Abstract
The distinct influences of eastern Pacific (EP) and central Pacific (CP) La Niña on rainfall anomalies over Southeast Asia and Australia in boreal autumn (September to November) are explored in this study. Composite results reveal that CP La Niña gives rise to significant and severe flooding over Southeast Asia and Australia, whereas EP La Niña fails to exert any evident impacts on rainfall over this region. This difference can be attributed to the distinct features of cooling sea surface temperature anomalies (SSTAs) between EP and CP La Niña. With a more westward location and stronger intensity of the negative SSTAs during CP La Niña autumn, the highest and lowest SLP anomalies are substantially enhanced and shift westwards too, further causing intense easterly winds over the western Pacific and westerly anomalies over the Indian Ocean driven by this SLP gradient. Subsequently, robust low-level convergence and high-level divergence is observed over the Maritime Continent and Australia, resulting in significant above-normal rainfall anomalies in those regions. In contrast, weak and eastern Pacific–confined cooling SSTAs during EP La Niña produce correspondingly weak low-level convergence over the Maritime Continent conditions that make it hard for significant rainfall anomalies to arise.
摘要
本文分析了东太平洋型和中太平洋型La Niña事件对东南亚及澳大利亚秋季降水的不同影响。合成分析结果表明东太平洋型La Niña事件对该地区降水影响较弱,而中太平洋型La Niña事件易于导致东南亚及澳大利亚东北部降水偏多。这种影响差异主要由于两类La Niña事件的冷海温位置和强度差异造成的。中太平洋型La Niña事件的冷海温偏西偏强,导致中西太平洋的西风异常以及印度洋的东风异常强度较强,并进一步引起海洋大陆区域的低层辐合增强,因此造成了显著的正降水异常。相反,东太平洋型La Niña事件的冷海温异常偏东偏弱,低层风场偏弱,相应地,低层辐合也偏弱,因此未能造成显著的降水异常发生。
1. Introduction
ENSO is the most important air–sea interactive mode in the tropics (e.g. McPhaden and Zhang Citation2009; Neelin et al. Citation1998). The warm phase of ENSO can extend tropical western Pacific convection more eastwards due to the spread of the warm pool, while the cold phase of ENSO constrains convection to a small zone because of a shrinking of the warm pool. Change in the convective location tends to stimulate climate anomalies around the tropics and extratropics through inducing atmospheric circulation anomalies by releasing latent heat, which is the dominant way ENSO influences climate (e.g. Lau and Nath Citation2003).
More importantly, ENSO-induced climate anomalies are complicated and not easy to predict, because of the complex properties of ENSO in many aspects. The spatial pattern is one such aspect. Based on the different locations of warming or cooling sea surface temperature anomalies (SSTAs), two types of ENSO have been recognized recently (Ashok et al. Citation2007; Kug, Jin, and An Citation2009). One type is termed central Pacific (CP) ENSO or ENSO Modoki, in which the dominant warming or cooling SSTAs are in the tropical central Pacific (Kao and Yu Citation2009). The other type is called eastern Pacific (EP) ENSO, and is characterized by the warming or cooling SSTAs being in the tropical eastern Pacific (Kao and Yu Citation2009). Many studies have documented that CP El Niño and EP El Niño exert considerably different climate influences (e.g. Chen and Tam Citation2010; Feng et al. Citation2011; Weng, Behera, and Yamagata Citation2009; Zhang, Jin, and Turner Citation2014). For example, Zhang et al. (Citation2011) found CP and EP El Niño show an obvious opposite impact on the western North Pacific (WNP) during boreal autumn. Specifically, CP (EP) El Niño is often accompanied by a weak anomalous WNP cyclone (anticyclone), and then different climate responses over the East Asian region appear. Composite results reported by Feng et al. (Citation2011) suggested that the two types of El Niño often give rise to different East Asian summer monsoon rainfall anomalies. EP El Niño generally leads to severe flooding around the Yangtze River valley in boreal summer, while CP El Niño results in above-normal rainfall over the Huaihe River, extending northwards to the Yellow River.
However, investigation of the impacts of the two types of La Niña has not been attempted as much as for the two types of El Niño. One possible reason may be because of the argument as to whether La Niña can indeed be classified into EP and CP types. Kug and Ham (Citation2011) concluded that the anomalous SST patterns between EP and CP La Niña are less distinct than those between EP and CP El Niño. Nevertheless, more and more studies have found that EP and CP La Niña show significantly different climate impacts (Ding et al. Citation2017; Song et al. Citation2017; Yuan and Yan Citation2013). Zhang et al. (Citation2015) revealed that EP La Niña is accompanied by a negative North Atlantic Oscillation (NAO), while CP La Niña shows a completely opposite NAO pattern and climate anomalies. Feng, Chen, and Li (Citation2017) showed that CP La Niña induces a winter quasi-annular mode in the mid–high latitudes in the Northern Hemisphere. By contrast, EP La Niña often triggers wave patterns with alternately positive and negative height anomalies. In this study, we aim to explore the different climate impacts of EP and CP La Niña during their developing autumns – a period when the spatial patterns between EP and CP La Niña are more distinct than during their mature winters.
It is known that the Southeast Asian and Australian regions often experience severe drought and flooding disasters, threatening peoples’ lives and leading to substantial economic losses. One cause for such calamitous events derives from the most dominant air–sea coupled event, i.e. ENSO. Considering the importance of rainfall anomalies in Southeast Asia and Australia, it is therefore desirable to thoroughly explore the different impacts of EP and CP La Niña on the rainfall anomalies of these regions.
The structure of this paper is organized as follows: Section 2 introduces the data and methods used in the study. Section 3 depicts the influences of the two types of La Niña on Southeast Asian and Australian rainfall anomalies during boreal autumn. Also explored in this section are the underlying physical mechanisms. Finally, a summary is given in Section 4.
2. Data and methods
The monthly atmospheric reanalysis data are from the National Centers for Environmental Prediction–National Center for Atmospheric Research. The data cover the period from 1948 to the present day, and their resolution is 2.5° latitude by 2.5° longitude in the horizontal direction, with 17 levels in the vertical direction (Kalnay et al. Citation1996). Hadley Center Global Sea Ice and Sea Surface Temperature data are employed to analyze the SST patterns of the two types of La Niña (Rayner et al. Citation2003). This SST data-set covers a long-term period and dates back as early as the year 1870. The monthly land precipitation data (Precipitation Reconstruction Over Land) are from the National Oceanic and Atmospheric Administration Climate Prediction Center (Chen et al. Citation2002).
Two indices are used to define the two types of La Niña. One is the Niño3 index, which is calculated by the area-averaged SSTAs over the region (5°S–5°N, 150°–90°W). The other is the El Niño Modoki index (EMI), which is defined by area-averaged SSTAs over the tropical central Pacific (10°S–10°N, 165°E–140°W) minus SSTAs over the western (10°S–20°N, 125°–145°E) and eastern (15°S–5°N, 110°–70°W) Pacific. Following the criterion established by Feng, Chen, and Li (Citation2017), EP (CP) La Niña events are chosen when the normalized Niño3 (EMI) index is less than −0.8, as well as EMI (Niño3). Accordingly, seven EP La Niña events (1955, 1967, 1970, 1975, 1984, 2005, and 2007) are identified, and seven CP La Niña events (1973, 1983, 1988, 1998, 1999, 2000, and 2010) too. The rationality of this criterion in separating EP and CP La Niña was justified in Feng, Chen, and Li (Citation2017).
3. Results
Figure shows the composite land rainfall anomalies in boreal autumn (September to November) during the developing phase of EP and CP La Niña. Despite most of Southeast Asia being covered by above-normal rainfall anomalies for EP La Niña, little of the region exceeds the 90% confidence level. Conversely, CP La Niña gives rise to severe flooding over all of Southeast Asia and, more importantly, nearly all the rainfall anomalies pass the 90% confidence level. Particularly, with respect to Australian rainfall anomalies, the two types of La Niña show markedly distinct influences: EP La Niña fails to exert any impacts on Australian rainfall, while CP La Niña triggers significantly above-normal rainfall anomalies over northeastern Australia. Based on the above results, we can conclude that EP La Niña typically exerts much less of an influence on Southeast Asian and Australian rainfall than CP La Niña. Therefore, we should pay attention when EP La Nina is used as a forecast factor.
Figure 1. Composite autumn mean (September–November) rainfall anomalies (units: mm d−1) during (a) EP La Niña and (b) CP La Niña years.
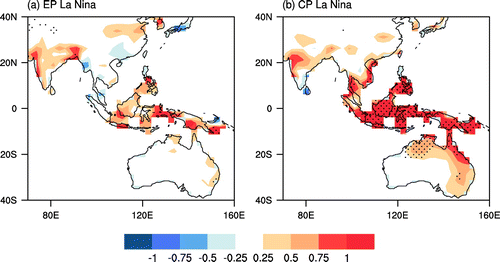
Furthermore, the related physical mechanisms are illustrated in Figures . Elucidating the dissimilarity between EP and CP La Niña in terms of SSTA distribution is pivotal to understanding the different impacts of EP and CP La Niña. Composite anomalous SST and sea level pressure (SLP) are plotted in Figure . During EP La Niña autumns, negative SSTAs appear mainly in the tropical eastern Pacific, with the maximum value centered at 105°W (Figure (a)), consistent with a typical EP La Niña defined by previous studies (e.g. Ashok et al. Citation2007). In contrast to EP La Niña, the dominant negative SSTAs of CP La Niña move to the tropical central Pacific (Figure (b)). It can be seen that CP La Niña is generally much stronger than EP La Niña. However, in terms of El Niño, the opposite situation is observed, as CP El Niño is usually much weaker than EP El Niño (Feng, Chen, and Li Citation2017). Additionally, it is worth noting that there are positive SSTAs over the western Pacific for CP La Niña, but not for EP La Niña. These distinct anomalous SST features for the two types of La Niña will trigger different atmospheric circulation anomalies. This is the key point to finding out the reasons why EP and CP La Niña cause different rainfall anomalies.
Figure 2. Composite SST anomalies (shading; units: °C) and SLP anomalies (contours; units: hPa) during (a) EP La Niña and (b) CP La Niña autumns.
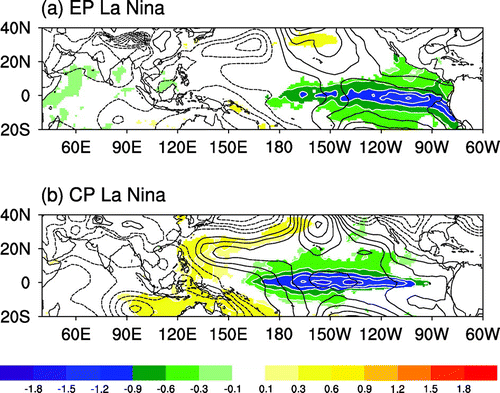
Figure 3. Composite 1000 hPa wind anomalies (vectors) and zonal wind speed (shading) during (a) EP La Niña and (b) CP La Niña autumns.
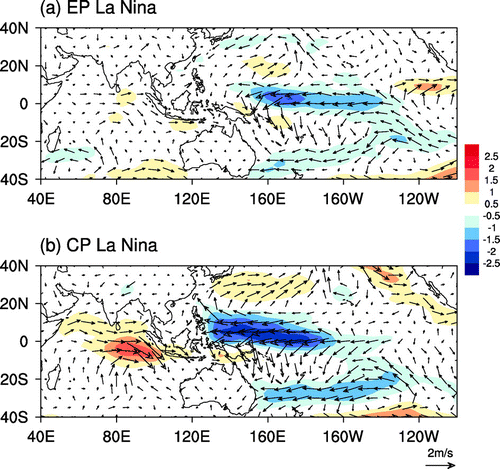
Figure 4. Composite 1000 and 200 hPa velocity potential (shading; units: 105 m2 s−1) and divergent winds (vectors) during (a, c) EP La Niña and (b, d) CP La Niña.
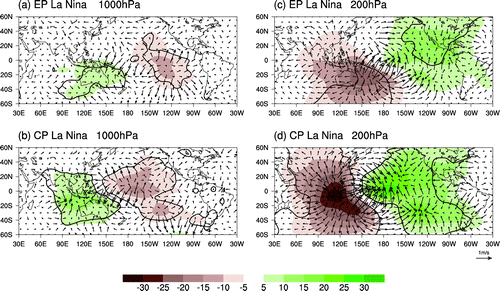
The cooling SSTAs of EP La Niña induce high SLP anomalies over the eastern Pacific and low SLP anomalies over the western Pacific and Indian Ocean (Figure (a)). However, CP La Niña–induced high SLP anomalies cover a larger domain and extend from the eastern Pacific to central Pacific, with the maximum value at about 150°W, corresponding to the maximum SSTA zone. At the same time, low SLP anomalies are pushed more westwards to the Maritime Continent and Indian Ocean (Figure (b)). Note that both high and low SLP anomalies are intensely enhanced during CP La Niña compared to EP La Niña, consistent with the stronger SSTAs of CP La Niña autumns. In addition, the warm SSTAs in the western Pacific during CP La Niña make a positive contribution to the strongly enhanced low SLP around the Maritime Continent, favoring the formation of positive rainfall anomalies.
SSTA-induced distinct anomalous SLP distributions will cause different surface wind anomalies in the tropics (Lindzen and Nigam Citation1987), which are shown in Figure . In the case of EP La Niña, the highest anomalous SLP is in the eastern Pacific and the lowest in the South China Sea. Therefore, the SLP gradient drives easterly wind anomalies blowing from the eastern to the western Pacific, and weak westerly wind anomalies from the Indian Ocean to the Maritime Continent (Figure (a)). The strongest SLP gradient lies in the tropical central Pacific around 170°E to 170°W (Figure (a)), yielding the strongest easterly wind anomalies, as seen in Figure (a). On the eastern side of this strongest easterly wind, there is a divergent zone at the low level and a convergent zone at the high level (Figure (a) and (c)), consistent with the cooling SSTAs. On the western side, a low-level convergent zone and high-level divergent zone over the Maritime Continent is observed (Figure (a) and (b)), with ascending motion being established, which is favorable for the positive rainfall anomalies there. However, such convergence and divergence is not strong enough to result in significant rainfall anomalies.
In contrast, with the westward movement of the highest and lowest SLP anomalies during CP La Niña, the easterly wind anomalies driven by this SLP gradient are observed in the central and western Pacific (Figure (b)). Along with the strongest SLP gradient shifting westwards (Figure (b)), the strongest easterly winds move to the western Pacific (Figure (b)). These shifts finally lead to the divergent and convergent zones travelling westwards at the same time (Figure (b) and (d)). Note that the anomalous easterly winds over the Pacific and the westerly over the Indian Ocean are both much stronger than those for EP La Niña, which is caused by the more significantly enhanced cooling SSTAs and correspondingly stronger SLP anomalies. Therefore, the associated low-level convergence and high-level divergence over the Maritime Continent and Australia is more obvious (Figure (b) and (d)), thereby causing more significantly positive rainfall anomalies when CP La Niña happens.
Additionally, with respect to Australia during CP La Niña, anomalous northwest winds over the South Pacific can transport plentiful moisture to the northeast part of the Australian continent. Together with the low-level convergence, excessive rainfall is thus observed. However, since the EP La Niña–induced anomalous northwest winds are located far from the Australian islands, it can hardly exert any impact on the Australian rainfall.
4. Summary
In this study, the distinct influences of EP and CP La Niña on rainfall anomalies over Southeast Asia and Australia in boreal autumn are explored through composite analysis. It is found that CP La Niña successfully exerts an evident impact, with significant and positive rainfall anomalies over Southeast Asia and Australia. However, EP La Niña fails to induce rainfall anomalies over this region. The related physical mechanisms responsible for this distinct impact between EP and CP La Niña are further analyzed. During EP La Niña, cooling SSTAs are weak and confined to the eastern Pacific, while CP La Niña is often characterized by strong cooling SSTAs located in the tropical central Pacific and warming SSTAs in the western Pacific, which is missing during EP La Niña. These inconsistent features of SSTAs between EP and CP La Niña give rise to anomalous SLP differences in its intensity and distribution. With the westward movement of cooling SSTAs, CP La Niña gives rise to the highest and lowest SLP anomalies being located more westwards and with much greater strength. Correspondingly, intense easterly winds and westerly anomalies driven by the SLP gradient are observed over the tropical western Pacific and Indian Ocean, respectively, during CP La Niña autumns. Subsequently, robust low-level convergence and high-level divergence can form over the Maritime Continent and Australia, which causes significant and positive rainfall anomalies in those regions. However, for EP La Niña, this convergence is considerably weakened due to weak wind anomalies, resulting in insignificant influences on rainfall anomalies. These findings suggest that when La Niña is used as a predictor, it is necessary to classify La Niña into two types.
Disclosure statement
No potential conflict of interest was reported by the authors.
Funding
This study was supported by the National Natural Science Foundation of China [grant number 41230527]; [grant number 41675091]; [grant number 41461164005].
References
- Ashok, K., S. Behera, S. Rao, H. Y. Weng, and T. Yamagata. 2007. “El Nino Modoki and Its Possible Teleconnection.” Journal of Geophysical Research 112: 505. doi:10.1029/2006/JC003798.
- Chen, G., and C. Tam. 2010. “Different Impacts of Two Kinds of Pacific Ocean Warming on Tropical Cyclone Frequency Over the Western North Pacific.” Geophysical Research Letters 37: L01803. doi:10.1029/2009GL041708.
- Chen, M., P. Xie, J. Janowiak, and P. Arkin. 2002. “Global Land Precipitation: A 50-yr Monthly Analysis Based on Gauge Observations.” Journal of Hydrometeorology 3: 249–266. doi:10.1175/1525-7541.
- Ding, S. Y., W. Chen, J. Feng, and H. F. Graf. 2017. “Combined Impacts of PDO and Two Types of La Nina on Climate Anomalies in Europe.” Journal of Climate 30: 3253–3278. doi:10.1175/JCLI-D-16-0376.1.
- Feng, J., W. Chen, C.-Y. Tam, and W. Zhou. 2011. “Different Impacts of El Nino and El Nino Modoki on China Rainfall in the Decaying Phases.” International Journal of Climatology 31: 2091–2101.10.1002/joc.v31.14
- Feng, J., W. Chen, and Y. J. Li. 2017. “Asymmetry of the Winter Extra-tropical Teleconnections in the Northern Hemisphere Associated with Two Types of ENSO.” Climate Dynamics 48: 2135–2151. doi:10.1007/s00382-016-3196-2.
- Kalnay, E. M., R. Kanamitsu, W. Kistler, D. Collins, D. Deaven, M. Gandin, S. Iredell, et al. 1996. “The NCEP/NCAR 40-Year Reanalysis Project.” Bulletin of the American Meteorological Society 77: 437–471.10.1175/1520-0477(1996)077<0437:TNYRP>2.0.CO;2
- Kao, H. Y., and J. Y. Yu. 2009. “Contrasting Eastern-Pacific and Central-Pacific Types of ENSO.” Journal of Climate 22: 615–632.10.1175/2008JCLI2309.1
- Kug, J., and Y.-G. Ham. 2011. “Are There Two Types of La Nina?” Geophysical Research Letters. doi:10.1029/2011GL048237.
- Kug, J., F. Jin, and S. An. 2009. “Two Types of El Nino Events: Cold Tongue El Nino and Warm Pool El Nino.” Journal of Climate 22: 1499–1515.10.1175/2008JCLI2624.1
- Lau, N. C., and M. J. Nath. 2003. “Atmosphere–Ocean Variations in the Indo-Pacific Sector During ENSO Episodes.” Journal of Climate 16: 3–20.10.1175/1520-0442(2003)016<0003:AOVITI>2.0.CO;2
- Lindzen, R. S., and S. Nigam. 1987. “On the Role of Sea Surface Temperature Gradients in Forcing Low-Level Winds and Convergence in the Tropics.” Journal of the Atmospheric Sciences 44: 2418–2436.10.1175/1520-0469(1987)044<2418:OTROSS>2.0.CO;2
- McPhaden, M. J., and X. Zhang. 2009. “Asymmetry in Zonal Phase Propagation of ENSO Sea Surface Temperature Anomalies.” Geophysical Research Letters 36: 2399. doi:10.1029/2009GL038774.
- Neelin, J. D., S. B. David, A. C. Hirst, F.-F. Jin, Y. Wakata, T. Yamagata, and S. E. Zebiak. 1998. “ENSO Theory.” Journal of Geophysical Research 103: 14261–14290. doi:10.1029/97JC03424.
- Rayner, N., D. E. Parker, E. B. Horton, C. K. Folland, L. V. Alexander, D. P. Rowell, E. C. Kent, and A. Kaplan. 2003. “Global Analyses of Sea Surface Temperature, Sea Ice, and Night Marine Air Temperature Since the Late Nineteenth Century.” Journal of Geophysical Research. doi:10.1029/2002JD002670.
- Song, L., S. Chen, W. Chen, and X. Chen. 2017. “Distinct Impacts of Two Types of La Niña Events on Australian Summer Rainfall.” International Journal of Climatology 37: 2532–2544. doi:10.1002/joc.4863.
- Weng, H., S. Behera, and T. Yamagata. 2009. “Anomalous Winter Climate Conditions in the PACIFIC Rim During Recent El Niño Modoki and El Niño Events.” Climate Dynamics 32: 663–674. doi:10.1007/s00382-008-0394-6.
- Yuan, Y., and H. M. Yan. 2013. “Different Types of La Niña Events and Different Responses of the Tropical Atmosphere.” Chinese Science Bulletin 58: 406–415. doi:10.1007/s11434-012-5423-5.
- Zhang, W., F.-F. JIN, J. Li, and H.-L. Ren. 2011. “Contrasting Impacts of Two-type El Nino Over the Western North Pacific During Boreal Autumn.” Journal of the Meteorological Society of Japan 89: 563–569.10.2151/jmsj.2011-510
- Zhang, W., F.-F. Jin, and A. G. Turner. 2014. “Increasing Autumn Drought Over Southern China Associated with ENSO Regime Shift.” Geophysical Research Letter 41. doi:10.1002/2014GL060130.
- Zhang, W., L. Wang, B. Q. Xiang, L. Qi, and J. H. He. 2015. “Impacts of Two Types of La Nina on the NAO During Boreal Winter.” Climate Dynamics 44: 1351–1366.10.1007/s00382-014-2155-z