ABSTRACT
The authors investigate the characteristics of propagation and the influence on tropical precipitation of 9–29-day intraseasonal variation over midlatitude East Asia during boreal winter, and find that the intraseasonal wind signal can propagate both eastward and southward. In the case of eastward propagation, the intraseasonal wind signal is mainly confined to the midlatitudes, featuring eastward migration of anomalous cyclones and anticyclones. In the case of southward propagation, intraseasonal meridional wind perturbations may extend from the mid to the low latitudes, and even the equatorial region. The accompanying wind convergence/divergence induces anomalous precipitation in the near-equatorial regions, forming a north–south dipole precipitation anomaly pattern between the southern South China Sea and the eastern China–Japan region. An anomalous meridional overturning circulation plays an important role in linking tropical and midlatitude intraseasonal wind and precipitation variations.
Graphical Abstract
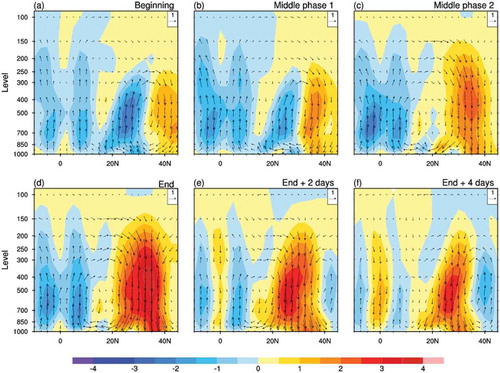
摘要
本文研究冬季东亚中纬度地区9–29天季节内变化的传播特征和其对热带降水的影响。分析发现风场的季节内信号既可以向东传播,也可以向南传播。向东传播时,风场的季节内信号主要限于中纬度地区,表现为气旋和反气旋的向东移动。向南传播时,源于中纬度的经向风扰动可以深入到低纬度地区,甚至到达赤道附近。伴随的辐合辐散引起赤道附近地区降水异常,形成南海南部和中国东部-日本之间一个南北向偶极型降水异常。一个异常经向翻转环流在连接热带和中纬度季节内风场和降水变化中起着重要作用。
1. Introduction
Intraseasonal variations have been identified over the mid–high latitudes of East Asia in boreal winter (Jeong et al. Citation2005, Citation2008; Park et al. Citation2010; He, Lin, and Wu Citation2011; Yang and Li Citation2016; Yao et al. Citation2016; Song et al. Citation2016; Song, Wu, and Jiao Citation2018), and they have a large influence on the weather and climate over East Asia. For example, Song, Wu, and Jiao (Citation2018) showed that intraseasonal oscillations play a large role in sustaining persistent strong cold events, such as the one in late-January 2016 – the so-called ‘century cold wave’, which set a new lowest-temperature record in many low-latitude East Asian regions (Song and Wu Citation2017). Thus, it is important to understand the characteristics, influences, and processes of intraseasonal variations over East Asia in boreal winter.
Most previous studies about intraseasonal variations over East Asia in boreal winter focus on their influence on temperature in mid–high latitude and subtropical regions (Jeong et al. Citation2005; He, Lin, and Wu Citation2011; Yang and Li Citation2016; Yao et al. Citation2016; Song et al. Citation2016; Song, Wu, and Jiao Citation2018). Here, we investigate plausible impacts of intraseasonal variations over midlatitude East Asia in other regions — in particular, precipitation in the tropics. Some studies have indicated that intraseasonal sea surface temperature and rainfall variations in the South China Sea may be induced by a southward propagating intraseasonal wind signal (Wu and Chen Citation2015; Wu Citation2016). Cao and Wu (Citation2017, Citation2018) revealed that northerly wind anomalies related to the East Asian winter monsoon play a dominant role in 10–20-day intraseasonal rainfall variations over the southern South China Sea and near the east coast of the Philippines in boreal winter. However, these studies only considered the southward propagating signal from the subtropical region. The question remains as to whether the midlatitude intraseasonal wind signal over midlatitude East Asia can propagate to the equatorial region and contribute to tropical rainfall intraseasonal variations.
In the present study we examine the propagation characteristics of intraseasonal wind variations over midlatitude East Asia and their influence on intraseasonal fluctuations of tropical rainfall. The rest of the paper is organized as follows: The data and methods used in the study are described in Section 2. Section 3 shows two different propagation directions of the wind field at the 9–29-day time scale. Section 4 presents the effect of southward propagating intraseasonal wind variations from midlatitude East Asia on tropical rainfall. A summary and discussion are provided in the final section.
2. Data and methods
The present study utilizes daily data from the NCEP-DOE Reanalysis-2 (Kanamitsu et al. Citation2002), which is available since 1979. Variables used include meridional and zonal wind, vertical velocity on 2.5°×2.5° grids at pressure levels, and surface wind at 10 m on T62 Gaussian grids. Precipitation is derived from the GPCP 1° daily precipitation dataset (Huffman et al. Citation2001), which is available since October 1996.
Our analysis focuses on intraseasonal variations on the 9–29-day time scale. Previous studies have indicated that boreal winter intraseasonal variability over East Asia displays obvious peaks above and below a 30-day time period (Yang and Li Citation2016; Yao et al. Citation2016; Cao and Wu Citation2017), and the characteristics of intraseasonal variations with 10–20-day and 30–60-day periods are different (Ye and Wu Citation2015). Meanwhile, our comparison shows that the variance of intraseasonal wind variations in winter in East Asia is dominated by that with a period shorter than 30 days (figure not shown). This study focuses on the intraseasonal signal in boreal winter covering November through March (NDJFM). Our analysis covers the time period from 1979/80 to 2015/16, except for the precipitation that is available from 1996 to 2015.
Following the method of Wu and Chen (Citation2015) and Wu (Citation2016), the intraseasonal variations on the 9–29-day time scale are obtained by a 9-day running mean minus a 29-day running mean. Composite analysis is utilized to obtain common features for different types of cases. The Student’s t-test is used to estimate the level of statistical significance for the composite anomalies.
3. Propagation characteristics of intraseasonal variation over East Asia
Lower-level wind is a fundamental element of the East Asian winter monsoon system and it has a direct impact on weather. So, we analyze the meridional wind variations at 850 hPa to detect the characteristics of intraseasonal variation over midlatitude East Asia. ) show the first three EOF modes of the meridional wind variations at 850 hPa on the 9–29-day time scale in the domain (15°–60°N, 90°–150°E) during NDJFM from 1979/80 to 2015/16.
Figure 1. The (a–c) first three EOF modes of meridional wind variations at 850 hPa, (d) lead–lag correlation between PC1, PC2, and PC3 during NDJFM from 1979/80 to 2015/16, and (e) lead–lag correlation between the area-mean meridional wind at 850 hPa in the north region (frame in (a)), east region (frame in (b)), and south region (frame in (c)) during NDJFM of 1992 and 1999 on the 9–29-day time scale.
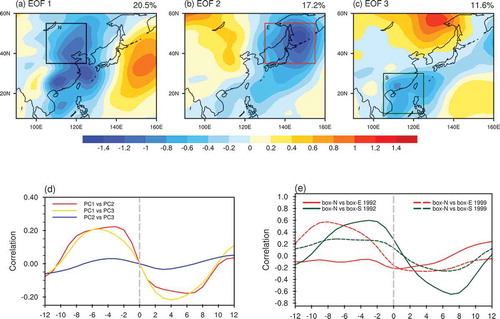
The three modes account for about 20.5%, 17.2%, and 11.6% of the 9–29-day variance, respectively. The leading mode has large negative loading along the east coast of the Asian continent ()). The second mode has large negative loading extending from the Sea of Okhotsk to the subtropical western North Pacific. The third mode displays a north–south contrast of loading between the midlatitudes and the subtropics. The principal component of the first mode (PC1) leads that of the second mode (PC2) and the third mode (PC3) by about 3–9 days ()). This lead–lag correlation and the spatial distribution indicate that the intraseasonal wind signal may propagate both eastward and southward. This feature is confirmed by the lead–lag correlation of area-mean 850-hPa meridional wind variations on the 9–29-day time scale in three regions as shown in , which are denoted as the north, east, and south region, respectively. ) displays two examples for the lead–lag correlation between the north region and the east/south region. In 1992, the correlation of meridional wind between the south and north region is above 0.4 when the meridional wind in the north region leads by 1–7 days, whereas the correlation between the east and north region is weak. This indicates that the intraseasonal wind signal is dominated by a southward propagation in 1992. In 1999, the correlation of meridional wind between in the east and north is above 0.4 when the meridional wind in the north region leads by 4–11 days, whereas the correlation between the south and north region is relatively weak (below 0.3). This suggests that the intraseasonal wind signal has a larger eastward propagation component in 1999.
To investigate the propagation characteristics further, we examine the day-to-day variations of the 9–29-day filtered area-mean meridional wind in the three regions in every year. Then, we select eastward and southward propagation cases based on the following criteria. First, we identify the peak days when the meridional wind values in the north region are greater than one standard deviation of the whole time series. Then, if the meridional wind value in the north region is declining and at the same time the meridional wind value in the east (south) region is rising to more than one standard deviation within a time interval of 12 (10) days, it is considered as an eastward (a southward) propagation case. The peak day of the meridional wind in the north and east (south) region is considered as the beginning and end phase of each case, respectively. In order to differentiate eastward from southward propagation, we only consider those monodirectional propagation cases. In other words, those cases when the meridional wind in both the east and south regions rises to one standard deviation after the peak of the meridional wind in the north region are excluded in the following analysis. The selection of the 12- or 10-day time interval is based on the temporal evolution of the area-mean meridional wind in the three regions. According to the above criteria, we identify 44 eastward propagation cases and 36 southward propagation cases. The time interval varies from case to case. The average time interval between the beginning and end phases for the eastward and southward propagation cases is 7 and 6 days, respectively. The maximum time interval is 12 and 10 days, respectively. presents the composite 850-hPa wind anomalies for the two types of propagation cases. Middle phases 1 and 2 are determined by dividing the time interval between the beginning and end phases by 3 for each individual case.
Figure 2. Composite 850-hPa wind anomalies (units: m s−1) for eastward and southward propagation cases on the 9–29-day time scale during NDJFM from 1979/80 to 2015/16. Only wind anomalies that are significant at the 90% confidence level are plotted. The wind scale is shown in the bottom-right corner.
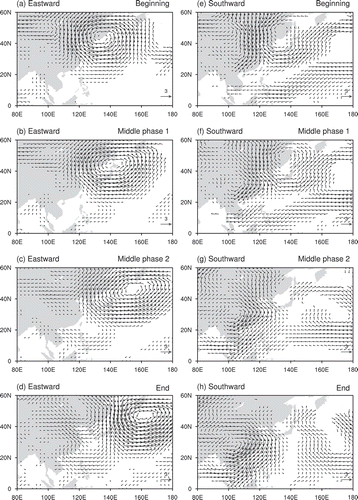
The composite 850-hPa wind-anomaly evolution shows clear differences between the eastward and southward propagation cases. In the beginning phase, a large anomalous cyclone is located over the Sea of Japan in both cases (). In comparison, an anomalous cyclone and anomalous northerly winds have a larger meridional extension in the southward propagation cases. In the eastward propagation cases, the anomalous cyclone remains intact and just moves eastward to the midlatitude North Pacific (). In the end phase, an anomalous anticyclone controls northeastern China ()). Given an average time interval of 7 days from the beginning to end phase, the average moving speed is about 4° of longitude per day. In the southward propagation cases, the anomalous cyclone weakens and anomalous northerly winds migrate southward to the equatorial region (). In the end phase, the whole South China Sea is covered by strong anomalous northeasterly winds, with anomalous convergence and divergence over the southern South China Sea and eastern China, respectively ()). These results indicate that the eastward propagation mainly features the eastward migration of anomalous cyclones and anticyclones and southward propagation is characterized by southward migration of anomalous northerly winds from midlatitude East Asia to the equatorial region.
4. Impact of intraseasonal variation on tropical precipitation
Previous studies found that intraseasonal variations in tropical precipitation around the Maritime Continent have a linkage to East Asian winter monsoon activity. Large rainfall anomalies over the southern South China Sea are preceded by strong northerly wind anomalies in the northern South China Sea and subtropical western North Pacific (Wu and Chen Citation2015; Cao and Wu Citation2017, Citation2018). Section 3 shows that a distinct northerly wind signal on the intraseasonal time scale can propagate from midlatitude East Asia to the equatorial region. In this section, we examine the intraseasonal rainfall variations in the southward propagation cases. displays the composite rainfall and 10-m wind anomalies from the beginning phase to 4 days after the end phase for 14 southward propagation cases during 1996/97 to 2014/15.
Figure 3. Composite precipitation (color shading; units mm d−1) and 10-m wind anomalies (vectors; units: m s−1) for southward propagation cases on the 9–29-day time scale during NDJFM from 1996/97 to 2014/15. Dark red dots denote precipitation anomalies that are significant at the 90% confidence level. Only wind anomalies that are significant at the 90% confidence level are plotted. The wind scale is shown in the bottom-right corner.
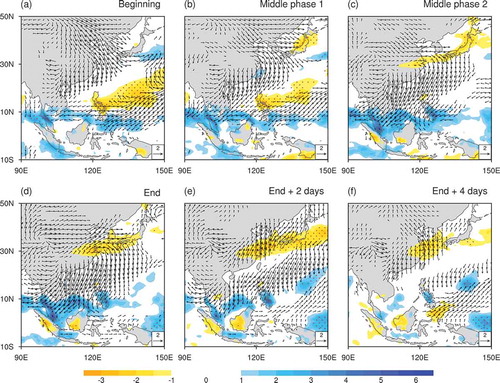
In the beginning phase, the precipitation anomaly distribution displays a tripole pattern from the tropics to the midlatitudes ()). Positive rainfall anomalies are observed over the eastern China–subtropical western North Pacific region in association with anomalous northerly wind convergence. Negative rainfall anomalies along 10°–20°N are attributable to an anomalous anticyclone over the tropical western North Pacific. The southern South China Sea has positive rainfall anomalies due to anomalous northerly wind convergence. Following the southward migration of anomalous northerly winds, positive rainfall anomalies in the north move southward and are replaced by negative rainfall anomalies (). At the same time, negative rainfall anomalies along 10°–20°N weaken. In the end phase, negative rainfall anomalies increase over the eastern China–Japan region following a switch in the anomalous wind direction that leads to anomalous divergence ()). In the meantime, positive rainfall anomalies over the southern South China Sea increase because of enhanced anomalous wind convergence. In the following days, both positive rainfall anomalies in the southern China Sea and negative rainfall anomalies over eastern China decrease with the weakening of wind anomalies ().
Notably, when positive rainfall anomalies increase over the southern South China Sea, negative rainfall anomalies develop over midlatitude Asia with large anomalous northerly winds in between (), consistent with Wu (Citation2016). Thus, there is anomalous convergence and upward movement and anomalous divergence and downward movement over the tropics and midlatitudes, respectively. This indicates there may be an anomalous vertical circulation in the meridional direction. To confirm this, we analyze the divergent component of anomalous meridional wind and anomalous vertical velocity at pressure levels. presents the evolution of the composite anomalous meridional circulation along 100°–117.5°E for the southward propagation cases.
Figure 4. Composite anomalies of divergent meridional wind (units: m s−1) and vertical p-velocity (units: 0.01 Pa s−1) (vectors) at pressure levels along 100°E–117.5°E for southward propagation cases on the 9–29-day time scale during NDJFM from 1996/97 to 2014/15. Shading denotes vertical p-velocity with sign reversed.
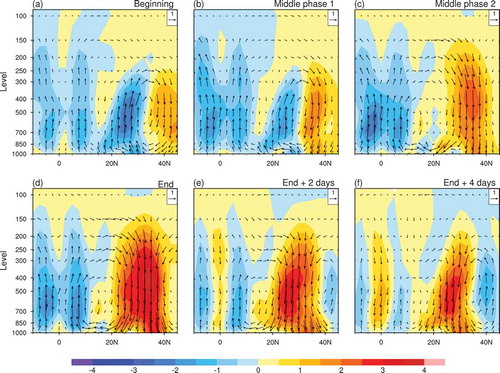
In the beginning phase, an anomalous vertical cell is present between 20°N and 45°N ()). Both anomalous descent in the north and anomalous northerly wind at the lower level intensify and move southward in the following phases (). In the end phase, anomalous downward motion reaches a peak, with the center along 30°–35°N ()). Following the southward movement of anomalous lower-level northerly winds, anomalous upward motion near the equator intensifies, forming an anomalous overturning cell between the tropics and midlatitudes. In the following days, anomalous vertical motion in the tropics becomes weak and anomalous downward motion in the subtropics weakens, accompanied by the appearance of an anomalous vertical cell of opposite direction to the north ().
We also examine the intraseasonal rainfall variations in the 20 eastward propagation cases during 1996/97 to 2014/15. The composite rainfall anomalies appear weaker and have smaller spatial coverage compared to the southward propagation cases. Positive rainfall anomalies are confined to east of the Philippines in middle phase 2 through 2 days after the end phase, and the northwestern South China Sea in the end phase to 4 days after (figure not shown). Both are associated with easterly wind anomalies.
5. Summary and discussion
The present study reveals that the 9–29-day intraseasonal wind signal over midlatitude East Asia in boreal winter can propagate both eastward and southward. In eastward propagation cases, the low-level wind signal is characterized by an eastward migration of anomalous cyclones and anticyclones along the midlatitudes. The average eastward moving speed is about 3–4° of longitude per day, based on composite analysis. In southward propagation cases, the midlatitude anomalous cyclones and anticyclones weaken and anomalous meridional winds move southward from the midlatitudes and reach the equatorial region. In addition to the eastward and southward propagation dominant cases, there are over 20 cases when southward propagation of northerly winds is accompanied by an eastward propagation component (not shown).
The southward propagating meridional wind anomalies induce prominent rainfall anomalies in the equatorial region. When anomalous meridional winds cover the South China Sea, opposite anomalous lower-level convergence/divergence and rainfall anomalies form over the southern South China Sea and eastern China–Japan region. An anomalous vertical cell is established between the tropics and midlatitudes, which plays an important role in linking the intraseasonal rainfall variations between the southern South China Sea and midlatitude East Asia.
There are two issues relevant to the present study worthy of investigation in the future. The first is what determines the propagation direction of intraseasonal wind anomalies over East Asia. Such change in the propagation direction is related to when intraseasonal wind signals over mid-latitude East Asia may induce intraseasonal tropical rainfall anomalies. The other issue is whether intraseasonal variations over the tropics may affect those over midlatitude East Asia. The present study focuses on the impacts of midlatitude intraseasonal wind variations on tropical rainfall anomalies. It is possible that the intraseasonal rainfall changes around the Maritime Continent may affect midlatitude Asia through anomalous heating–induced circulation changes, such as meridional overturning circulation between the tropics and midlatitudes.
Acknowledgments
The NCEP-DOE Reanalysis-2 data were obtained from ftp://ftp.cdc.noaa.gov/. The GPCP precipitation data were retrieved from https://climatedataguide.ucar.edu/climate-data/gpcp-daily-global-precipitation-climatology-project.
Disclosure statement
No potential conflict of interest was reported by the authors.
Additional information
Funding
References
- Cao, X., and R. Wu. 2017. “Origins of Intraseasonal Rainfall Variations over the Southern South China Sea in Boreal Winter.” Atmospheric and Oceanic Science Letters 10 (1): 44–50. doi:10.1080/16742834.2017.1232584.
- Cao, X., and R. Wu. 2018. “Origins and Interrelationship of Intraseasonal Rainfall Variations around the Maritime Continent during Boreal Winter.” Theoretical and Applied Climatology 132 (1–2): 543–554. doi:10.1007/s00704-017-2106-9.
- He, J., H. Lin, and Z. Wu. 2011. “Another Look at Influences of the Madden-Julian Oscillation on the Wintertime East Asian Weather.” Journal of Geophysical Research 116: D03109. doi:10.1029/2010JD014787.
- Huffman, G. J., R. F. Adler, M. M. Morrissey, S. Curtis, R. Joyce, B. McGavock, and J. Susskind. 2001. “Global Precipitation at One-Degree Daily Resolution from Multi-Satellite Observations.” Journal of Hydrometeorology 2 (1): 36–50. doi:10.1175/1525-7541(2001)002<0036:GPAODD>2.0.CO;2.
- Jeong, J.-H., C.-H. Ho, B.-M. Kim, and W.-T. Kwon. 2005. “Influence of the Madden-Julian Oscillation on Wintertime Surface Air Temperature and Cold Surges in East Asia.” Journal of Geophysical Research 110: D11104. doi:10.1029/2004JD005408.
- Jeong, J.-H., B.-M. Kim, C.-H. Ho, and Y.-H. Noh. 2008. “Systematic Variation in Wintertime Precipitation in East Asia by MJO-induced Extratropical Vertical Motion.” Journal of Climate 21 (4): 788–801. doi:10.1175/2007JCLI1801.1.
- Kanamitsu, M., W. Ebisuzaki, J. Woollen, S. Yang, J. J. Hnilo, M. Fiorino, and G. L. Potter. 2002. “NCEP-DOE AMIP-II Reanalysis (R-2).” Bulletin of the American Meteorological Society 83 (11): 1631–1643. doi:10.1175/BAMS-83-11-1631.
- Park, T.-W., C.-H. Ho, S. Yang, and J.-H. Jeong. 2010. “Influences of Arctic Oscillation and Madden-Julian Oscillation on Cold Surges and Heavy Snowfalls over Korea: A Case Study for the Winter of 2009–2010.” Journal of Geophysical Research: Atmospheres 115: D23122. doi:10.1029/2010JD014794.
- Song, L., L. Wang, W. Chen, and Y. Zhang. 2016. “Intraseasonal Variation of the Strength of the East Asian Trough and Its Climatic Impacts in Boreal Winter.” Journal of Climate 29 (7): 2557–2577. doi:10.1175/JCLI-D-14-00834.1.
- Song, L., and R. Wu. 2017. “Processes for Occurrence of Strong Cold Events over Eastern China.” Journal of Climate 30 (22): 9247–9266. doi:10.1175/JCLI-D-16-0857.1.
- Song, L., R. Wu, and Y. Jiao. 2018. “Relative Contributions of Synoptic and Intraseasonal Variations to Strong Cold Events over Eastern China.” Climate Dynamics 50 (11–12): 4619–4634. doi:10.1007/s00382-017-3894-4.
- Wu, R. 2016. “Coupled Intraseasonal Variations in the East Asian Winter Monsoon and the South China Sea-Western North Pacific SST in Boreal Winter.” Climate Dynamics 47 (7–8): 2039–2057. doi:10.1007/s00382-015-2949-7.
- Wu, R., and Z. Chen. 2015. “Intraseasonal SST Variations in the South China Sea during Boreal Winter and Impacts of the East Asian Winter Monsoon.” Journal of Geophysical Research 120 (12): 5863–5878. doi:10.1002/2015JD023368.
- Yang, S., and T. Li. 2016. “Intraseasonal Variability of Air Temperature over the Mid-High Latitude Eurasia in Boreal Winter.” Climate Dynamics 47 (7–8): 2155–2175. doi:10.1007/s00382-015-2956-8.
- Yao, S., Q. Sun, Q. Huang, and P. Chu. 2016. “The 10–30-Day Intraseasonal Variation of the East Asian Winter Monsoon: The Temperature Mode.” Dynamics of Atmospheres and Oceans 75: 91–101. doi:10.1016/j.dynatmoce.2016.07.001.
- Ye, K.-H., and R. Wu. 2015. “Contrast of Local Air-Sea Relationship between 10–20-Day and 30–60-Day Intraseasonal Oscillations during May-September over the South China Sea and the Western North Pacific.” Climate Dynamics 45 (11–12): 3441–3459. doi:10.1007/s00382-015-2549-6.