Abstract
This study aimed to investigate the inhibitory effect of Zuojin Pill (ZJP) on KYSE 150 cells and xenograft tumours of the digestive tract cancer – esophageal cancer (EC) and its underlying mechanism. Initially, in vivo research demonstrated that oral administration of ZJP effectively inhibited the growth of EC tumours, and in vitro studies revealed that ZJP suppressed the proliferation, migration, and invasion of cells. Then, network pharmacology prediction identified the core target, active ingredient, and involvement of the PI3K-Akt signalling pathway in ZJP treatment of EC. Cell studies validated that ZJP affected the expression of core targets, and its active ingredients inhibited the proliferation of cells. Additionally, ZJP was shown to induce cell apoptosis, decrease mitochondrial membrane potential, and increase the level of intracellular oxidative stress. Further experimental verifications suggested that the inhibitory effects of ZJP on EC might be attributed to the modulation of PI3 K/Akt signalling pathways.
1. Introduction
Esophageal cancer (EC), one of the most aggressive gastrointestinal cancers, has been recognized as a significant public health issue worldwide [Citation1]. Currently, available treatments primarily focus on esophagectomy and chemoradiotherapy [Citation2]. However, the 5-year relative survival rate for EC reaches only around 20% [Citation3], and esophagectomy severely impacts patients’ quality of life [Citation4]. Additionally, skin injury associated with chemoradiotherapy has become a serious concern [Citation5]. Consequently, it is imperative to conduct research and develop new and effective therapeutic interventions. Traditional Chinese medicine (TCM) has been used clinically in Asia for over 2,000 years and has gradually gained global acceptance due to its notable curative properties, abundant resources, and lower toxicity. An increasing number of TCM formulae have been clinically reported to manage cancer, either as a stand-alone or in conjunction with conventional treatment [Citation6–8].
Zuojin Pill (ZJP), a classical TCM formula listed in the Chinese Pharmacopeia [Citation9], has been prescribed to treat a wide range of gastrointestinal disorders in China since ancient times [Citation10]. It consists of two commonly used herbs, Coptis chinensis Franch (C. chinensis) and Euodia rutaecarpa (Juss.) Benth (E. fructus), in a 6:1 (W/W) ratio [Citation11]. However, despite its historical use, the therapeutic effects of ZJP on EC and its related mechanisms remain largely unexplored. Given ZJP's potential as a pharmacological candidate for EC treatment, identifying its effective compounds and understanding its underlying mechanisms are paramount for future research and development.
Multi-component, multi-pathway, and multi-target are inherent advantages of TCM formulae [Citation12]. However, the complexity of these formulas makes it exceptionally challenging to assess their effects and mechanisms of action through conventional pharmacological methods alone [Citation13,Citation14]. Network pharmacology has been proven to be a valuable tool in deciphering the molecular and pathway mechanisms of such complex system [Citation15,Citation16]. Therefore, this study employed a comprehensive research strategy. Initially, in vitro and in vivo experiments were performed to confirm ZJP's inhibitory effect on EC. Then, network pharmacology was utilized to obtain the active ingredients of ZJP and predict its potential therapeutic targets. Ultimately, in vitro experiments were further conducted to validate the mechanism and targets of ZJP in treating EC.
2. Material and methods
2.1. Reagents, materials and chemicals
Nude mice were purchased from Chengdu Dashuo Biotechnology Co., Ltd. (Chengdu, Sichuan; production license number: SCXK (Sichuan) 2015-030). KYSE 150 cells were purchased from Wuhan Pu-nuo-sai Life Technology Co. Ltd. (Wuhan, China). ZJP was purchased from Xiang Lian Yao Ye Co., Ltd (Beijing, China). RPMI-1640 culture medium was purchased from Gibco Co. (Grand Island, NY, United States). Quercetin, Isorhamnetin, Palmatine, Coptisine, and Berberrubine were purchased from Aladdin Biochemical Tech. Co., LTD (Shanghai, China). The total RNA extraction kits, cDNA synthesis kits, and RT-qPCR EasyTM (One Step)-SYBR Green I kit were purchased from Foregene Biotechnology Co., Ltd. (Chengdu, China). Cell counting kit-8 (CCK-8) was purchased from Boster Biol. Tech. (Wuhan, China). Hematoxylin and Eosin (H&E) were purchased from Beijing Bailingwei Technology (Beijing, China). EDU staining kit and acridine orange/ethidium bromide (AO/EB) staining kit were purchased from Sangon Biotech (Shanghai) Co., LTD (Shanghai, China). The assay kits for superoxide dismutase (SOD), catalase (CAT), and malondialdehyde (MDA) were purchased from Suzhou Michy Biomedical Technology Co., Ltd. (Suzhou, China). The assay kits for DCFH-DA and JC-1 were purchased from the US Everbright Inc. (Suzhou, China). Annexin V-FITC/PI assay kit and enhanced chemiluminescence luminescence (ECL) reagent were purchased from 4A Biotech (Beijing, China). The primary antibodies for PI3 K, phosphorylation (P)-PI3 K, Akt, P-Akt, Bax, Bcl-2, horseradish peroxidase-(HPR-) and fluorescence-conjugated secondary antibodies were purchased from ABclonal Biotech. Co., Ltd. (Wuhan, China). All other reagents used in the experiments were of analytical grade.
2.2. In vivo antitumor activity
Twenty-four male nude mice weighing 18 ± 2 g (4 weeks) were housed in an SPF animal facility. After seven days of acclimatization, KYSE 150 cells (1 × 106 cells) were subcutaneously injected into the right dorsal region of each mouse. Once the tumour reached approximately 100 mm3, the mice were randomized into four groups(6 mice/per group): a control group and three ZJP treatment groups (Low dose: 0.63 g/kg; Middle dose: 1.26 g/kg; High dose: 2.52 g/kg) [Citation17]. ZJPs at different doses were administered intragastrically for 14 consecutive days. Body weight and tumour volume were recorded at 2, 4, 6, 8, 10, 12 and 14 days post-grouping. The tumour volume was calculated using the formula: V = 1/2 × (length × width2). After 14 days of gavage, the mice were sacrificed, and tumour tissues were collected and fixed in 4% paraformaldehyde. The Animal Ethics Committee of Chengdu University of Traditional Chinese Medicine approved all animal experiments.
The fixed tumour tissues were dehydrated, embedded in paraffin, and sectioned (4-6µm). These sections were stained with H&E and examined under an optical microscope for morphological analysis. Images were captured with a BA200 Digital trinocular microscope camera system. Each slice was firstly observed at 20 times magnification, followed by 200 times magnification to examine specific Xenograft tumour lesions further. To assess the safety of ZJP, H&E staining was also performed on the liver and kidneys of nude mice.
2.3. In vitro antitumor activity
2.3.1. Cell culture and treatment
Throughout the study, KYSE 150 cells were used. These cells were cultured in RPMI-1640 medium, supplemented with 10% FBS and 1% penicillin/streptomycin. The cells were incubated in a humidified atmosphere of 5% CO2 at 37 °C, and the medium was changed every two days.
In 96-well plates, 1 × 104 cells/well cells were cultured. When the cells reached 70-80% confluence, they were treated with different concentrations of ZJP (2.5, 5, 10, 20, 40, 80, and 100 µg/mL). The media were removed after 12, 24, and 48 hours of incubation. In per well, 100 µL CCK-8 buffer (10 µL concentrate + 90 µL media) was added, and the cells were incubated for another 30 minutes. The absorbance of each well was then measured at 450 nm.
2.3.3. EDU staining
KYSE 150 cells (1 × 105 cells/well) were treated with 20, 40, and 80 µg/mL ZJP for 24 hours. Subsequently, 300 µL of EDU-containing medium was added to incubate the cells for 2.5 hours. Following incubation, these cells were treated with 4% paraformaldehyde, 2 mg/mL glycine, 0.5% Triton X-100, detection mixture, 0.5% Triton X-100, and Hoechst staining solution. Eventually, EDU-positive cells were observed and photographed using a laser confocal microscope (Leica, SP8 SR, Wetzlar, Germany).
2.3.4. Transwell and wound healing assay
In a serum-free medium, KYSE 150 cells (1 × 105 cells/well) were loaded into the upper chamber, while media containing 10% FBS was placed in the lower chamber. The cells were treated with ZJP of different concentrations (20, 40, and 80 µg/mL) for 24 h. The cells that migrated through the membrane were then fixed in 4% paraformaldehyde and stained with crystal violet for 5 minutes at room temperature. Stained cells were then counted using Image J software.
For the wound healing assay, 1 × 106 KYSE 150 cells were cultured in 6-well plates and incubated with a serum-free medium for 12 h once they reached about 90% confluence. A sterile pipette tip was then used to create a linear wound within the cell monolayers, and the cells were treated with ZJP for 24 h. Scratch images were obtained at 0, 6, 12, and 24 h, respectively, using an inverted microscope. The scratch area was calculated using Image J software.
2.4. Network analysis
2.4.1. Targets prediction of ZJP and EC
Based on criteria of Oral bioavailability (OB) ≥ 30% and drug-likeness (DL) ≥ 0.18, active compounds from C. chinensis and E. rutaecarpa were collected utilizing the Traditional Chinese Medicine Systems Pharmacology Database (TCMSP, https://old.tcmsp-e.com/index.php). The potential targets of these active compounds were obtained through TCMSP and the Swiss Target Prediction analysis platform (http://www.swisstargetprediction.ch/). These potential targets were then converted to standard gene names using the UniProt database (https://www.uniprot.org/).
The term “Esophageal cancer” was input into the Genecards database (http://www.Genecards.org) to identify relevant targets. The intersection targets between ZJP and EC were analyzed in the STRING database (https://sting-db.org/). Using Cytoscape software (ver.3.9.1), Protein–Protein Interaction (PPI) results were imported for visualization. The topological importance of nodes was assessed by calculating the “Degree” parameter in the PPI network.
The Drug-Compounds-Target-Disease (DCTD) diagram of compounds and targets of ZJP was constructed using Cytoscape software. In this network diagram, edges symbolize mutual relationships. Finally, by calculating the degree, the importance of each node was evaluated within the DCTD network.
2.4.4. Pathway enrichment analysis
Gene ontology (GO) function and the Kyoto Encyclopedia of genes and genomes (KEGG) were analyzed using Metascape (http://www.Metascape.org). The top 20 items of KEGG pathways and the top 10 items for the biological process (BP), cellular component (CC), and molecular function (MF) were presented.
2.5. Experimental validation
2.5.1. Quantitative real time-polymerase chain reaction (qRT-PCR)
qRT-PCR assays were used to determine the mRNA expressions of core targets. Briefly, KYSE 150 cells (2 × 105 cells/well) were cultured in 6-well plate and treated with ZJP (80 µg/mL). Following the instructions of the kit, total RNA extraction and cDNA synthesis were performed. The cDNA was then amplified according to the SYBR Green RT–PCR reaction kit instructions. GAPDH was employed as an internal standard for normalization. Table presents the qRT-PCR primers. Relative quantitative analysis of 2−△△CT method represented mRNA expression levels. All experiments were repeated three times.
Table 1. The sequences of primers used in qRT-PCR.
2.5.2. Impact of active ingredients on cell viability by CCK-8
In 96-well plates, 1 × 104 cells were cultured and treated with 3.125, 6.25, 12.5, 25, 50, and 100 µg/mL of five potential active compounds and incubated for 24 h. After removing the media, 100 µL of CCK-8 buffer was added to the cells for 30 minutes. The absorbance of each well was then measured at 450 nm.
2.5.3. AO/EB staining and mitochondrial membrane potential (MMP) assessment
KYSE 150 cells (1 × 105 cells /well) were treated with ZJP (20, 40, and 80 µg/mL) for 24 h and then with a proportional mix of AO/EB staining solution and JC-1 probe for 15 minutes in the dark. Morphology observation was performed under a laser confocal microscope,.
2.5.4. Cytometer assay of apoptosis and reactive oxygen species (ROS) level
KYSE 150 cells were treated as described above and collected using EDTA-free trypsin. For apoptosis assays, cells were resuspended in Annexin V binding buffer and diluted to 1 × 106 cells /ml. To 100 µL of cells, 5 µL of AnnexinV-FITC and 10 µL of PI staining solution were added. For ROS assay, cells were incubated with 10µM DCFH-DA, washed with PBS to remove excess DCFH-DA fluorescent probe, and then collected. Finally, the KYSE 150 cells were analyzed using a FACSCantoII Flow cytometer (BD Company, New York, NY, USA).
2.5.5. MDA, SOD, and CAT determination
As described above, cells were incubated with ZJP in 6-well plates. KYSE 150 cells (1 × 105 cells /well) were washed with PBS and lysed with lysis buffer to collect the total protein. The levels of MDA, SOD, and CAT in the cells were determined with commercial assay kits following the manufacturer's instructions.
2.5.6. Immunofluorescence assay of EC xenograft tumor
Well-fixed tumour tissues were dehydrated and sectioned into 4-6 µm frozen slices. The slices were placed at room temperature for 30 minutes, washed three times using PBS, and blocked with 2% BSA at room temperature. They were incubated overnight at 4 °C using primary antibodies of PI3 K (1:200), P-Akt (1:200), P-PI3 K (1:300), Akt (1:200), Bcl-2 (1:100), and Bax (1:200). Afterward, corresponding fluorescent secondary antibodies (1:150) were applied to the sections at room temperature for 2 h in the dark. Finally, the sections were washed and mounted with an anti-fluorescence quencher containing DAPI.
2.5.7. KYSE 150 cells immunofluorescence assay
KYSE 150 cells were seeded and treated in laser confocal plates as described above. The cells were treated sequentially with paraformaldehyde, 0.3% Triton X-100, and 10% serum. Primary antibodies of Akt (1:200), PI3 K (1:200), P-PI3 K (1:300), P-Akt (1:200), Bcl-2 (1:100), and Bax (1:200) were employed to incubate the cells overnight at 4 °C, and then corresponding fluorescent secondary antibodies (1:150) were applied at room temperature for 2 h. Last, the cells were washed and sealed with a fluorescent sealant containing DAPI.
2.5.8. Western blotting
KYSE 150 cells (5 × 106) were cultured in petri dishes and treated with ZJP for 24 h. After removing the supernatant and washing three times with cold PBS, RIPA lysis buffer was added to lysate the cells for 30 mins at 4°C to obtain total cell protein. Protein concentration was detected using the BCA protein assay kit, and the samples were diluted to the same concentration for analysis. The prepared proteins were isolated using SDS-PAGE and transferred to an activated PVDF membranes. The membrane was blocked with 5% fat-free milk for 1 h and then incubated overnight with the corresponding primary antibody PI3 K (1:1000), P-PI3 K (1:1000), Akt (1:1000), P-Akt (1:1000), Bcl-2 (1:1000), and Bax (1:1000) at 4°C. After washing with TBST three times, the membrane was incubated with HPR-conjugated antibody (1:2000) for 1 h at room temperature. Protein bands were visualized using an ECL kit, with GAPDH (1:50000) as an internal reference. Grayscale analysis of each blot was performed using Image J software.
2.6. Statistical analysis
Data are presented as mean ± standard deviations (SD). One-way analysis of variance (ANOVA) was used for data that met the criteria for normal distribution. LSD test was employed when variances were homogeneous. Otherwise, the Dunnett T3 test was applied. The Kruskal–Wallis H test was adopted for data not conforming to the normal distribution. Results were considered highly significant when P < 0.01 and statistically significant when P < 0.05.
3. Results
3.1. Inhibitory effect of ZJP on xenograft tumor
Figure shows the effects of ZJP (0.63, 1.26, and 2.52 g/kg) on tumour xenografts. Over the 2–14 d period, the control group of nude mice consistently weighed slightly more than ZJP treatment groups, likely due to their larger xenograft tumour volumes. However, no significant difference in body weight was observed among the four groups (P > 0.05) (Figure A).
Figure 1. Inhibitory effects of Zuojin Pill (ZJP) on KYSE 150 tumour xenografts. (A) Body weight change of nude mice; (B) Xenograft tumour volume change; (C) Xenograft tumour weight of nude mice; (D) Effects of ZJP on liver; (E) Effects of ZJP on kidney; (F) Effects of ZJP on KYSE 150 cells xenograft tumour. Values are indicated as mean ± SD (n = 6), *P < 0.05, **P < 0.01, compared with the control group.
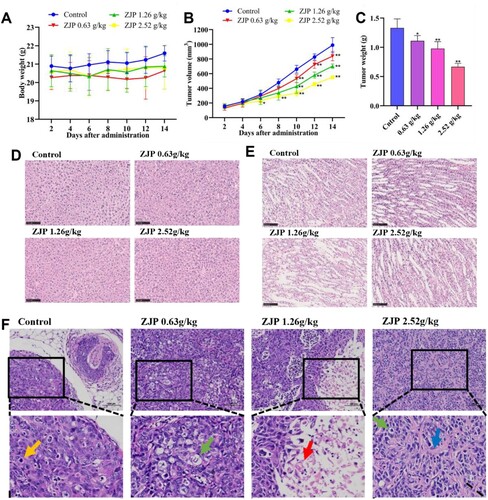
The tumour volume was recorded for four groups at 2, 4, 6, 8, 10, 12, and 14 days post-grouping, and the differences between the groups were calculated on the same day. Compared with the control group, the 2.52 g/kg ZJP group exerted a statistically significant inhibitory effect on tumour growth starting from day 6 (P < 0.05), whereas the 0.63 and 1.26 g/kg doses demonstrated significant effects from day 10 (P < 0.01). By day 14, the tumour volume was reduced by more than 50% with the administration of 2.52 g/kg ZJP (P < 0.01) (Figure B). Additionally, ZJP significantly reduced tumour weight (P < 0.05) (Figure C).
H&E staining of liver and kidney tissues indicated that ZJP did not cause damage to these organs in nude mice (Figure D-E). H&E staining of tumour xenografts revealed extensive tumour cell necrosis, pyknosis, and dissolution of nuclei, with swelling of the cytoplasm and vacuolization (green arrow) in the ZJP-treated groups compared to the control group. Tumour cell necrosis frequently led to the formation of cystic structures in local tumours (red arrows), and the tumour tissue exhibited increased interstitial hyperplasia and connective tissue collagen hyperplasia (blue arrow) (Figure F).
3.2. Inhibitory Effect of ZJP on KYSE 150 Cells
The proliferation of KYSE 150 cells was inhibited by ZJP in a dose-dependent and time-dependent manner (Figure A-C). After 24 hours of treatment, the half-maximum inhibitory concentration (IC50) of ZJP was observed to be 80 µg/mL. Based on these findings, concentrations of 20, 40, and 80 µg/mL were chosen for subsequent experiments to further investigate ZJP's effects on inhibiting KYSE 150 cell proliferation over a 24-hour period. The effect of these concentrations on KYSE 150 cells is depicted in Figure D. The inhibitory effect of ZJP on KYSE 150 cell proliferation was further validated by EDU staining (Figure E-F).
Figure 2. ZJP inhibitory effect on KYSE 150 cells. (A-C) Cell viability after treated with different concentrations of ZJP for 12, 24, and 48 h; (D) ZJP inhibitory effect on cell proliferation; (E-F) EDU staining results; (G-H) ZJP inhibitory effect on cell invasion; (I-J) ZJP inhibitory effect on cell migration. Values are expressed as mean ± SD (n = 3), #P > 0.05, *P < 0.05, **P < 0.01, compared with the control group.
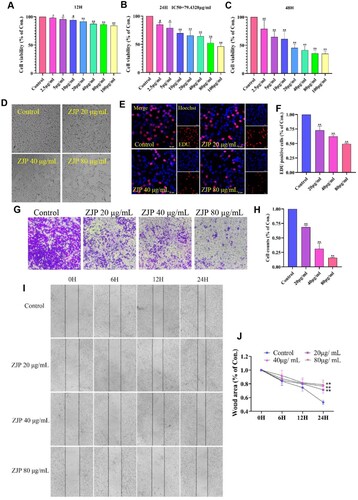
As shown in Figure G-H, ZJP at various concentrations significantly inhibited the invasion of KYSE 150 cells (P < 0.01). The wound area of cells at 0, 6, 12, and 24 h after ZJP administration was monitored. Statistical analysis of the wound area of the cells at 24 h revealed that ZJP effectively inhibited cell migration (P < 0.01) (Figure I-J).
3.3. Targets and pathway prediction for ZJP treatment of EC
From the TCMSP database, 34 targeted ZJP compounds were obtained, including nine from C. chinensis, 23 from E. rutaecarpa, and two common ingredients (Quercetin and Berberine) from both E. rutaecarpa and C. chinensis (Table ). After eliminating duplicate targets, 755 ZJP targets were obtained from the online database and previous studies. Additionally, the Genecard database yielded 953 related target genes. After intersecting the target genes of ZJP and EC, 206 intersection genes were identified as potential genes of ZJP treat EC (Figure A).
Figure 3. Prediction of anti-EC targets of ZJP. (A) Drug and disease intersection targets Venn diagram; (B) PPI network of intersection targets; (C) The top 20 genes; (D) Drug-Compounds-Target-Disease (DCTD) network diagram.
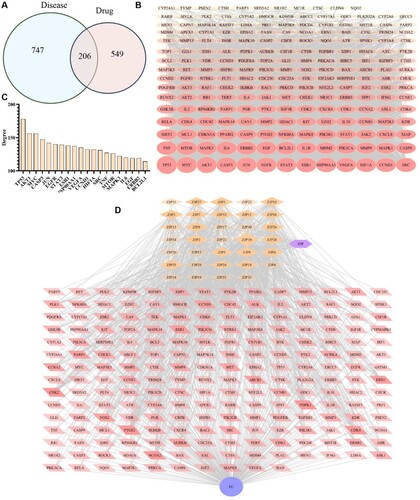
Table 2. Active compounds in ZJP.
A PPI network was constructed using the STRING database. For visualization, the 206 overlapping genes were imported into Cytoscape software, and the network is presented in Figure B. As shown in Figure C, the 20 most significant targets were selected based on node degree. The results highlighted the main potential targets of ZJP, including TP53, MYC, JUN, Akt1, EGFR, ESR1, CCND1, STAT3, CASP3, VEGFA, HSP90AA1, and SRC.
A DCTD network was created in the Cytoscape using 34 compounds and 206 intersecting targets, encompassing 242 nodes and 1409 edges. As shown in Figure D, 34 compounds interacted with multiple targets. The importance of each compound in the network was evaluated by calculating their degrees, with Quercetin, Isorhamnetin, Palmatine, Berberrubine, and Coptisine identified as the principal active compounds of ZJP.
The analyses of GO and KEGG were further performed. The GO analysis ranked the top ten types of biological processes (BPs), cellular components (CCs), and molecular functions (MFs) according to the p-values (Figure A-B). Notably, protein kinase activity and alcohol group as acceptor were the main items of MFs. While the BPs were associated with positive regulation of phosphorylation and protein phosphorylation. The principal CCs included plasma membrane signalling receptor complex and receptor complex. Additionally, multiple signalling pathways were enriched by KEGG analysis of 206 overlapping genes, with the top 20 pathways presented in the bubble chart (Figure C-D). Significant pathways included the PI3K-Akt signalling pathway, the pathway in cancer, apoptosis, pancreatic cancer, and hepatitis B.
3.4. Verify the core targets and active compounds of ZJP
As shown in Figure A, ZJP was observed to increase TP53 and CASP3 mRNA expression levels, while decreasing the mRNA expression of MYC, JUN, AKT1, CCND1, STAT3, SRC, ERBB2, and BCL2L1 (P < 0.05). However, ZJP did not significantly impact the mRNA expression of EGFR, ESR1, VEGFA, HSP90AA1, HIF1A, MTOR, MAPK3, TNF, and IL6.
Figure 5. (A) Effects of ZJP on mRNA expression of TP53, MYC, JUN, AKT1, EGFR, ESR1, CCND1, STAT3, CASP3, VEGFA, HSP90AA1, SRC, HIF1A, ERBB2, MTOR, MAPK3, TNF, IL6, and BCL2L1; (B) Inhibitory effects of Quercetin, Isorhamnetin, Palmatine, Berberrubine, and Coptisine chloride on KYSE 150 cells. Values are indicated as mean ± SD (n = 3), #P > 0.05, *P < 0.05, **P < 0.01, compared with the control group.
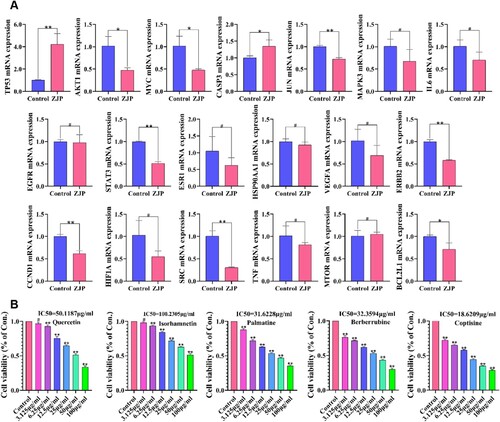
Figure B illustrates the inhibitory effects of the five active ingredients on KYSE cells. The IC50 of Quercetin, Isorhamnetin, Palmatine, Berberrubine, and Coptisine were 50.1187, 100.2305, 31.6228, 32.3594, and 18.6209 µg/mL, respectively. Among these, Coptisine demonstrated the most potent inhibitory effect on KYSE 150 cells.
3.5. ZJP induced KYSE 150 cell apoptosis and oxidative stress
The AO/EB staining principle lies in AO's ability to produce green fluorescence through intact cell membranes, while EB can only generate red fluorescence through damaged cell membranes. Hence, the red/green fluorescence intensity can be utilized to evaluate apoptosis. Compared with the control group, treatment with ZJP led to a notable increase in red fluorescence intensity and a sharp decrease in green fluorescence intensity (Figure A), indicating a potential enhancement of apoptosis in KYSE 150 cells by ZJP. Annexin V/PI staining was used to quantitatively investigate the apoptotic effect of ZJP on KYSE 150 cells, revealing a dose-dependent induction of apoptosis (Figure B-C).
Figure 6. Effects of ZJP on apoptosis, mitochondrial membrane potential (MMP), and oxidative stress in KYSE 150 cells. (A) AO/EB staining assays apoptotic; (B-C) Flow cytometry assays apoptotic; (D-E) JC-1 staining assays MMP; (F-G) The intracellular reactive oxygen species (ROS) levels in KYSE 150 cells; (H-J) The levels of MDA and activities of SOD and CAT in KYSE 150 cells. Values are expressed as mean ± SD (n = 3), #P > 0.05, *P < 0.05, **P < 0.01, compared with the control group.
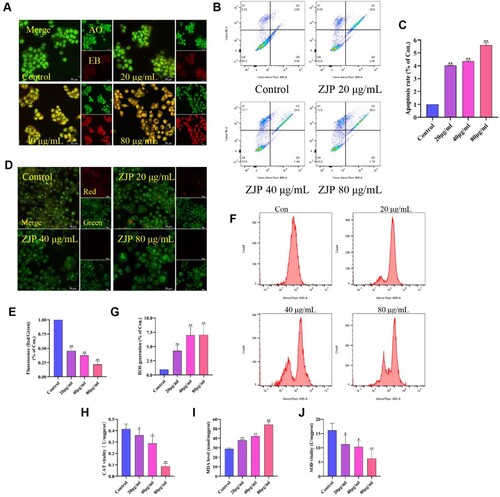
The reduction in MMP can induce cell apoptosis [Citation18]. Figure D-E illustrates the JC-1 detection results. ZJP intervention significantly decreased red fluorescence intensity and red/green fluorescence ratio (P < 0.01), suggesting that ZJP might induce apoptosis by disrupting MMP. Furthermore, ZJP enhanced KYSE 150 cells apoptosis, and the crucial apoptosis factors include excess of ROS [Citation19]. As shown in Figure F-G, exposure of KYSE 150 cells to ZJP led to a sharp increase in intracellular ROS content.
As depicted in Figure H, ZJP at concentrations of 40 and 80 µg/mL reduced CAT levels to 0.28 U/mg (P < 0.05) and 0.08 U/mg, respectively (P < 0.01). However, ZJP at 20 µg/mL had no significant effect on CAT. MDA levels in KYSE 150 cells increased to 37.88 , 42.07, and 54.40 nmol/mg when treated with ZJP at 20, 40, and 80 µg/mL (P < 0.01) (I). ZJP at 80 µg/mL reduced the SOD content of KYSE 150 cells to 6.22 U/mg (P < 0.01). Nonetheless, ZJP at 20 and 40 µg/mL reduced SOD without significant differences (J). These findings suggested that ZJP might induce cellular injury through oxidative stress.
3.6. Mechanism of ZJP on EC
The effect of ZJP on EC might involve the PI3 K/Akt and apoptosis signalling pathways, as indicated by the findings from GO and KEGG analyses. Hence, immunofluorescence and western blot were utilized to delve into the molecular mechanisms underlying the pro-apoptotic effect of ZJP in both KYSE 150 cells and xenograft tumours. The immunofluorescence results from xenograft tumours preliminarily demonstrated that ZJP could inhibit PI3 K and Akt protein phosphorylation and suppress Bcl-2 protein expression (Figure A-F). Then, immunofluorescence was adopted to examine the expression of PI3 K, P-PI3 K, Akt, P-Akt, Bax, and Bcl-2 proteins in KYSE 150 cells and found that the ratio of P-PI3 K/PI3 K, and P-Akt/Akt and Bcl-2/Bax was reduced (P < 0.01) (Figure G-L). Furthermore, western bolt results revealed no significant difference in the expression of PI3 K and Akt among the groups (P > 0.05). However, the levels of Bcl-2, P-PI3 K, and P-Akt were decreased, while the expression of Bax was increased, indicating that ZJP could effectively inhibit the PI3 K/Akt signalling pathway and promote cell apoptosis (Figure M-P).
Figure 7. Immunofluorescence and western blot results of xenograft tumour tissue and KYSE 150 cells. (A-F) Effects of ZJP on P-PI3 K/PI3 K, P-Akt/Akt, and Bax/Bcl-2 level in xenograft tumours; (G-L) Effects of ZJP on P-Akt/Akt, P-PI3 K/PI3 K, and Bax/Bcl-2 level of KYSE 150 cells; (M-P) Western blot detecting the protein level of PI3 K, P-PI3 K, Akt, P-Akt, Bax and Bcl-2. GAPDH was used as the sample loading control. Values are expressed as mean ± SD (n = 3), #P > 0.05, *P < 0.05, **P < 0.01, compared with the control.
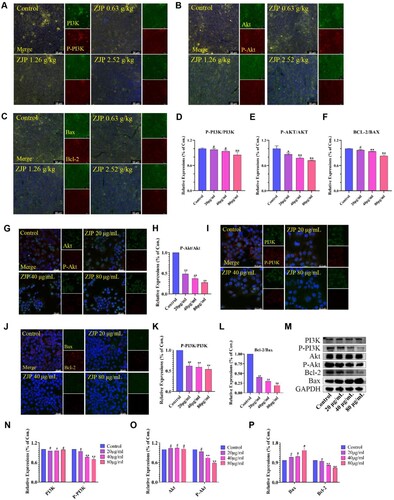
4. Discussion
The tumour xenograft animal model provides a sophisticated platform for studying tumorigenesis in vivo [Citation20]. To further explore the role of ZJP in EC development, KYSE 150 cells (1 × 106 cells) were subcutaneously injected into the right dorsal side of nude mice to establish a xenograft tumour model in this study. The results indicated that ZJP reduced the volume and weight of tumours in these animals without causing toxicity to the kidneys and liver. Morphologically, H&E staining analysis of xenograft tumours revealed that ZJP induced significant necrosis and vacuolization of tumour cells in xenograft tumours. These findings tentatively suggest that ZJP may inhibit EC development.
EC is an aggressive tumour typically diagnosed in advanced stages. The absence of serosa facilitates its rapid spread to adjacent mediastinal structures. Even at an early stage, the extensive lymphatic drainage network accelerates tumour diffusion [Citation21]. Hence, this study assessed the inhibitory effects of ZJP on human EC squamous cells (KYSE 150 cells). Results of CCK-8 and EDU staining demonstrated that ZJP inhibited the growth of the KYSE 150 cells in time – and dose-dependent manners. Additionally, Transwell and wound-healing assays demonstrated that ZJP could effectively inhibit the migration and invasion of KYSE 150 cells. All these findings are consistent with previous results showing that ZJP can suppress the growth of xenogeneic tumours.
TCM formulae have played essential roles in disease prevention and treatment [Citation22,Citation23], yet deciphering their mechanism of action remains challenging due to their complex components and multifaceted targets. However, the development of network pharmacology has provided a pragmatic approach to a more comprehensive and sophisticated understanding of their effects and underlying mechanisms [Citation24]. The TCMSP, a highly efficient system pharmacology platform [Citation25], facilitated the screening of 34 chemical constituents of ZJP in this study. The Swiss Target Prediction, a web server that accurately predicts the targets of bioactive molecules based on 2D and 3D similarity measurements with known ligands [Citation26], was employed to obtain 755 targets of 34 constituents from TCMSP, Swiss Target Prediction, and literature sources. The GeneCards, which allows efficient navigation of genes and disease information [Citation27], retrieved 953 EC-related targets. The subsequent intersection of potential target genes of ZJP and EC yielded 206 overlapping genes. Furthermore, the PPI network diagram was constructed by STRING database, and the 206 overlapping genes were visualized using Cytoscape. PI3 K/Akt and apoptosis pathways were predicted to be the primary pathways attributed to the potential effect of ZJP on EC. Nonetheless, these predictions are based solely on database analyses, and the authenticity and feasibility of the 2D network drug prediction remain unverified, necessitating the subsequent experimental validation to substantiate these findings.
The effect of ZJP on 20 core targets was assessed through qRT-PCR analysis. Our findings revealed that ZJP upregulated TP53 and CASP3 mRNA expression, while downregulating MYC, JUN, AKT1, CCND1, STAT3, SRC, ERBB2, and BCL2L1 mRNA expression levels. Currently, the constituents isolated from ZJP include alkaloids, glycosides, flavonoids, cinnamates, lactones, and alcohols. Through the construction of the DCTD Network diagram, the major active ingredients of ZJP were identified as Quercetin, Isorhamnetin, Palmatine, Berberrubine, and Coptisine. This experiment validated that these five active ingredients exerted inhibitory effects on KYSE 150 cells. The therapeutic effects of ZJP on EC were associated with PI3 K/Akt and apoptotic pathways, as predicted by KEGG analysis. AO/EB staining and flow cytometry illustrated that ZJP promoted apoptosis in KYSE 150 cells. Reduction in MMP is considered a vital indicator of mitochondrial dysfunction and a precursor to apoptosis [Citation28], and the accumulation of ROS is closely related to MMP disruption [Citation29]. Our results revealed that ZJP could reduce MMP in KYSE 150 cells, highlighting its potential to induce MMP depolarization to promote apoptosis. Intervention with ZJP could significantly increase the ROS and MDA content in KYSE 150 cells, while also inhibiting the activities of CAT and SOD. Furthermore, PI3K-Akt signal pathway has been shown to play a crucial role in tumour development and influence tumour responses to cancer therapies [Citation30]. Mutations in the PI3 K and AKT genes are common in cancer patients. Small molecule inhibitors targeting the major kinase components of the PI3 K/AKT signalling pathway are a prominent research focus in cancer therapy [Citation31]. AKT is the downstream signalling molecule of PI3 K. When PI3 K is activated, AKT will also be phosphorylated and activated [Citation32]. AKT regulates downstream effectors such as GSK3β and mTOR, thereby regulating cell survival, growth, and metabolism [Citation33]. In this study, immunofluorescence staining and western blot analysis validated that ZJP could inhibit the expressions of P-PI3 K, P-Akt, and Bcl-2 and reduce the P-PI3 K/PI3 K, P-Akt/Akt, and Bcl-2/Bax ratios.
5. Conclusions
In conclusion, this research systematically identified compounds in ZJP and explored their therapeutic effect on EC through network pharmacology, as well as in vivo and vitro experiments. Furthermore, the study demonstrated that ZJP might exert its therapeutic action on EC by modulating the PI3 K/Akt signalling pathway to promote apoptosis. However, further investigation is warranted to determine whether ZJP can induce EC cell death via multiple pathways. Additional verification of the targets and mechanisms of ZJP's active ingredients is also needed. Moreover, identifiying active ingredients in ZJP through network pharmacology screening presents certain limitations. The next phase should involve experimental screening to pinpoint and validate its key active components.
Supplementary Materials.docx
Download MS Word (480.7 KB)Acknowledgments
The authors would like to thank the reviewers for all helpful comments to improve their manuscript.
Disclosure statement
No potential conflict of interest was reported by the author(s).
Additional information
Funding
References
- Short MW, Burgers KG, Fry VT. Esophageal cancer. Am Fam Physician. 2017 Jan 1;95(1):22–28. PMID: 28075104.
- Watanabe M, Otake R, Kozuki R, et al. Recent progress in multidisciplinary treatment for patients with esophageal cancer. Surg Today. 2020 Jan;50(1):12–20. doi:10.1007/s00595-019-01878-7
- Siegel RL, Miller KD, Fuchs HE, et al. Cancer statistics, 2022. CA Cancer J Clin. 2022 Jan;72(1):7–33. doi:10.3322/caac.21708
- Kato H, Nakajima M. Treatments for esophageal cancer: a review. Gen Thorac Cardiovasc Surg. 2013 Jun;61(6):330–335. doi:10.1007/s11748-013-0246-0
- Yang X, Ren H, Guo X, et al. Radiation-induced skin injury: pathogenesis, treatment, and management. Aging (Albany NY). 2020 Nov 16;12(22):23379–23393. doi:10.18632/aging.103932
- Guo W, Huang J, Wang N, et al. Integrating network pharmacology and pharmacological evaluation for deciphering the action mechanism of herbal formula zuojin pill in suppressing hepatocellular carcinoma. Front Pharmacol. 2019 Oct 9;10:1185, doi:10.3389/fphar.2019.01185
- Wang N, Pei H, Xing LW. Natural regulatory T cells and bifunctional regulatory T cells: potential targets of traditional Chinese medicine in treating colitis-associated colon cancer. Integr Med Discov. 2024; 8:e24006. doi:10.53388/IMD202408006
- Lu C, Ke L, Li J, et al. Chinese medicine as an adjunctive treatment for gastric cancer: methodological investigation of meta-analyses and evidence Map. Front Pharmacol. 2022 Jan 10;12:797753, doi:10.3389/fphar.2021.797753
- Commission CP, Wan Z. The pharmacopoeia of the people’s republic of China. Beijing: China Medical Science Press; 2020, p. 802.
- Zhao YL, Shi WL, Shan LM, et al. Differences in effects of Zuojin Pills and its similar formulas on Wei cold model in rats. Chin J Integr Med. 2009 Aug;15(4):293–298. doi:10.1007/s11655-009-0293-7
- Huang S, Zhang Z, Li W, et al. Network pharmacology-based prediction and verification of the active ingredients and potential targets of Zuojinwan for treating colorectal cancer. Drug Des Devel Ther. 2020 Jul 14;14:2725–2740. doi:10.2147/DDDT.S250991
- Wang K, Miao X, Kong F, et al. Integrating network pharmacology and experimental verification to explore the mechanism of effect of zuojin pills in pancreatic cancer treatment. Drug Des Devel Ther. 2021 Sep 4;15:3749–3764. doi:10.2147/DDDT.S323360
- Li RL, Wang LY, Liu S, et al. Natural flavonoids derived from fruits are potential agents against atherosclerosis. Front Nutr. 2022 Mar 24;9:862277, doi:10.3389/fnut.2022.862277
- Peng W, He CX, Li RL, et al. Zanthoxylum bungeanum amides ameliorates nonalcoholic fatty liver via regulating gut microbiota and activating AMPK/Nrf2 signaling. J Ethnopharmacol. 2024 Jan 10;318(PtA):116848. doi:10.1016/j.jep.2023.116848
- Li CJ, Ma XP, Nie H. Mechanism of Shenxiong glucose injection against myocardial ischemia-reperfusion injury based on network pharmacology and molecular docking. Zhongguo Zhong Yao Za Zhi. 2022 May;47(10):2759–2766. doi:10.19540/j.cnki.cjcmm.20211108.401
- Wang Z, Li S. Network pharmacology in quality control of traditional Chinese medicines. Chin Herb Med. 2022 Sep;2022;14(4):477–478. doi:10.1016/j.chmed.2022.09.001
- Wei Y, Ren S, Wang R, et al. Based on network pharmacology to explore the potential bioactive compounds and mechanisms of zuojin pill for the treatment of ulcerative colitis. Evid Based Complement Alternat Med. 2021 Aug 26;2021:7567025, doi:10.1155/2021/7567025
- Jelic MD, Mandic AD, Maricic SM, et al. Oxidative stress and its role in cancer. J Cancer Res Ther. 2021 Jan-Mar;17(1):22–28. doi:10.4103/jcrt.JCRT_862_16
- Hao M, Liu R. Molecular mechanism of CAT and SOD activity change under MPA-CdTe quantum dots induced oxidative stress in the mouse primary hepatocytes. Spectrochim Acta A Mol Biomol Spectrosc. 2019 ;220:117104, doi:10.1016/j.saa.2019.05.009
- Behzadi R, Ahmadpour S, Amiri FT, et al. Choosing the right protocol to establish MCF-7 tumor xenograft in nude mice. Anticancer Agents Med Chem. 2023;23(2):222–226. doi:10.2174/1871520622666220517090735
- Zhang Q, Chen WW, Sun X, et al. Hbo1 induces histone acetylation and is important for non-small cell lung cancer cell growth. Int J Biol Sci. 2022 May 16;18(8):3313–3323. doi:10.7150/ijbs.72526
- Liu P, Xu H, Shi Y, et al. Potential molecular mechanisms of plantain in the treatment of gout and hyperuricemia based on network pharmacology. Evid Based Complement Alternat Med. 2020 Oct 22;2020:3023127, doi:10.1155/2020/3023127
- Ru J, Li P, Wang J, et al. Tcmsp: a database of systems pharmacology for drug discovery from herbal medicines. J Cheminform. 2014 Apr 16;6:13, doi:10.1186/1758-2946-6-13
- Gfeller D, Grosdidier A, Wirth M, et al. Swisstargetprediction: a web server for target prediction of bioactive small molecules. Nucleic Acids Res. 2014 Jul;42(Web Server issue):W32–W38. doi:10.1093/nar/gku293
- Stelzer G, Rosen N, Plaschkes I, et al. The GeneCards suite: from gene data mining to disease genome sequence analyses. Curr Protoc Bioinformatics. 2016 Jun 20;54:1.30.1–1.30.33. doi:10.1002/cpbi.5
- Peng W, Wu JG, Jiang YB, et al. Antitumor activity of 4-O-(2″-O-acetyl-6″-O-p-coumaroyl-β-D-glucopyranosyl)-p-coumaric acid against lung cancers via mitochondrial-mediated apoptosis. Chem Biol Interact. 2015 May 25;233:8–13. doi:10.1016/j.cbi.2015.03.014
- Peng W, Hu C, Shu Z, et al. Antitumor activity of tatariside F isolated from roots of Fagopyrum tataricum (L.) Gaertn against H22 hepatocellular carcinoma via up-regulation of p53. Phytomedicine. 2015 Jul 15;22(7-8):730–736. doi:10.1016/j.phymed.2015.05.003
- Luo M, Zheng Y, Tang S, et al. Radical oxygen species: an important breakthrough point for botanical drugs to regulate oxidative stress and treat the disorder of glycolipid metabolism. Front Pharmacol. 2023 May 12;14:1166178, doi:10.3389/fphar.2023.1166178
- Pistritto G, Trisciuoglio D, Ceci C, et al. Apoptosis as anticancer mechanism: function and dysfunction of its modulators and targeted therapeutic strategies. Aging (Albany NY). 2016 Apr;8(4):603–619. doi:10.18632/aging.100934
- Li RL, Zhang Q, Liu J, et al. Hydroxy-α-sanshool possesses protective potentials on H2O2-stimulated PC12 cells by suppression of oxidative stress-induced apoptosis through regulation of PI3 K/AKT signal pathway. Oxid Med Cell Longev. 2020 Jul 9;2020:3481758, doi:10.1155/2020/3481758
- Yu L, Wei J, Liu P. Attacking the PI3 K/AKT/mTOR signaling pathway for targeted therapeutic treatment in human cancer. Semin Cancer Biol. 2022;85:69–94. doi:10.1016/j.semcancer.2021.06.019
- Peng Y, Wang Y, Zhou C, et al. Pi3 K/AKT/mTOR pathway and its role in cancer therapeutics: are We making headway? Front Oncol. 2022;12:819128, doi:10.3389/fonc.2022.819128
- Luo Q, Du R, Liu W, et al. Pi3 K/AKT/mTOR signaling pathway: role in esophageal squamous cell carcinoma, regulatory mechanisms and opportunities for targeted therapy. Front Oncol. 2022;12:852383, doi:10.3389/fonc.2022.852383