), 2992, 3033 (CH), 3399 (NH) cm−1.
3.1.16. 3-(Benzo[d]thiazol-2-yl)-7,8,9-triphenyl-3,4,7,10-tetrahydropyrido[2’,3’:4,5]pyrimido[2,1-b][1,3,5]thiadiazin-6(2H)-one (8)
Yield 82%; white microcrystals; mp = 224–226°C; Anal. Calcd for C34H25N5OS2 (583.73): C, 69.96; H, 4.32; N, 12.00. Found: C, 69.87; H, 4.28; N, 11.86%; 1H-NMR: δ 4.20 (s, CH, 1H), 4.73 (s, CH2, 2H), 5.29 (s, CH2, 2H), 6.74–7.66 (m, Ar-H, 19H), 11.29 (br s, NH, 1H) ppm; MS m/z (%): 583 (M+, 12), 552 (61), 437 (29), 313 (27), 284 (31), 178 (35), 105 (100), 77(96); IR (KBr): v 1612 (C=N), 1703 (C=O), 2953, 3071 (CH), 3413 (NH) cm−1.
3.1.17. 3-(1,5-Dimethyl-3-oxo-2-phenyl-2,3-dihydro-1H-pyrazol-4-yl)-7,8,9-triphenyl-3,4,7,10-tetrahydropyrido[2’,3’:4,5]pyrimido[2,1-b][1,3,5]thiadiazin-6(2H)-one (10)
Yield 73%; white microcrystals; mp = 242–244°C; Anal. Calcd for C38H32N6O2S (636.23): C, 71.68; H, 5.07; N, 13.20. Found C, 71.57; H, 4.99; N, 13.08%; 1H-NMR: δ 2.27 (s, CH3, 3H), 2.52 (s, CH3, 3H), 4.14 (s, CH, 1H), 4.58 (s, CH2, 2H), 5.22 (s, CH2, 2H), 6.98–7.68 (m, Ar-H, 20H), 11.29 (br s, NH, 1H) ppm; MS m/z (%): 636 (M+, 2), 578 (33), 551 (45), 498 (56), 408 (43), 313 (17), 239 (13), 129 (21), 105 (99), 77 (90), 57 (100); IR (KBr): v 1619 (C=N), 1693, 1703 (2C=O), 2956, 3070 (CH), 3245 (NH) cm−1.
3.1.18. 7,8,9-Triphenyl-3-(pyridin-2-yl)-3,4,7,10-tetrahydropyrido[2’,3’:4,5]pyrimido[2,1-b][1,3,5]thiadiazin-6(2H)-one (12)
Yield 86%; white microcrystals; mp = 235–237°C; Anal. Calcd for C32H25N5OS (527.64): C, 72.84; H, 4.78; N, 13.27. Found C, 72.77; H, 4.71; N, 13.19%; 1H-NMR: δ 4.16 (s, CH,1H), 5.18 (s, CH2, 2H), 5.87 (s, CH2, 2H), 6.93–8.35 (m, Ar-H, 19H), 11.10 (br s, NH, 1H) ppm; 13C-NMR: δ 31.5 (CH), 53.2, 70.2 (2CH2), 104.7, 108.1, 114.7, 116.2, 119.4, 123.7, 125.9, 126.0, 128.2, 129.1, 129.7, 130.4, 134.5, 135.2, 136.0, 136.6, 140.2, 141.2, 143.8, 145.6, 151.3, 157.9 (Ar-C and C=N), 163.5 (C=O) ppm; MS m/z (%): 527 (M+, 3), 479 (14), 404 (26), 372 (29), 294 (36), 211 (26), 105 (100), 77 (81); IR (KBr): v 1628 (C=N), 1703 (C=O), 2960, 3067 (CH), 3446 (NH) cm−1.
3.1.19. 7,8,9-Triphenyl-3-(pyrimidin-2-yl)-3,4,7,10-tetrahydropyrido[2’,3’:4,5]pyrimido[2,1-b][1,3,5]thiadiazin-6(2H)-one (14)
Yield 82%; white microcrystals; mp = 242–244°C; Anal. Calcd for C31H24N6OS (528.63): C, 70.43; H, 4.58; N, 15.90. Found C, 70.31; H, 4.47; N, 15.81%; 1H-NMR: δ 4.41 (s, CH, 1H), 4.52 (s, CH2, 2H), 4.62 (s, CH2, 2H), 6.75–7.66 (m, Ar-H, 18H), 12.27 (br s, NH, 1H) ppm; MS m/z (%): 528 (M+, 3), 495 (17), 443 (21), 320 (31), 243 (38), 156 (25), 105 (100), 77 (87); IR (KBr): v 1608 (C=N), 1702 (C=O), 2965, 3069 (CH), 3365 (NH) cm−1.
3.1.20 Synthesis of bis-fused thiadiazinenones 16 and 18
A mixture of thione 1 (0.818 g, 2 mmol), the p-phenylene diamine 15 (0.108 g, 1 mmol) or p,p′-diaminodiphenylmethane 17 (0.198 g, 1 mmol) and formaldehyde 3 (0.12 g 4 mmol) with one drop of HCl under grinding technique at 25°C for 30 min. The formed products were crystalized from DMF to give the respective bis-fused thiadiazinones 16 and 18.
3.1.21. 3,3’-(1,4-Phenylene)bis(7,8,9-triphenyl-3,4,7,10-tetrahydropyrido[2’,3’:4,5]-pyrimido[2,1-b][1,3,5]thiadiazin-6(2H)-one) (16)
Yield 71%; pale yellow microcrystals; mp > 300°C; Anal. Calcd for C60H46N8O2S2 (975.19): C, 73.90; H, 4.75; N, 11.49. Found C, 73.82; H, 4.61; N, 11.36%; 1H-NMR: δ 4.60 (s, 2CH, 2H), 4.86 (s, 2CH2, 2 × 2H,), 4.96 (s, 2CH2, 2 × 2H), 6.97–7.60 (m, Ar-H, 2 × 17H), 12.35 (br s, 2NH, 2H) ppm; MS m/z (%): 975 (M+, 63), 949 (16), 856 (13), 722 (20), 624 (24), 563 (19), 446 (14), 368 (29), 252 (39), 105 (64), 57 (100); IR (KBr): v 1600 (C=N), 1707 (C=O), 2955, 3062 (CH), 3357 (NH) cm−1.
3.1.22. 3,3’-(Methylene-bis(4,1-phenylene))bis(7,8,9-triphenyl-3,4,7,10-tetrahydropyrido-[2’,3’:4,5] pyrimido[2,1-b][1,3,5]thiadiazin-6(2H)-one) (18)
Yield 72%; brown microcrystals; mp > 300°C; Anal. Calcd for C67H52N8O2S2 (1064.37): C, 75.54; H, 4.92; N, 10.52; Found C, 75.44; H, 4.81; N, 10.41%; 1H-NMR: δ 2.64 (s, CH2, 2H), 4.41 (s, 2CH, 2H), 4.60 (s, 2CH2, 2 × 2H), 4.64 (s, 2CH2, 2 × 2H), 6.16–7.95 (m, Ar-H, 2 × 19H), 12.32 (br s, 2NH, 2H) ppm; MS m/z (%): 1064 (M+, 63), 999 (18), 907 (22), 845 (16), 773 (31), 640 (24), 387 (29), 284 (16), 180 (26), 105 (69), 77 (100); IR (KBr): v 1629 (C=N), 1725 (C=O), 2952, 3060 (CH), 3260 (NH) cm−1.
3.2. Molecular docking
In the present study, fat and obesity protein (FTO protein) is selected as therapeutic target for identifying potential inhibitors for obesity. All newly synthesized compounds 4–18 are subjected to molecular docking by calculating the minimum energy to inhibit the target protein. The protein structure (PDB ID: 3LFM) is downloaded from RCSB protein databank [Citation59]. Ligand molecules are sketched using ChemDraw ultra 8.0 (in cdx format) and converted using Open Babel 2.4.1 (SDF format) [Citation60]. All the compound structures and the target are subjected to energy minimization using universal force field [Citation61] in Open Babel and CHARMM Force Field [Citation62] in Discovery Studio, respectively. PyRx-Virtual screening tool [Citation63] is used to perform the molecular docking. Electrostatic potential map of PM6 semiempirical method was performed using Gaussian 03. Finally, the computational study for prediction of ADMET and physicochemical properties of the best docked compounds are calculated through AdmetSAR (http://lmmd.ecust.edu.cn/admetsar2/) and Mol inspiration (https://www.molinspiration.com) softwares.
3.3. Biological study
3.3.1. Experimental animal study
White male albino rats (Wistar rat) (Rattus norvegicus), with age 10 weeks and about 120–160 g body weight. The used animals were collected from Faculty of Veterinary Medicine, Cairo University, Egypt.
Adult rats were kept in Lab Animal Experimental Facility, University of South Valley, under acclimatization for 1 week before experimentation. The animals were (3) rats per cage, kept at a temperature of 23 ± 2°C, with acceptable ventilation and a relative humidity of 50 ± 5%. The rats were fed either a regular mouse pellet diet or a high-fat diet (HFD), with consistent light/dark cycles lasting 12 h each [Citation64]. They were allowed to drink ad libitum during the whole course of the experiment.
Animals were divided into four groups, G1 (control group), G2 (HFD group) injected with 35 mg/kg STZ, IP and supplemented with HFD composed as follow: Powdered NPD (365 mg), Lard (310 mg), Vitamin and mineral mix (60 mg), Casein (250 mg), Cholesterol (10 mg), dl-Methionine (3 mg), Yeast powder (1 mg) and Sodium chloride (1 mg) for 24 weeks. The two further groups were subjected to both STZ and HFD and treated with bis-fused thiadiazinones 16 (G3) and 18 (G4). Our protocol complies with Faculty of Science, South Valley University for the Care and Use of Laboratory Animals.
3.3.2. Histopathology technique
Liver and adipose samples were collected and fixed in 10% neutral buffered formalin. After dehydration and clearance, the tissues were fixed in paraffin and sectioned in 5 µm thickness. The serial sections were subjected to staining with haematoxylin and eosin [Citation65]. Scoring of hepatic steatosis was done according to [Citation66], wherein the amount of fat contained within each hepatocyte was graded: grade 0 (healthy, <5%), grade 1 (mild, 5–33%), grade 2 (moderate, 34–66%) and grade 3 (severe, >66%). The diameter of fat cell was measured using ImageJ analysis software, NIH, USA and expressed as mean ± SD.
3.3.3. Statistical analysis
All data were expressed as mean ± standard deviation. ANOVA test (one-way analysis of variance) was carried out with Tukey’s post hoc analysis using GraphPad Prism 5 (GraphPad Software for Windows Inc., San Diego, CA, United States) and p value < 0.05 was considered to be statistically significant.
4. Conclusion
During the current investigation, we synthesized a new fused thiadiazineone derivatives 4a-m, 6, 8, 10, 12 and 14 via grinding technology starting from the corresponding thione 1 and a variety of aromatic amines or heterocyclic amines and formaldehyde, and their structural and spectral data were elucidated. Furthermore, the docking approach was carried out to investigate the mode of interactions of the compounds 4–18 against FTO protein. The ligand molecules are nicely docked to the target FTO, with docking affinities (ΔGbind); −11.6 to −8.0 kcal/mol. Finally, the biological activity of the tested compounds were in line with in silico results, wherein they exhibited that bis-fused thiadiazinones 16 and 18 revealed marked amelioration of adiposity in the HFD model especially in hepatic and adipose tissues; making them as possible anti-obesity agents.
Disclosure statement
No potential conflict of interest was reported by the author(s).
References
- Costa G, Gidaro MC, Vullo D, et al. Active components of essential oils as anti-obesity potential drugs investigated by in silico techniques. JAgric Food Chem. 2016;64:5295–5300.
- Sumaryada T, Simamora REM, Ambarsari L. Docking evaluation of catechin and its derivatives on fat mass and obesity-associated (FTO) protein for anti-obesity agent. J Appl Pharm Sci. 2018;8:63–68.
- Gandhi SP, Lokhande KB, Swamy VK, et al. Computational data of phytoconstituents from Hibiscus rosa-sinensis on various anti-obesity targets. Data Br. 2019;24:103994.
- Gomha SM, Riyadh SM. Cellulose sulfuric acid as an eco-friendly catalyst for novel one-pot synthesis of pyrido[2,3-d][1,2,4]triazolo[4,3-a]pyrimidin-5-ones. J Braz Chem Soc 2015;26:916–923.
- Qurioga J, Hormaza A, Insuasty B, et al. Synthesis of pyrimido[4,5-b]quinolines in the reaction of 6-aminopyrimidines with dimedone and benzaldehydes. J Heterocycl Chem. 1998;35:231–233.
- Gomha SM, Abdallah MA, Mourad MA, et al. Application of Mannich and Michael reactions in synthesis of pyridopyrimido[2,1-b][1,3,5]thiadiazinones and pyridopyrimido[2,1-b][1,3]thiazinones as anticancer agents. Heterocycles. 2016;92:688–699.
- Nasr MN, Gineinah MM. Pyrido[2,3-d]pyrimidines and pyrimido[5′,4′:5,6]pyrido[2, 3-d]pyrimidines as new antiviral agents: synthesis and biological activity. Arch Pharm. 2002;335:289–295.
- Taylor EC, Palmer DC, George TJ, et al. Synthesis and biological activity of L-5-deazafolic acid and L-deazaaminopterin: synthetic strategies to 5-deazapteridines. J Org Chem. 1983;48:4852–4860.
- Nam G, Yoon CM, Kim E, et al. Syntheses and evaluation of pyrido[2,3-d]pyrimidine-2,4-diones as PDE IV inhibitors. Bioorg Med Chem Lett. 2011;5:611–614.
- Pai SB, Liu SH, Zhu ZL, et al. Inhibition of hepatitis B virus by a novel L-nucleoside, 2'-fluoro-5-methyl-beta-L-arabinofuranosyl uracil. Antimicrob Agents Chemother. 1996;40:380–386.
- Huron DR, Corre ME, Kraker AJ, et al. A novel pyridopyrimidine inhibitor of Abl kinase is a picomolar inhibitor of Bcr-abl-driven K562 cells and Is effective against STI571-resistant Bcr-abl mutants. Clinical Cancer Res. 2003;9:1267–1273.
- Smejkalova H, Erb TJ, Fuchs G. Methanol assimilation in methylobacterium extorquens AM1: demonstration of all enzymes and their regulation. PLoS ONE. 2010;5:e13001.
- Erb TJ, Berg IA, Brecht V, et al. Synthesis of C5-dicarboxylic acids from C2-units involving crotonyl-CoA carboxylase/reductase: the ethylmalonyl-CoA pathway. Proc Natl Acad Sci USA. 2007;104:10631–10636.
- Khomyakova M, Bukmez O, Thomas LK, et al. A methylaspartate cycle in haloarchaea. Science 2011;331:334–337.
- Gangjee A, Vasudevan A, Queener SF, et al. 2,4-Diamino-5-deaza-6-substituted pyrido[2,3-d]pyrimidine antifolates as potent and selective nonclassical inhibitors of dihydrofolate reductases. J Med Chem. 1996;39:1438–1446.
- Gangjee A, Adair OO, Pagley M, et al. N9-substituted 2,4-diaminoquinazolines: synthesis and biological evaluation of lipophilic inhibitors of pneumocystis carinii and toxoplasma gondii dihydrofolate reductase. J Med Chem 2008;51:6195–6200.
- Chan DCM, Rosowsky A. Synthesis of the lipophilic antifolate piritrexim via a palladium(0)-catalyzed cross-coupling reaction. J Org Chem. 2005;70:1364–1368.
- Tong L. Structure and function of biotin-dependent carboxylases. Cell Mol Life Sci. 2013;5:863–891.
- Shivaiah K-K, Upton B, Nikolau BJ. Kinetic, structural, and mutational analysis of Acyl-CoA carboxylase from thermobifida fusca YX. Front Mol Biosci. 2021;7:615614.
- Subramaniapillai SG. Mannich reaction: a versatile and convenient approach to bioactive skeletons. J Chem Sci 2013;125:467–482.
- Roman G. Mannich bases in medicinal chemistry and drug design. Eur J Med Chem. 2015;89:743–816.
- Mammadbayli EH, Hajiyeva GE, Ibrahimli SI, et al. Synthesis and antimicrobial activity of norbornene-containing Mannich bases. Russ J Appl Chem. 2019;92:1161–1169.
- Afsah EMM, Kandil ED, Abdelmageed SM, et al. Synthesis of novel Mannich bases and hybrid Mannich bases related to isoindolin-1,3-dione. J Heterocycl Chem. 2020;57:346–354.
- Banerjee D, Yogeeswari P, Bhat P, et al. Novel isatinyl thiosemicarbazones derivatives as potential molecule to combat HIV-TB co-infection. Eur J Med Chem. 2011;46:106–121.
- Roman G. Anticancer activity of Mannich bases: a review of recent literature. ChemMedChem. 2022;17:e202200258.
- Liu C, Zhou L, Jiang D, et al. Multicomponent reactions of Aldo-X bifunctional reagent α-oxoketene dithioacetals and indoles or amines: divergent synthesis of dihydrocoumarins, quinolines, furans, and pyrroles. Asian J Org Chem. 2016;5:367–372.
- Cioc RC, Ruijter E, Orru RVA. Multicomponent reactions: advanced tools for sustainable organic synthesis. Green Chem. 2014;16:2958–2975.
- Abu-Melha S, Muhammad ZA, Abouzid AS, et al. Multicomponent synthesis, DFT calculations and molecular docking studies of novel thiazolyl-pyridazinones as potential antimicrobial agents against antibiotic-resistant bacteria. J Mol Str. 2021;1234:130180.
- Abu-Melha S, Gomha SM, Abouzied AS, et al. Microwave-assisted one pot three-component synthesis of novel bioactive thiazolyl-pyridazinediones as potential antimicrobial agents against antibiotic-resistant bacteria. Molecules. 2021;26:4260.
- Alshabanah LA, Al-Mutabagani LA, Gomha SM, et al. Three-component synthesis of some new coumarin derivatives as anti-cancer agents. Front Chem. 2022;9:762248S.
- Gomha SM, Kheder NA, Abdelhamid AO, et al. One pot single step synthesis and biological evaluation of some novel bis(1,3,4-thiadiazole) derivatives as potential cytotoxic agents. Molecules. 2016;21:1532.
- Gomha SM, Edrees MM, Faty RAM, et al. Microwave-assisted one pot three-component synthesis of some novel pyrazole scaffolds as potent anticancer agents. Chem Central J. 2017;11:37.
- Gu Y. Multicomponent reactions in unconventional solvents: state of the art. Green Chem. 2012;14:2091–2128.
- Mohamed MAA, Abd Allah OA, Bekhit AA, et al. Synthesis and antidiabetic activity of novel triazole derivatives containing amino acids. J Heterocycl Chem. 2020;57:2365–2378.
- Saadati MR, Maleki B, Tayebee R, et al. 6-methylguanamine-supported cofe2o4: an efficient catalyst for one-pot three-component synthesis of Isoxazol-5(4H)-One derivatives. Polycycl Aromat Compd. 2020;42:885–896.
- Al-Shaal MG, Hausoul PJC, Palkovits R. Efficient, solvent-free hydrogenation of α-angelica lactone catalysed by Ru/C at atmospheric pressure and room temperature. Chem Commun. 2014;50:10206–10209.
- Sheldon RA. Selective catalytic synthesis of fine chemicals: opportunities and trends. J Mol Catal A Chem. 1996;107:75–83.
- Acharyulu PVR, Dubey PK, Prasada Reddy PVV, et al. Synthesis of new 4(3H)-quinazolinone derivatives under solvent-free conditions using PEG-400. Arkivoc. 2008;11:104–111.
- Sayed AR, Gomha SM, Abdelrazek FM, et al. Design, efficient synthesis and molecular docking of some novel thiazolyl-pyrazole derivatives as anticancer agents. BMC Chem. 2019;13:1–13.
- Metwally NH, Abdelrazek FM, Eldaly SM. Synthesis, molecular docking, and biological evaluation of some novel bis-heterocyclic compounds based N,N′-([1,1′-biphenyl]-4,4′-diyl)bis(2-cyanoacetamide) as potential anticancer agents. J Heterocycl Chem. 2018;55:2668–2682.
- Gomha SM, Muhammad ZA, Abdel-aziz HM, et al. Green synthesis, molecular docking and anticancer activity of novel 1,4-dihydropyridine-3,5-dicarbohydrazones under grind-stone chemistry. Green Chem Lett Rev. 2020;13:6–17.
- Gomha SM, Abdelrazek FM, Abdelrahman AH, et al. Synthesis of some novel thiazole, thiadiazole and 1,4-phenylene-bis-thiazole derivatives as potent antitumor agents. Heterocycles. 2016;92:954–967.
- Gomha SM, Riyadh SM. Synthesis of triazolo[4,3-b][1,2,4,5]tetrazines and triazolo[3,4-b][1,3,4]thiadiazines using chitosan as ecofriendly catalyst under microwave irradiation. Arkivoc. 2009;xi:58–68.
- Gomha SM, Muhammad ZA, Abdel-aziz MR, et al. One pot synthesis of new thiadiazolyl-pyridines as anticancer and antioxidant agents. J Heterocycl Chem. 2018;55:530–536.
- Abbas EMH, Gomha SM, Farghaly TA. Multicomponent reactions for synthesis of bioactive polyheterocyclic ring systems under controlled microwave irradiation. Arab J Chem. 2014;7:623–629.
- Gomha SM, Riyadh SM. Multicomponent synthesis of novel penta-heterocyclic ring systems incorporating benzopyranopyridines scaffold. Synthesis. 2014;46:258–262.
- Abdelrazek FM, Gomha SM, Abdel-aziz HM, et al. Efficient synthesis and in silico study of some novel pyrido[2,3-d][1,2,4]triazolo[4,3-a]pyrimidine derivatives. J Heterocycl Chem. 2020;57:1759–1769.
- Abdelrazek FM, Gomha SM, Farghaly MS, et al. One-pot, three-component synthesis of pyrido[2,3-d]pyrimidinones using aluminate sulfonic acid nanocatalyst under grinding technique. Polycycl Arom Comp. 2021;41:1472–1482.
- Abdelmonsef AH, Mosallam AM. Synthesis, in vitro biological evaluation and in silico docking studies of new quinazolin-2,4-dione analogues as possible anticarcinoma agents. J Heterocycl Chem. 2020;57:1637–1654.
- El-Naggar M, Mohamed ME, Mosallam AM, et al. Synthesis, characterization, antibacterial activity, and computer-aided design of novel quinazolin-2,4-dione derivatives as potential inhibitors against vibrio cholerae. Evol Bioinform. 2020;16:1–13.
- Rashdan HRM, Abdelmonsef AH. In silico study to identify novel potential thiadiazole-based molecules as anti-Covid-19 candidates by hierarchical virtual screening and molecular dynamics simulations. Struct Chem. 2022;33(5):1727–1739.
- Rashdan HRM, El-Naggar M, Abdelmonsef AH. Synthesis, molecular docking studies and in silico admet screening of new heterocycles linked thiazole conjugates as potent anti-hepatic cancer agents. Molecules. 2021;26:1–17.
- Abdelmonsef AH, et al. A search for antiinflammatory therapies: synthesis, in silico investigation of the mode of action, and in vitro analyses of new quinazolin-2,4-dione derivatives targeting phosphodiesterase-4 enzyme. J Heterocycl Chem. 2021;1:1–19.
- Abdelmonsef AH. Computer-aided identification of lung cancer inhibitors through homology modeling and virtual screening. Egypt J Med Hum Genet. 2019;20(1):1–14.
- Noser AA, Abdelmonsef AH, El-naggar M, et al. New amino acid Schiff bases as anticancer agents via potential mitochondrial complex I-associated hexokinase inhibition and targeting AMP-protein kinases / mTOR signaling pathway. Molecules. 2021;26:1–28.
- Abdelmonsef AH, Mohamed ME, El-Naggar M, et al. Novel quinazolin-2,4-dione hybrid molecules as possible inhibitors against malaria: synthesis and in silico molecular docking studies. Front Mol Biosci. 2020;7:1–19.
- Noser AA, Shehadi IA, Abdelmonsef AH, et al. Newly synthesized pyrazolinone chalcones as anticancer agents via inhibiting the PI3K/Akt/ERK1/2 signaling pathway. ACS Omega. 2022;7:25265–25277.
- Hussein HM, El-Adasy A-BA, El-Saghier AM, et al. Synthesis, characterization, in silico molecular docking, and antibacterial activities of some new nitrogen-heterocyclic analogues based on a p- phenolic unit. RSC Adv. 2022;12:12607–12621.
- Berman HM. The protein data bank. Nucleic Acids Res. 2000;28:235–242.
- O’Boyle NM, Banck M, James CA, et al. Open Babel: an open chemical toolbox. J Cheminform. 2011;3:33.
- Rappé K, Casewit CJ, Colwell KS, et al. UFF, a full periodic table force field for molecular mechanics and molecular dynamics simulations. J Am Chem Soc. 1992;114:10024.
- Brooks R, Brooks CL, Mackerell AD, et al. CHARMM: molecular dynamics simulation package. J Comput Chem 2009;30:1545–1614.
- Dallakyan S, Olson AJ. Small-molecule library screening by docking with PyRx. Chem Biol. 2015;1263:243–250.
- Srinivasan K, Patole PS, Kaul CL, et al. Reversal glucose intolerance by pioglitazone in high fat diet-fed rats. Methods Find Exp Clin Pharmacol. 2004;26:327.
- Bancroft JD, Layton C. The haemtoxylins and eosin. Bancroft’s theory and practice of histological techniques, expert consult: online and print, 7: Bancroft’s theory and practice of histological techniques 2013, p. 173.
- Nassir F, Rector RS, Hammoud GM, et al. Pathogenesis and prevention of hepatic steatosis. Gastroenterol Hepatol. 2015;11:167.