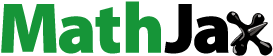
Abstract
Breast cancer is a leading cause of cancer-related deaths in women, and researchers are seeking more effective treatments. Nanotechnology offers a promising approach by creating nanocarriers to reduce the side effects of cancer drugs. This study synthesized AgNPs-5FU nanoparticles by binding the anticancer drug 5-fluorouracil (5FU) to a silver nanocarrier, significantly increasing 5FU's cytotoxicity. Characterization using DLS, SEM, UV-Vis, and FTIR confirmed the nanoparticles' properties, with a size of 18–28 nm and a PDI of 0.598. The release of 5FU from AgNPs-5FU was about 70%, demonstrating sustained and controlled release. Cytotoxicity results showed that AgNPs-5FU had an IC50 value of 23.006 in MCF-7 cells and 10.41 in 4T1 cells, making it 2.14 and 4.64 times more effective than free 5FU, respectively. Low cytotoxicity was observed in HUVEC cells. This study indicates that AgNPs-5FU has significantly higher potential activity against cancer cells compared to free 5FU.
1. Introduction
Cancer is the world’s leading cause of death, and among different types of cancer, breast cancer is the second most common cause of death in women after lung cancer. In addition, breast cancer constitutes 30 per cent of cancers in women and is the most common type of cancer in women. Breast cancer is responsible for 15 per cent of cancer-related deaths in women [Citation1–3]. Breast cancer is a hormonal and heterogeneous disease with a number of clearly defined morphological subtypes [Citation4]. Despite various treatment options such as surgery, radiotherapy and chemotherapy, the survival rate for locally advanced and metastatic breast cancer has not increased significantly [Citation5]. All of these factors necessitate the development of new models for breast cancer treatment.
The medication 5-Fluorouracil (5FU) was found in 1956 and entered biological application in 1962. It is a cytotoxic drug which has for years been used in the treatment of various neoplastic diseases: cancers of the lung, breast, skin, liver, colon, bladder, pancreas, head and chest [Citation6–8]. It is a primidine analologue and metabolised through the same metabolic pathways as uracil. It therefore inhibits thymidylate synthase, reduces thymidine nucleotide synthesis and induces DNA damage [Citation9]. Many known anti-cancer drugs such as 5FU show poor pharmacokinetics, limited bioavailability and high toxicity [Citation10]. Although 5FU is water soluble, there are several barriers such as its lack of cancer selectivity, poor absorption by oral administration, dose dependent toxicity, and high drug resistance. Due to its limited biological utility, positive response rates for various types of cancer are very low. Therefore, 5FU can cause serious toxicity in gastrointestinal, hematological, neural, cardiac and dermatological areas [Citation11–13].
Studies are ongoing in various fields to reduce the side effects of anticancer drugs and nanotechnology is one of these fields. The rise of nanotechnology has had a tremendous impact on medicine with nanoparticles-based drug delivery system, enabling selective and targeted treatment of tumour cells. Realisation of improved targeting potential, and controlled drug release for 5FU may be possible by nanocarrier systems. Nanodrug delivery systems can increase drug concentration in targeted tumour cells while sparing healthy cells. The targeting potential of the nanodrugs also depends on the size and surface characteristics. Thus, it is possible to increase circulation time and targeting efficiency of drugs with these systems [Citation12]. For all these reasons, it is necessary to investigate the therapeutic potential of 5FU in drug delivery systems. Researchers continue developing various nanocarrier systems to enhance the cytotoxicity of different agents on cancer cells. Thus, better results may be obtained by investigating the effect of 5FU on potential drug carrier systems to obtain a better therapeutic effect [Citation14–17].
Silver nanoparticles (AgNPs) are widely applied, especially in medical fields especially in treatment, diagnosis, drug delivery and, wound dressings [Citation18]. Moreover, AgNPs have multifunctional biological properties such as antibacterial, antiviral, antiangiogenic, antifungal, anti-inflammatory, and anti-cancer effects [Citation19]. However, the systemic toxicity of silver nanoparticles is the topic of several investigations. The toxicity of AgNPs depends on various factors such as size, shape, concentration, surface charge and functionalization [Citation20]. Different studies have been conducted on this subject in the literature. There was no variations in the micronucleated polychromatic erythrocytes and oral toxicity of different dosages of AgNPs in rats [Citation21]. According to a different study by Lee et al. subcutaneous injection of AuNP, AgNP or their combination did not cause any noticeable systemic toxicity [Citation22]. Inorganic nanoparticles can also be targeted using a variety of markers for cancer cell receptors, including human epidermal growth factor receptor-2, folate, CD44, and gastrin-releasing peptide [Citation23]. The efficacy of silver nanoparticles can be increased by activating them with infrared light, radio waves, and a magnetic field [Citation24, Citation25]. Silver nanoparticles have recently been used with composite materials such alumino silicate minerals, kaolinite, and halloysite to stabilize and reduce their systemic toxicity [Citation26].
AgNPs were used in our study due to their superior physicochemical and biological properties. These properties maximise their possible use in many fields while reducing their disadvantages to humans and the environment [Citation27]. Among the different types of anti-cancer treatment, nanoparticle-based treatment has received increasing attention in breast cancer chemotherapy because it allows better tissue penetration than free drugs and increases the entry of cancer drugs by affecting the permeability of membranes [Citation8, Citation28, Citation29].
In this study, we synthesized 5FU-loaded silver nanoparticles (AgNPs-5FU) and investigated the anticancer effects of 5FU and AgNPs-5FU on three breast cancer cell lines (MCF – 7, MDA-MB – 231, 4T1) and normal HUVEC cells. The novelty of this study is that we obtained smaller AgNPs-5FU than in previous studies, and the effects of these AgNPs-5FU on different breast cancer cells were demonstrated in the same study. Furthermore, this study is unique in that it provides a larger data set than previous studies by comparing 5FU-loaded AgNPs, free 5FU and AgNPs components on various breast cancer cell lines. The findings have the potential to aid in the development of more effective breast cancer therapies.
2. Materials and methods
MCF-7, MDA-MB-231, 4T1 and HUVEC cell lines were purchased from ATCC (USA). Silver nitrate Ag (NO3), trisodium citrate, NaOH, (TSC) and, sodium borohydride (NaBH4) were obtained from Sigma (USA). MTT kit, Dimethylsulfoxide, and Dulbecco’s Modified Eagle’s Medium (DMEM) and, trypsin were obtained from Sigma (USA). Streptomycin, penicillin and FBS were purchased from Gibco (UK), PBS from Sigma (USA), and 5FU from Glentham (United Kingdom).
2.1. Silver nanoparticles synthesis
Stock solutions of 1 × 10−3 M Ag(NO3), 2 × 10−3 M NaBH4 and 4.28 × 10−3 M trisodium citrate were prepared. 24 mL of NaBH4 and 24 mL of trisodium citrate stock solution prepared in a beaker were mixed with a magnetic stirrer at 60°C. It was added dropwise from Ag(NO3) stock solution. The temperature of the mixture was raised to 90°C. The AgNPs solution produced was stored in a dark environment to be loaded with 5FU [Citation30]. 5FU stock solution was prepared as 1 mg/ mL, and 1 each mL 5FU and AgNPs solution were stirred for about half an hour in the dark.
2.2. Characterization of silver nanoparticles
Characterization of AgNPs and AgNPs-5FU was studied by DLS, SEM and, FTIR analysis.
2.3. FTIR analysis of AgNPs and AgNPs-5FU
A Perkin Elmer Spectrum 400 spectrometer with 4 cm−1 resolution and 10 scans per spectrum was used to examine AgNPs and AgNPs-5FU. Then, the samples of liquid were put onto the FTIR crystal and allowed to dry. After the samples had dried, FTIR spectra were collected. After evaluating the samples, the sample section of the FTIR instrument was cleaned with a solution of alcohol and water, and another sample was analyzed.
2.4. DLS analysis of AgNPs and AgNPs-5FU
The laser scattering method was used to determine the particle size of the materials. The results in this work were taken at 25 °C using a Malvern Zetasizer (Malvern Instruments Ltd., Malvern, UK) equipped with a high-concentration zeta potential cell. After dilute using distilled water, the size of the NPs was evaluated. Light dispersion was carried out at 25°C and at a 90° angle.
2.5. SEM analysis of AgNPs and AgNPs-5FU
An EVO40-LEO electronically controlled digital electron scanning microscope was used to examine the surface characteristics of the particles (SEM). The samples were put onto surface area and dried. Liquid samples were placed onto copper conductor electrodes prior to examination. After drying for 24 hours, SEM analyses were performed following the Au/Pd coating.
2.6. AgNPs-5FU drug diffusion
The release of 5FU drug from AgNPs was examined in vitro at 37 ± 0.5 °C in buffer solutions (pH 7.4) using a dialysis tube cellulose barrier (Sigma Aldrich Company, average flat width 10 mm) (0.4 in.) and PBS buffer solution. Diffusion parameters were used for AgNPs-5FU in the evaluation of 5FU release. Drug absorption 5FU was measured at 290 nm via UV-VIS spectrophotometry in PBS. Diffusion parameters were calculated following our previous study [Citation30]. Diffusion parameters were examined following Fick’s law.
2.7. Cell culture
In this paper, MCF-7 (breast cancer), MDA-MB231 (triple-negative breast cancer), 4T1 (murine breast cancer) cells and normal HUVEC (Human umbilical vein endothelial cells) cell lines were used. The cells were cultured in DMEM containing 10% FBS, 100 units/mL penicillin and 100 μg/mL of streptomycin in a humidified incubator with an atmosphere of 5% CO2 at 37°C. The cytotoxic effects of AgNPs, AgNPs-5FU, and 5-FU on cancerous (MCF-7, MDA-MB231, 4T1) and non-cancerous (HUVEC) cells were determined by MTT assay for 72 h [Citation31].
2.8. Cytotoxic effect
The cellular cytotoxicity of 5FU and AgNPs-5FU was investigated by MTT assay (3-(4,5-dimethylthiazol-2-yl)-2,5-diphenyltetrazolium bromide). This procedure has been previously reported [Citation12]. The concentrations of 5FU and AgNPs-5FU that were used on malignant (MCF-7, MDA-MB-231, 4T1) and normal (HUVEC) cells separately were 3.125, 6.25, 12.5, 25, and 50 µg/mL.
Experiments were repeated at least three times.
3. Results and discussion
Breast cancer is a very important cause of cancer-related deaths in women. The aim of treatment is to minimize or eliminate relapse, resistance and toxic effects while providing patients with maximum benefit from the treatment, and at the same time ensuring that patients have a good quality of life [Citation32]. One of the biggest challenges in its treatment is the heterogeneous nature of breast cancer, which plays an important role is of importance the selection of therapeutic approaches. Clinical response varies widely among different breast cancer subtypes due to its heterogeneous nature [Citation33].
In this paper, the size distribution of particles of AgNPs-5FU was measured using a Dynamic Light Scattering (DLS). According to the DLS results, AgNPs-5FU showed homogeneous distribution and the nanoparticle size was in the range of 13–78 nm (Figure ). The Derived Count Rate (kcps) was 288.70, while the PDI value was 0.598. These values indicate that the drug-bound nanoparticles were both stable and at the desired concentration.
The DLS results of AgNPs (Fig a) and AgNPs-5FU (Fig b) are shown in Figure . While the average size of the AgNPs produced was about 2 nm, the nanoparticle size distribution also detected a particle at 3.615 nm. When the drug 5FU was bound to the AgNPs, the average particle size increased to 28 nm, and the largest particle was detected at 78.82 nm. While the PDI value of the AgNPs was 0.412, this value increased to 0.535 when the drug was bound. This implies that the nanoparticles are still stable despite the binding of the drug to the AgNPs, as indicated by the device alerts. While the surface charge of the synthesized AgNPs was −18.6 mV, it increased to −46.2 mV when the drug was bound (Figure (a,b)). The conductivity of the solution decreased from 0.0862 mS/cm to 0.0802 mS/cm for the AgNPs. It is well known that nano-drugs with negative surface charge are effective in cancer cells [Citation34].
In a previous study, we discovered that when we employed 4 × 10−3M NaBH4 and 4.28 × 10−3M trisodium citrate in the composition of AgNPs, the size of the AgNPs was less than 5 nm [Citation35]. As a result, when drug is bound to AgNPs, the NPs size is well below 100 nm. Unlike previous research, we obtained Ag-NPs smaller than 5 nm in our study, and when we loaded 5FU into AgNPs, the AgNPs-5FU size was smaller than in previous studies. It is well known that the size of nanoparticles has a substantial impact on cytotoxicity.
In Figure , the SEM image is given at a 100 nm scale, which confirms the size distribution. Looking at the SEM images, it was determined that AgNPs (Figure (a)) were around 10 nm. When 5FU drug was bound to AgNPs, it was observed that AgNPs-5FUs were around 20–30 nm. (Figure (b)). SEM images also confirm the DLS results.
The results of FTIR analysis of the study demonstrate various bonds shown at different peaks. Figure shows FTIR spectrums of the samples. In Figure (a), the first peak belonging to the -OH band is seen at 3252 cm−1, while in Figure (a) this peak has shifted to 3279 cm−1. A vibration band of the carboxylate group in trisodium citrate was observed at 1636 cm−1 for AgNP. When 5FU was bonded to the silver nanoparticles, it shifted to 1646 cm−1 due to the amide group of the drug. The characteristic peak of the Ag nanoparticle was clearly revealed at 628 cm−1 [Citation36]. In Figure (b), peaks of 2976–2872 cm−1 aliphatic symmetrical vibrations belonging to 5FU are seen. At the same time, two peaks of C–O stretching vibration belonging to AgNPs-5FU were determined at 1064–1040 cm−1, and peaks of C–H tension vibrations belonging to the ring structure at 878 cm−1 were determined [Citation37].
The UV-VIS spectrum of AgNPs and AgNPs-5FU is given in the Figure . A specific absorption band specific to AgNPs is observed at 403 nm in the spectrum of AgNPs. This band, which should be seen at 400 nm, has shifted to 403 nm [Citation38]. The reason for this is due to the fact that the absorption shifts to a lower wavelength as the particle size decreases with the quantum dot effect of the nanoparticle size. As expected, AgNPs-5FU dissolved in aqueous medium gave a specific absorption band at 330 nm. This is due to the excitations in n-σ*, σ-σ* and π-π* electronic transitions due to the amine, C=O and C=C groups in the structure of 5FU. Especially due to aromatic ring structures, π-π* electronic transitions are very common. As the particle size increased, the absorption band shifted to the higher wavelength. This is an indication that 5FU also chemically binds to AgNPs.
The Figure (a) shows the absorbance of 5FU and the Figure (b) shows drug release of 5FU from AgNPs and 5FU loading capacity of AgNPs. According to Figure (b), it is seen that after 30 hours, approximately 52% of 5FU is released, and after 60 hours approximately 70% of it is released. According to Figure (b), it is seen that the drug loading capacity is around 75% at the beginning, but it increases over time and is around 92.8% at the end of 60 hours. The conclusion demonstrated that the AgNPs have a sustained and controlled release characteristic (Figure ). After 50 hours, the diffusion of 5FU reached a balance. We successfully demonstrated controlled drug release of 5FU via AgNPs.
Figure 6. (a) Absorbance of AgNPs-5FU. (b) Drug Loading and Drug Release Capacity of AgNPs-5FU. (c) Fick Diffusion of AgNPs.
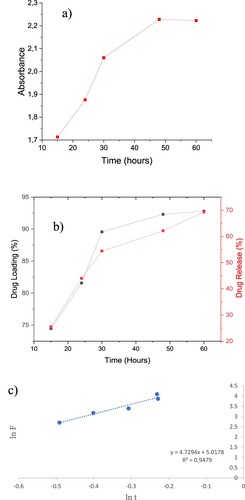
Fick’s law;
According to this equation, n: 4.7294 and k: 5.0178, kinetic parameters (n, k) examined by through lnF-Int. The fact that the exponential number n is greater than 1 indicates that there is a super diffusion mechanism. This shows that AgNPs-5FU diffuses very easily into the cell (Figure (c)).
Table and Figure show the cytotoxic effects of 5FU and AgNPs-5FU on breast cancer cells (MCF-7, MDA-MB-231, 4T1) and normal HUVEC cells.
Figure 7. The cytotoxic effect of 5FU, AgNPs-5FU and AgNPs on MCF-7, MDA-MB-231, 4T1 and HUVEC cell lines.
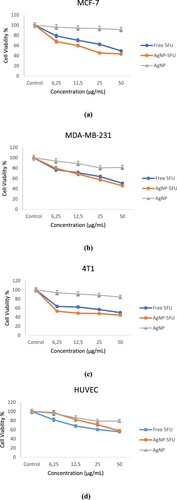
Table 1. The IC50 values of 5FU and AgNPs-5FU on MCF-7, MDA-MB-231, 4T1 and HUVEC cell lines.
Compared with AgNPs-5FU and 5FU in the same concentration range, our findings clearly showed that AgNPs 5FU had more cytotoxic on all cancer cells than free 5FU. Furthermore, they had no significant cytotoxicity on normal HUVEC cells. As indicated in Table , AgNPs-5FU was 4.64 times more effective on murine breast cancer cells and 2.14 times more effective on MCF-7 human breast cancer cells compared to free 5FU.
Our results showed that 4T1 and MCF-7 were more chemosensitive to AgNPs-5FU than MDA-MB-231. These cells are triple negative breast cancer cells lacking estrogen receptor (ER), progesterone receptor (PR) and human epidermal receptor 2 (Her2) [Citation39]. Therefore MDA-MB-231 cells are known to be highly aggressive and invasive breast cancer cells and resistant to many anti-cancer drugs [Citation40]. Nordin et al. applied hydromethanolic and ethyl acetate extracts to MCF-7 and MDA-MB-231 cells and found that the IC50 values of these extracts on MCF-7 were 57.35 and 54.98 g/mL, respectively, while the IC50 values of the same extracts for MDA-MB-231 were above 100 g/mL [Citation41]. Our results also support the finding that MDA-MB-231 cells are more resistant to anticancer therapy with 5FU and AgNPs-5FU.
In this study, the release mechanism of 5FU from AgNPs was investigated using Fick’s law and State II diffusion. According to Fick’s law, the n value was above 1 (Figure (c)). According to State II diffusion, the release mechanism of 5FU from AgNPs had a zero-order mechanism. In conclusion, it is possible that the Ag-5FU nanoparticle system can rapidly dissolve in water and diffuse into the cell, and this system below 100 nm can rapidly diffuse from the cell walls. Thus, the diffusion coefficient of the nano-sized drug increases rapidly. The increase in the diffusion coefficient allows the nanomedicine to affect more cancer cells than its free form, even at a lower dose [Citation42].
Recently, it has been demonstrated that drug loaded AgNPs are effective in treating cancer cells, including colon, lung, ovarian, and cervical cancers [Citation43, Citation44]. In a study with 5FU, the IC50 value was found to be 11.79 µM for MCF-7 cells [Citation45], while in another study, the IC50 values were 195.9 µM for BJ, 214.3 µM for DLD−1, and 202.2 µM for SK-MES−1 [Citation46]. Ashour et al., reported that the size of PEG/5FU-NPs were in the range of 176 and 253 nm and 5FU loaded AgNPs increased cytotoxicity in colon cancer cells compared to free 5FU [Citation47]. In another study, in which the size of chitosan-conjugated 5FU-NPs was 208 nm, the IC50 value of free 5FU was 4.21 g/mL, while the IC50 value of chitosan-conjugated 5FU-NPs was 3.43 g/mL in the caco-2 cell line [Citation48]. PEG coated Methotrexate-AgNPs (PEG-Ag-MTX) demonstrated improved anticancer activity against MCF-7 cells, with an IC50 of 258.6 g/mL for PEG-Ag-MTX versus MTX alone (512.7 g/mL) [Citation49]. In our study, IC50 value of 5FU was 49.23, while IC50 value of AgNPs-5FU was 23.006 on MCF -7 cells. The IC50 value of 5FU was 48.36, while the IC50 value of AgNPs-5FU was 10.41 on 4T1 cells. AgNPs-5FU was 4.64 times more effective than free 5FU cells on 4T1 cells while 2.14 times more effective on MCF-7 breast cancer cells. The increased efficacy of 5FU demonstrated that AgNPs-5FU can improve 5FU’s therapeutic effects on breast cancer cells. The findings show that AgNPs-FU demonstrated selective cytotoxicity against cancer cells.
4. Conclusion
Our findings display the predominant anticancer effect of AgNPs-5FU on MCF-7 and 4T1 cells while low toxicity to normal HUVEC cells and the anticancer effect of 5FU can be enhanced by silver nanoparticle-mediated nanodrug delivery systems. Thus, AgNPs-5FU may be a promising anticancer drug candidate that reduces the side effects of 5FU.
Disclosure statement
No potential conflict of interest was reported by the author(s).
Data availability statement
The data that support the findings of this study are available on request from the authors.
References
- Siegel RL, Miller KD, Fuchs HE, et al. (2022). Cancer statistics, 2022. CA Cancer J Clin.
- Hortobagyi GN, de la Garza Salazar J, Pritchard K, et al. The global breast cancer burden: variations in epidemiology and survival. Clin Breast Cancer. 2005;6:391–401. doi:10.3816/CBC.2005.n.043
- Tran P, Lee S-E, Kim D-H, et al. Recent advances of nanotechnology for the delivery of anticancer drugs for breast cancer treatment. J Pharm Investig. 2020;50:261–270. doi:10.1007/s40005-019-00459-7
- Mohammadi S, Salimi A, Hamd-Ghadareh S, et al. A FRET immunosensor for sensitive detection of CA 15-3 tumor marker in human serum sample and breast cancer cells using antibody functionalized luminescent carbon-dots and AuNPs-dendrimer aptamer as donor-acceptor pair. Anal Biochem 2018;557:18–26. doi:10.1016/j.ab.2018.06.008
- Howell A, Anderson AS, Clarke RB, et al. Risk determination and prevention of breast cancer. Breast Cancer Res. 2014;16:1–19.
- Liu W, Li X, Wong Y-S, et al. Selenium nanoparticles as a carrier of 5-fluorouracil to achieve anticancer synergism. ACS Nano. 2012;6:6578–6591. doi:10.1021/nn202452c
- Botticelli A, Scagnoli S, Roberto M, et al. 5-Fluorouracil degradation rate as a predictive biomarker of toxicity in breast cancer patients treated with capecitabine. J Oncol Pharm Pract. 2020;26:1836–1842. doi:10.1177/1078155220904999
- Nabih S, Hassn SS. Nonchemical integration of Au/Ag-based reduced graphene nanohybrid combined with 5-Fluorouracil drug to treat cancer cells. Life Sci 2021;272:119262. doi:10.1016/j.lfs.2021.119262
- Diasio RB, Harris BE. Clinical pharmacology of 5-fluorouracil. Clin Pharmacokinet. 1989;16:215–237. doi:10.2165/00003088-198916040-00002
- Entezar-Almahdi E, Mohammadi-Samani S, Tayebi L, et al. Recent advances in designing 5-fluorouracil delivery systems: a stepping stone in the safe treatment of colorectal cancer. Int J Nanomed. 2020;15:5445. doi:10.2147/IJN.S257700
- Visser GW, Gorree G, Peters GJ, et al. Tissue distribution of [18F]-5-fluorouracil in mice: effects of route of administration, strain, tumour and dose. Cancer Chemother Pharmacol 1990;26:205–209. doi:10.1007/BF02897200
- Bharali DJ, Khalil M, Gurbuz M, et al. How we got here, where we are going and being a cog in something turning. Int J Nanomed. 2009;4:1. doi:10.2217/17435889.4.1.1
- Di Paolo A, Danesi R, Falcone A, et al. Relationship between 5-fluorouracil disposition, toxicity and dihydropyrimidine dehydrogenase activity in cancer patients. Ann Oncol. 2001;12:1301–1306. doi:10.1023/A:1012294617392
- Mir MA, Akhter MH, Afzal O, et al. Design-of-experiment-assisted fabrication of biodegradable polymeric nanoparticles: in vitro characterization, biological activity, and in vivo assessment. ACS Omega. 2023;8:38806–38821.
- Karim S, Akhter MH, Burzangi AS, et al. Drug delivery challenges and current progress in nanocarrier-based ocular therapeutic system. Gels. 2022;8:82. doi:10.3390/gels8020082
- Chandran SP, Natarajan SB, Chandraseharan S, et al. Nano drug delivery strategy of 5-fluorouracil for the treatment of colorectal cancer. J Cancer Res Pract. 2017;4:45–48. doi:10.1016/j.jcrpr.2017.02.002
- Afzal O, Akhter MH, Ahmad I, et al. Mrna lipoplexes with cationic and ionizable α-amino-lipophosphonates: membrane fusion, transfection, mRNA translation and conformation. Pharmaceutics. 2022;14:581. doi:10.3390/pharmaceutics14030581
- Aziz SG-G, Aziz SG-G, Akbarzadeh A. Advances in silver nanotechnology: an update on biomedical applications and future perspectives. Drug Res. 2017;67:198–203. doi:10.1055/s-0042-112810
- Zhang X-F, Liu Z-G, Shen W, et al. Silver nanoparticles: synthesis, characterization, properties, applications, and therapeutic approaches. Int J Mol Sci. 2016;17:1534. doi:10.3390/ijms17091534
- Kumar V, Sharma N, Maitra S. In vitro and in vivo toxicity assessment of nanoparticles. Int Nano Lett. 2017;7:243–256. doi:10.1007/s40089-017-0221-3
- Kim YS, Kim JS, Cho HS, et al. Twenty-eight-day oral toxicity, genotoxicity, and gender-related tissue distribution of silver nanoparticles in Sprague-Dawley rats. Inhal Toxicol. 2008;20:575–583. doi:10.1080/08958370701874663
- Lee JH, Gulumian M, Faustman EM, et al. Blood biochemical and hematological study after subacute intravenous injection of gold and silver nanoparticles and coadministered gold and silver nanoparticles of similar sizes. Biomed Res Int. 2018;2018:1–10.
- Núñez C, Estévez SV, del Pilar Chantada M. Inorganic nanoparticles in diagnosis and treatment of breast cancer. J Biol Inorg Chem. 2018;23:331–345. doi:10.1007/s00775-018-1542-z
- Shen H, You J, Zhang G, et al. Cooperative, nanoparticle-enabled thermal therapy of breast cancer. Adv Healthc Mater. 2012;1:84–89. doi:10.1002/adhm.201100005
- Johannsen M, Thiesen B, Jordan A, et al. Magnetic fluid hyperthermia (MFH) reduces prostate cancer growth in the orthotopic Dunning R3327 rat model. Prostate. 2005;64:283–292. doi:10.1002/pros.20213
- Burridge K, Johnston J, Borrmann T. Photoactivity of nano-structured calcium silicate–titanium dioxide composite materials. J Mater Chem. 2011;21:1240–1245. doi:10.1039/C0JM02685A
- Abdel-Fattah WI, Ali GW. On the anti-cancer activities of silver nanoparticles. J Appl Biotechnol Bioeng. 2018;5:43–46.
- Ishiguro K, Yan IK, Lewis-Tuffin L, et al. Targeting liver cancer stem cells using engineered biological nanoparticles for the treatment of hepatocellular cancer. Hepatol Commun. 2020;4:298–313. doi:10.1002/hep4.1462
- Sanad MF, Abdellatif SO, Ghali HA. Enhancing the performance of photovoltaic operating under harsh conditions using carbon-nanotube thermoelectric harvesters. J Mater Sci Mater Electron. 2019;30:20029–20036. doi:10.1007/s10854-019-02371-0
- Danışman-Kalındemirtaş F, Kariper İA, Hepokur C, et al. Selective cytotoxicity of paclitaxel bonded silver nanoparticle on different cancer cells. J Drug Deliv Sci Technol. 2021;61:102265. doi:10.1016/j.jddst.2020.102265
- Mosmann T. Rapid colorimetric assay for cellular growth and survival: application to proliferation and cytotoxicity assays. J Immunol Methods. 1983;65:55–63. doi:10.1016/0022-1759(83)90303-4
- Fahad Ullah M. Breast cancer: current perspectives on the disease status. Breast Cancer Metast Drug Resist Challeng Progr. 2019;2019:51–64.
- Ji X, Lu Y, Tian H, et al. Chemoresistance mechanisms of breast cancer and their countermeasures. Biomed Pharmacother. 2019;114:108800. doi:10.1016/j.biopha.2019.108800
- Kalındemirtaş FD, Kariper İA, Sert E, et al. The evaluation of anticancer activity by synthesizing 5FU loaded albumin nanoparticles by exposure to UV light. Toxicol in Vitro. 2022;84:105435. doi:10.1016/j.tiv.2022.105435
- Hepokur C, Kariper İA, Mısır S, et al. Silver nanoparticle/capecitabine for breast cancer cell treatment. Toxicol in Vitro. 2019;61:104600. doi:10.1016/j.tiv.2019.104600
- Shaik MR, Khan M, Kuniyil M, et al. Plant-extract-assisted green synthesis of silver nanoparticles using Origanum vulgare L. extract and their microbicidal activities. Sustainability. 2018;10:913. doi:10.3390/su10040913
- Singh P, Tyagi G, Mehrotra R, et al. Thermal stability studies of 5-fluorouracil using diffuse reflectance infrared spectroscopy. Drug Test Anal. 2009;1:240–244. doi:10.1002/dta.41
- Gharibshahi L, Saion E, Gharibshahi E, et al. Influence of poly (vinylpyrrolidone) concentration on properties of silver nanoparticles manufactured by modified thermal treatment method. PLoS One. 2017;12:e0186094.
- Kim KM, Jung J. Upregulation of G protein-coupled estrogen receptor by Chrysin-nanoparticles inhibits tumor proliferation and metastasis in triple negative breast Cancer Xenograft model. Front Endocrinol. 2020;672:1–11.
- Zordoky BN, Bark D, Soltys CL, et al. The anti-proliferative effect of metformin in triple-negative MDA-MB-231 breast cancer cells is highly dependent on glucose concentration: implications for cancer therapy and prevention. Biochim Biophys Acta. 2014;1840:1943–1957. doi:10.1016/j.bbagen.2014.01.023
- Nordin ML, Abdul Kadir A, Zakaria ZA, et al. HPLC-DAD finger printing, antioxidant, cholinesterase, and α-glucosidase inhibitory potentials of a novel plant Olax nana. BMC Complem Altern Med. 2018;18:1–10. doi:10.1186/s12906-017-2057-9
- Siepmann J, Peppas NA. An introduction to the most cited papers in the history of advanced drug delivery reviews (1987–2012). Adv Drug Deliv Rev 2012;64:1–3. doi:10.1016/j.addr.2012.10.006
- Surapaneni SK, Bashir S, Tikoo K. Gold nanoparticles-induced cytotoxicity in triple negative breast cancer involves different epigenetic alterations depending upon the surface charge. Sci Rep. 2018;8:12295. doi:10.1038/s41598-018-30541-3
- Yuan Y-G, Zhang S, Hwang J-Y, et al. Silver nanoparticles potentiates cytotoxicity and apoptotic potential of camptothecin in human cervical cancer cells. Oxid Med Cell Longev. 2018;2018:1–21.
- Correia A, Silva D, Correia A, et al. Probing the occurrence of soluble oligomers through amyloid aggregation scaling laws. Biomolecules. 2018;8:108. doi:10.3390/biom8040108
- Tigu AB, Toma V-A, Mot AC, et al. The synergistic antitumor effect of 5-fluorouracil combined with allicin against lung and colorectal carcinoma cells. Molecules. 2020;25:1947. doi:10.3390/molecules25081947
- Ashour AE, Badran M, Kumar A, et al. Physical pegylation enhances the cytotoxicity of 5-fluorouracil-loaded PLGA and PCL nanoparticles. Int J Nanomed. 2019;14:9259–9273.
- Ullah S, Azad AK, Nawaz A, et al. 5-fluorouracil-loaded folic-acid-fabricated chitosan nanoparticles for site-targeted drug delivery cargo. Polymers. 2022;14:2010.
- Muhammad Z, Raza A, Ghafoor S, et al. PEG capped methotrexate silver nanoparticles for efficient anticancer activity and biocompatibility. Eur J Pharm Sci. 2016;91:251–255. doi:10.1016/j.ejps.2016.04.029