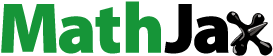
ABSTRACT
Ammonia (NH3) volatilized from agricultural production and its secondary aerosols contribute greatly to air pollution. Different long-term crop straw management practices may significantly affect the soil fertility and soil nitrogen cycle, however, the effect on NH3 volatilization has not been well studied. Therefore, a one-year field experiment was conducted to evaluate the effect of straw incorporation on NH3 volatilization from subtropical calcareous agricultural soil from a longterm perspective, including four treatments: synthetic fertilizer (CK); synthetic fertilizer incorporation with 100% or 50% of the previous season’s crop straw (SI1 and SI2, respectively); and synthetic fertilizer incorporation with 50% burned crop straw (SI2B). Soil NH3 volatilizations were monitored through a wheat–maize rotation year by using a dynamic chamber method. The results demonstrated that NH3 volatilization primarily occurred within 38 days and 7–10 days following nitrogen fertilization events for the wheat and maize seasons, respectively. Different crop straw management practices mainly impacted the NH3 flux of the basal fertilization rather than the topdressing fertilization; long-term crop straw incorporation effectively lowered NH3 loss (35.1% for SI1 and 16.1% for SI2 compared to CK; and the inhibiting effect increased with increasing straw amount, possibly contributed by the high straw carbon/nitrogen ratio, and enhanced microbial activity, which contributed to inorganic nitrogen immobilization and lower ammonium content in the topsoil. However, SI2B significantly increased (29.9%) the annual NH3 flux compared with SI2, indicating that long-term 100% straw incorporation could be a promising straw management practice for mitigating NH3 loss and increasing soil fertility.
Graphical abstract
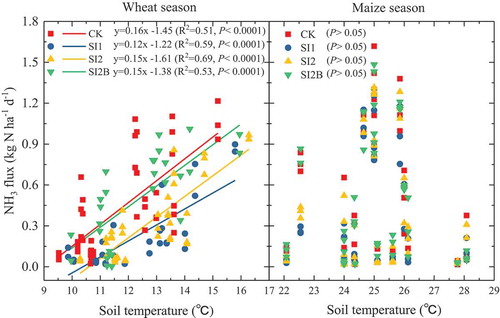
摘要
长期秸秆还田或秸秆焚烧会显著影响土壤肥力及土壤氮素循环, 但该措施对土壤氨挥发的影响仍尚不明确。本研究利用秸秆还田长期定位试验小区, 研究了无秸秆配施 (CK), 配施100%或50%秸秆 (SI1, SI2) 和配施50%秸秆焚烧 (SI2B) 对土壤氨挥发的影响。结果表明: 氨挥发在小麦季持续38天, 而玉米季持续7–10天。秸秆还田显著影响混施基肥期的土壤氨挥发而非表施追肥期。与CK相比, SI1和SI2分别降低了35.1%和16.1%的年累积氨排放, 可能因为秸秆的高C/N比及较高的微生物活性促进了无机氮的固定降低土壤NH4+的浓度。SI2B比SI2增加了29.9%的氨排放。因此, 长期合理的秸秆还田可为石灰性旱地土壤氨挥发减排提供选择和依据。
关键词:
1. Introduction
Agricultural production, as the main anthropogenic source of ammonia (NH3) emissions (Bouwman et al. Citation1997), contributes 80%–90% of total emissions (Kang et al. Citation2016). The volatilized NH3 and its secondary inorganic aerosols may account for about 37% of the total PM2.5 (fine particles with a diameter of 2.5 μm or less) mass (Tao et al. Citation2014), and result in serious air pollution (Gu et al. Citation2014). Furthermore, the emitted NH3 can indirectly result in water eutrophication (Vitousek et al. Citation1997), soil acidification (Guo et al. Citation2010), and a reduction in plant diversity (Bobbink et al. Citation2010) through nitrogen (N) deposition (Liu et al. Citation2010). Recent studies have indicated that areas of high NH3 emissions are concentrated in eastern and southwestern China (Kang et al. Citation2016), which are also areas of serious air pollution in China (Yang et al. Citation2011). Particularly, the unique topographic surroundings of the Sichuan Basin are conducive to PM2.5 pollutant accumulation (Tao et al. Citation2014), so it is essential to reduce NH3 fluxes in this area – especially from calcareous arable soil, seen as NH3 volatilization ‘hotspots’.
Straw incorporation, as an environmentally friendly method, is widely used to maintain or increase soil organic carbon (SOC) storage in arable soils (Xia et al. Citation2018; Zhao et al. Citation2018). Because the carbon (C) and N biogeochemical cycles are tightly coupled (Cleveland and Liptzin Citation2007), straw incorporation may also influence N cycle processes in arable soil. Microbial activities in arable soils are generally C limited (Attard et al. Citation2016), and straw incorporation is favorable for the proliferation of microorganisms, increasing the microbial abundance and activity and consequently improving the immobilization of inorganic N by microbes (Marie-Anne et al. Citation2010) and thus decreasing the NH3 volatilization substrate. Furthermore, the physicochemical properties of soil (e.g., pH, soil bulk density, soil aeration, soil water holding capacity, cation exchange capacity (CEC), etc.) are also affected by straw incorporation; in particular, in fields with long-term returning of straw, these factors will also affect NH3 volatilization (Duan and Xiao Citation2000). Studies examining the effect of straw incorporation on NH3 volatilization have reported negative effects (Wang et al. Citation2012; Yan et al. Citation2016; Cao et al. Citation2018; Li et al. Citation2019). However, some studies have reported that straw incorporation can significantly stimulate NH3 volatilization (Wang et al. Citation2012; Sun et al. Citation2018; Xia et al. Citation2018). These opposite effects indicate a high level of variation in N migration and transformation after straw incorporation, which might depend on the quantity and quality (C/N ratio) of crop straw, soil properties (pH, CEC, soil bulk density), and climatic conditions, amongst other factors (Duan and Xiao Citation2000; Ju and Zhang Citation2017). However, few studies on the effects of the amount and method of crop straw incorporation have been conducted, and the NH3 volatilization mechanism is still not adequately understood (Zhou et al. Citation2016). Moreover, from the long-term perspective, the effect of different straw management practices on NH3 volatilization is influenced by both the incorporated crop straw itself and changes in soil physicochemical properties, which respond slowly to different straw management practices. Therefore, long-term straw incorporation experiments are more suitable for comprehensively elucidating the effects of crop straw incorporation on NH3 volatilization, but such experiments have rarely been carried out. Straw burning used to be common practice in China (Qu et al. Citation2012; Tao et al. Citation2014), therefore, it is meaningful to study the long-term effects of straw burning on soil NH3 volatilization, to further estimate historical NH3 emissions.
An experiment was established in a typical wheat–maize rotation system in the subtropical area of China to study the effects of long-term straw incorporation on NH3 volatilization from alkaline soil. Based on that experiment, the objectives of the present study were to (1) quantify the NH3 volatilization dynamics and fluxes from long-term straw-incorporation soil; and (2) evaluate the effects of different straw incorporation quantities and methods on NH3 volatilization.
2. Materials and methods
2.1. Site description and experiment design
The field experiment was located in Yanting Agro-Ecological Station of the Chinese Academy of Sciences (31°16ʹN, 105°28ʹE) in Sichuan Province. The climate is classified as ‘moderate subtropical monsoon’, with annual mean precipitation of 826 mm and average annual air temperature of 17.3°C. The soil properties (0–20 cm) are listed in .
Table 1. Soil pH, total nitrogen (TN), soil organic matter (SOM) content, alkali-hydrolysable nitrogen (AHN), available phosphorus (AP), available potassium (AK), and soil bulk density (BD) of topsoil (0–15 cm) (mean ± SE) for different treatments.
The long-term wheat–maize rotation experiment, initiated in 2004, was based on a random design including four treatments with three replicate plots (25 m × 2 m; slope: 6.5°) as follows: synthetic fertilizer (crop straw removed, CK); synthetic fertilizer incorporation with 100% or 50% of the previous season’s crop straw (SI1 and SI2, respectively); and synthetic fertilizer incorporation with 50% burned crop straw (burned in-situ, SI2B). Before synthetic fertilizer application, the corresponding crop straw (chopped into 3–5-cm pieces) was broadcast evenly and the straw in the SI2B plots was burned to ashes and cooled. Then, ammonium carbonate (130 kg N ha−1 for the wheat season and 90 kg N ha−1 for the maize season), superphosphate (90 kg P2O5 ha−1 (P2O5: phosphorus pentoxide)), and potassium chloride (36 kg K2O ha−1 (K2O: potassium oxide)) were broadcast as basal fertilization to all the plots, followed by mixing with surface soil (15 cm) using a rotary cultivator. Urea was surface-broadcast (60 kg N ha−1) in the maize season as topdressing after heavy rain during the flare opening stage. The mean crop straw C/N ratio used for the wheat season was 82.6 (maize straw), and for the maize season it was 75.0 (wheat straw). The pH of the burned ash was 12.68 for wheat straw and 12.71 for maize straw.
2.2. Measurement of NH3 volatilization
The NH3 volatilization was measured using a dynamic chamber method. The sampling system was composed of a vacuum pump, a cylindrical dynamic chamber (polymethyl methacrylate, with a height of 15 cm and inner diameter of 20 cm), and an acid trap (60 ml 0.05 mol L−1 sulfuric acid solution) to capture volatilized NH3 (Cao et al. Citation2013). The chamber was inserted into the soil (1 cm) and ambient air located at 2.5 m above the soil was pumped to mix the inner air in the chamber, and then the NH3 in the downstream air was trapped by acid. The background NH3 in the ambient air (2.5 m) was also determined, using a sealed chamber without insertion into the soil. The NH4+-N concentration (NH4+: ammonium) in acid solution was measured using a continuous-flow analyzer (AA3 Bran + Luebbe, Norderstedt, Germany). The NH3 volatilization was monitored twice per day (08:00–10:00 and 15:00–17:00) with a 15 L min−1 airflow rate (Hayashi, Nishimura, and Yagi Citation2006). Firstly, the NH3 volatilization sampling frequency was continually measured for half a month following fertilization, and then the frequency was changed to twice per week throughout each season. The daily NH3 volatilization flux was modified based on the equation published by Hayashi, Nishimura, and Yagi (Citation2006):
where F is the daily flux of NH3 volatilization (kg N ha−1 d−1), cs is the NH4+-N concentration trapped in acid solution for soil plots (mg L−1), cb is the NH4+-N concentration trapped in acid solution for background ambient air (2.5 m) (mg L−1), v is the acid solution volume (L), r is the chamber radius (m), and t is the duration of NH3 sampling (h).
The seasonal cumulative NH3 volatilization flux was determined by linear interpolation of the daily fluxes between the two closet days when observations were taken.
2.3. Environmental factors
Daily precipitation and air temperature were observed by an automatic meteorological station near the experimental plot. The soil temperature (5 cm depth) and soil moisture (at 0–6 cm) were simultaneously measured during NH3 volatilization sampling throughout the experimental period by using a TidbiT v2 Temperature Data Logger (Part UTBI-001, Onset Corp., Pocasset, MA, USA) and a portable frequency domain reflectometry (FDR) moisture sensor (MPKit-B Hangzhou Tuopu Instrument Co. Ltd, China), respectively. The soil moisture measured by FDR was converted to water-filled pore space (WFPS) by the equation WFPS = soil volumetric water content/(1 − soil bulk density/2.65) × 100%, in which the assumed soil particle density was 2.65 g cm−3. The soil heterotrophic respiration rate and soil inorganic N (NH4+ and NO3−) dynamic content (NO3−: nitrate) were simultaneously monitored during the NH3 monitoring period. For more details on the materials and methods, please refer to the Supplementary Material.
2.4. Data processing and statistical analysis
Significant differences in means among the treatments were compared by the least significant difference at the P < 0.05 level with one-way ANOVA using the SPSS 19.0 software program (SPSS Inc., 2008). The relationships between NH3 volatilization flux and environmental factors (soil temperature and WFPS) were analyzed using linear regression. Figures were prepared using the Origin 9.4 software (Origin Lab Corporation, Northampton, USA).
3. Results and discussion
3.1. Related variables
Crop straw incorporation is considered a sustainable and environmentally friendly agricultural practice for improving soil biological and physical fertility and enhancing SOC storage (Blair et al. Citation2006). Also, more apparent effects are usually observed in long-term straw incorporation fields. The changes in soil fertility will significantly influence the migration and transformation processes of N following the application of N fertilizer (Wang et al. Citation2015), such as NH3 volatilization etc., and this was further proved by our long-term crop straw incorporation experiment. For example, on average, the SI1 and SI2 treatments significantly increased soil total N (52.1%), soil organic matter content (55.1%), alkali-hydrolysable N (30.6%), and available potassium (70.6%) in the topsoil (0–15 cm) compared with CK. The above indexes in the burned crop straw treatment (SI2B) also showed a tendency to increase; however, no significant changes were observed in soil available phosphorus and soil pH among all the treatments (). The soil bulk density was reduced by 2.8%–7.1% under different straw incorporation treatments (). Similar results have also been observed by others in the same study region (Zhou et al. Citation2014; Wang et al. Citation2015). Moreover, soil heterotrophic respiration, as an important indicator for microbial activity (Liang et al. Citation2018), in our study, on average, enhanced by 41.6% and 20.8% for the SI1 and SI2 treatments, respectively, while it decreased by 14.5% for the SI2B treatment (Figure S1).
3.2. Temporal variations of NH3 volatilization
shows that all the treatments peaked on the first day following ammonium carbonate application in the basal period of both the wheat and maize seasons. The peak value of NH3 flux in the SI1 and SI2 treatments decreased by 18.1% and 11.0% for the wheat season, and significantly decreased by 65.3% and 47.4% for the maize season (P < 0.05), respectively. The NH3 peak value had a decreasing tendency with an increase in the straw incorporation rate, which is inconsistent with the results of Li et al. (Citation2019). Meanwhile, the highest flux was observed on the third day after broadcast topdressing with urea in the maize season (). This lag effect has also been observed in some other studies after urea application, such as in the subtropical sugarcane production system (Dattamudi et al. Citation2016), rice–wheat rotation system (Sun et al. Citation2018), and typical vegetable system (Li Citation2013), mainly due to the time taken for the hydrolysis of urea into ammonium (Proctor, Koenig, and Johnston Citation2010). Proctor, Koenig, and Johnston (Citation2010) reported that the increased urease activity facilitated by straw incorporation could also be a contributing factor to an earlier and higher peak value of NH3 fluxes in a dryland Kentucky bluegrass seed production system; however, our study did not observe this phenomenon in the urea topdressing period. On the contrary, the SI1 and SI2 straw incorporation treatments lowered the peak value of NH3 fluxes by 13.4% and 9.4%, respectively.
Figure 1. Temporal variations of (a) air temperature (AT), soil temperature (ST, 0–5 cm), precipitation, and soil water-filled pore space (WFPS), and (b) daily NH3 flux from different treatments, in the wheat season and maize seasons. Vertical bars indicate standard errors of three spatial replicates. The arrows indicate the time of fertilization.
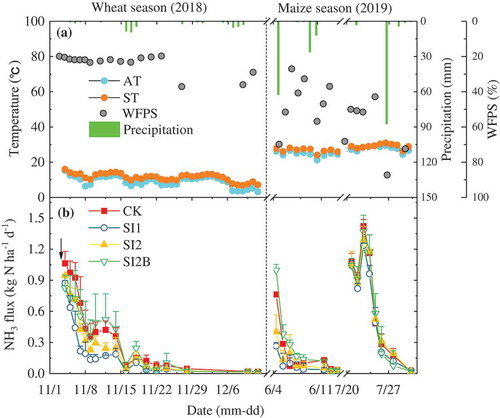
The NH3 volatilization lasted for 38 days in the wheat season following N application, while it only lasted for 8–10 days in the maize season, which might be attributable to the lower soil temperature inhibiting the processes of NH3 diffusion and NH4+ transformation (Sun et al. Citation2018), and which was further demonstrated by the positive correlation between daily NH3 flux and soil temperature (P < 0.0001) () in the wheat season. Moreover, the soil temperature in the maize season was no longer a significant constraining factor (P > 0.05), which favored the process of nitrification (Figure S2) (Clay, Malzer, and Anderson Citation1990) and decreased the substrate (NH4+-N) for NH3 volatilization. The NH3 volatilization characteristics indicated that long-term monitoring for the winter season and high-frequency monitoring for the summer season are needed to increase the flux accuracy.
3.3. Seasonal and annual cumulative NH3 fluxes
As shown in , the annual cumulative NH3 fluxes ranked as: SI2B > CK > SI2 > SI1. The NH3 cumulative flux decreased with an increased straw incorporation rate. Compared with CK, straw incorporation (SI1 and SI2) significantly lowered the NH3 flux by 48.6% and 27.5% (P < 0.05) in the wheat season, respectively. SI1 also evidently decreased the NH3 flux by 48.5% in the basal fertilization of the maize season, and SI2 lowered it by 17.0% in this period. These findings are comparable with a previous study from Northeast China with different straw incorporation rates in the maize season (100% straw incorporation decreased the NH3 flux by 24.6%) (Li et al. Citation2019). However, there was no significant difference among different treatments in the topdressing period (surface-broadcast urea) in the maize season, probably because the poor contact between urea and soil diminished the influence of the soil itself, or the effects of in-season crop straw management practices on NH4+ transformation or immobilization. The mean NH3 flux of the maize basal period was lower than that of the wheat season, probably because of the lower N application rate and higher nitrification rate (Figure S2) in the maize season (Duan and Xiao Citation2000), which ultimately decreased the substrate for NH3 volatilization. The topdressing N rate accounted for 40% of the total N input in the maize season; however, the average NH3 flux of topdressing accounted for 71.4% of the total NH3 flux in maize season (), indicating that surface N application largely stimulates NH3 volatilization (Nett et al. Citation2015).
Table 2. NH3 emission fluxes (mean ± SE) during the wheat and maize seasons.
The highest cumulative NH3 flux was observed in the SI2B treatment in the basal fertilization of both seasons, indicating that straw burning tends to stimulate NH3 loss from ammonium carbonate application as the basal fertilizer. With respect to straw management practices, 50% straw burning and 50% straw incorporation in the basal fertilization increased NH3 losses by 16.5%, on average, and decreased them by 22.3%, compared with CK, respectively (), indicating obvious disadvantages of straw burning in minimizing NH3 loss with respect to straw management. Similarly, Phongpan and Mosier (Citation2003) also found that straw burning could stimulate NH3 loss in two field experiments.
3.4. The effects of long-term crop straw incorporation on NH3 volatilization
Our result showed that, following basal fertilization, 100% and 50% crop straw incorporation led to a 48.6% and 27.5% reduction in NH3 loss for the wheat season, and reduced to a 48.5% and 17.0% NH3 loss for the maize season, respectively. The inhibiting effect of straw incorporation on NH3 volatilization might benefit from the higher soil microbial activity (soil heterotrophic respiration increased by 41.6% for SI1, on average, and by 20.8% for SI2 (Figure S1)), which enhanced NH4+ microbial immobilization from NH4+ to labile organic-N or microbial N following the high C/N ratio of crop straw (ranging from 75.9 to 82.6) application and higher nitrification rate (Figure S2) (Huang et al. Citation2004). As a result, compared with CK, the average NH4+ content for the SI1 and SI2 treatments during the NH3 monitoring period, respectively, decreased by 21.3% and 19.7% for the wheat season, and by 28.0% and 32.6% for the maize season (Figure S2). Recently, Wang et al. (Citation2015) also found that long-term straw incorporation increased the NH4+ immobilization rate by 15.6 times (from 0.9 to 14.4 mg N kg−1 soil d−1) under a wheat–maize rotation system in the same region by using a complex 15N tracing method. Furthermore, a 15N labeling experiment in a paddy system showed that crop straw incorporation decreased NH3 losses by 24%, promoted the immobilized soil inorganic N, and then improved the N mineralization in the later crop growing stage (Cao et al. Citation2018). Moreover, the present study showed long-term crop straw incorporation could improve soil fertility (), which might facilitate the absorption of N by crops, and then indirectly reduce the NH3 loss. A global agroecosystem meta-analysis also indicated that crop straw incorporation can significantly increase the N uptake and N use efficiency of crops, by 10.9% and 15.0% respectively (Xia et al. Citation2018). On the contrary, previous studies have reported that straw incorporation might stimulate NH3 loss from urea fertilizer – for example, in a rice–oilseed rape cropping system (Su et al. Citation2014) and rice–wheat rotation system (Wang et al. Citation2012; Sun et al. Citation2018)—due to the increased urease activity and catalyzed hydrolysis of urea into NH4+. Overall, the comprehensive effect of straw incorporation on NH3 loss should be considered based on the cropping system, length of straw incorporation, fertilizer type, soil properties, and climatic conditions.
3.5. The effects of long-term crop straw burning on NH3 volatilization
Crop straw residue, as a kind of obstacle to farming, was often burned to facilitate crop rotation, which had been proven to be a significant seasonal source of PM2.5 (Qu et al. Citation2012). However, the effects of straw burning on NH3 volatilization were not well studied. Our long-term straw burning experiment showed that straw burning stimulated NH3 loss from the basal fertilization with ammonium carbonate, probably because straw burning decreased the soil microbial activity (Dattamudi et al. Citation2016) to assimilate NH4+ (e.g., the processes of nitrification and immobilization). For example, the soil respiration result showed SI2B decreased soil heterotrophic respiration by 14.5% on average, compared to CK, throughout the NH3 monitoring period (Figure S1). Meanwhile, it increased the top-soil NH4+ content by 28.0% and 54.8%, on average, in the wheat and maize season, respectively, which might also be attributable to this phenomenon. Moreover, the straw ash itself, with high pH (the pH of both wheat and maize straw averaged 12.7), might also contribute to the formation of free NH3 in soil. Phongpan and Mosier (Citation2003) also found that straw burning increased NH3 loss by 5% in a rice cropping system with urea application. Fortunately, the Chinese government has implemented some economic and policy incentives to encourage straw return and prohibit straw burning (Zhao et al. Citation2018), which might have an extra benefit towards reducing NH3 loss.
4. Conclusion
The present study showed that different long-term crop straw management practices significantly affected NH3 volatilization. Crop straw incorporation effectively reduced annual NH3 volatilization losses by 16.1% to 35.1%, and the inhibiting effect increased with the straw application rate, which might be due to enhanced microbial N immobilization and a higher nitrification rate. Meanwhile, straw burning resulted in a 29.9% annual NH3 volatilization increase compared to the same amount of unburned straw incorporation, possibly attributable to reduced microbial activity and the high pH of straw ash. Therefore, proper crop straw management practices could be an environmentally friendly strategy for subtropical calcareous agriculture soils towards reducing NH3 volatilization and increasing soil fertility.
Supplemental Material
Download Zip (500.3 KB)Disclosure Statement
No potential conflict of interest was reported by the authors.
Supplemental Material
Supplemental data for this article can be accessed here
Additional information
Funding
References
- Attard, E., X. Le Roux, X. Charrier, O. Delfosse, N. Guillaumaud, G. Lemaire, and S. Recous. 2016. “Delayed and Asymmetric Responses of Soil C Pools and N Fluxes to Grassland/cropland Conversions.” Soil Biology and Biochemistry 97: 31–39.
- Blair, N., R. D. Faulkner, A. R. Till, and P. R. Poulton. 2006. “Long-term Management Impacts on Soil C, N and Physical Fertility - Part 1: Broadbalk Experiment.” Soil & Tillage Research 91: 30–38.
- Bobbink, R., K. Hicks, J. Galloway, T. Spranger, R. Alkemade, M. Ashmore, M. Bustamante, et al. 2010. “Global Assessment of Nitrogen Deposition Effects on Terrestrial Plant Diversity: A Synthesis.” Ecological Applications 20: 30–59.
- Bouwman, A. F., D. S. Lee, W. A. H. Asman, F. J. Dentener, K. W. VanderHoek, and J. G. J. Olivier. 1997. “A Global High-resolution Emission Inventory for Ammonia.” Global Biogeochemical Cycles 11: 561–587.
- Cao, Y., Y. Tian, B. Yin, and Z. Zhu. 2013. “Assessment of Ammonia Volatilization from Paddy Fields under Crop Management Practices Aimed to Increase Grain Yield and N Efficiency.” Field Crops Research 147: 23–31.
- Cao, Y. S., H. F. Sun, J. N. Zhang, G. F. Chen, H. T. Zhu, S. Zhou, and H. Y. Xiao. 2018. “Effects of Wheat Straw Addition on Dynamics and Fate of Nitrogen Applied to Paddy Soils.” Soil & Tillage Research 178: 92–98.
- Clay, D., G. Malzer, and J. Anderson. 1990. “Ammonia Volatilization from Urea as Influenced by Soil Temperature, Soil Water Content, and Nitrification and Hydrolysis Inhibitors.” Soil Science Society of America Journal 54: 263–266.
- Cleveland, C. C., and D. Liptzin. 2007. “C: N: P Stoichiometry in Soil: Is There a “Redfield Ratio” for the Microbial Biomass?” Biogeochemistry 85: 235–252.
- Dattamudi, S., J. J. Wang, S. K. Dodla, A. Arceneaux, and H. P. Viator. 2016. “Effect of Nitrogen Fertilization and Residue Management Practices on Ammonia Emissions from Subtropical Sugarcane Production.” Atmospheric Environment 139: 122–130.
- Duan, Z. H., and H. L. Xiao. 2000. “Effects of Soil Properties on Ammonia Volatilization.” Soil Science and Plant Nutrition 46: 845–852.
- Gu, B. J., M. A. Sutton, S. X. Chang, Y. Ge, and J. Chang. 2014. “Agricultural Ammonia Emissions Contribute to China’s Urban Air Pollution.” Frontiers in Ecology and the Environment 12: 265–266.
- Guo, J. H., X. J. Liu, Y. Zhang, J. L. Shen, W. X. Han, W. F. Zhang, P. Christie, K. W. T. Goulding, P. M. Vitousek, and F. S. Zhang. 2010. “Significant Acidification in Major Chinese Croplands.” Science 327: 1008–1010.
- Hayashi, K., S. Nishimura, and K. Yagi. 2006. “Ammonia Volatilization from the Surface of a Japanese Paddy Field during Rice Cultivation.” Soil Science and Plant Nutrition 52: 545–555.
- Huang, Y., J. Zou, X. Zheng, Y. Wang, and X. Xu. 2004. “Nitrous Oxide Emissions as Influenced by Amendment of Plant Residues with Different C:N Ratios.” Soil Biology & Biochemistry 36: 973–981.
- Ju, X. T., and C. Zhang. 2017. “Nitrogen Cycling and Environmental Impacts in Upland Agricultural Soils in North China: A Review.” Journal of Integrative Agriculture 16: 2848–2862.
- Kang, Y., M. Liu, Y. Song, X. Huang, H. Yao, X. Cai, H. Zhang, et al. 2016. “High-resolution Ammonia Emissions Inventories in China from 1980 to 2012.” Atmospheric Chemistry and Physics 16: 2043–2058.
- Li, D. J. 2013. “Emissions of NO and NH3 from a Typical Vegetable-Land Soil after the Application of Chemical N Fertilizers in the Pearl River Delta.” PloS One 8: 8.
- Li, J., H. Yang, F. Zhou, X. C. Zhang, J. F. Luo, Y. Li, S. Lindsey, Y. L. Shi, H. B. He, and X. D. Zhang. 2019. “Effects of Maize Residue Return Rate on Nitrogen Transformations and Gaseous Losses in an Arable Soil.” Agricultural Water Management 211: 132–141.
- Liang, G. P., H. J. Wu, A. A. Houssou, D. X. Cai, X. P. Wu, L. L. Gao, B. S. Wang, and S. P. Li. 2018. “Soil Respiration, Glomalin Content, and Enzymatic Activity Response to Straw Application in a Wheat-maize Rotation System.” Journal of Soils and Sediments 18: 697–707.
- Liu, X. J., L. Song, C. E. He, and F. S. Zhang. 2010. “Nitrogen Deposition as an Important Nutrient from the Environment and Its Impact on Ecosystems in China.” Journal of Arid Land 2: 137–143.
- Marie-Anne, D. G., A. T. Classen, H. F. Castro, and C. W. Schadt. 2010. “Labile Soil Carbon Inputs Mediate the Soil Microbial Community Composition and Plant Residue Decomposition Rates.” New Phytologist 188: 1055–1064.
- Nett, L., R. Fuß, H. Flessa, and M. Fink. 2015. “Emissions of Nitrous Oxide and Ammonia from a Sandy Soil following Surface Application and Incorporation of Cauliflower Leaf Residues.” The Journal of Agricultural Science 153: 1341–1352.
- Phongpan, S., and A. R. Mosier. 2003. “Effect of Crop Residue Management on Nitrogen Dynamics and Balance in a Lowland Rice Cropping System.” Nutrient Cycling in Agroecosystems 66: 133–142.
- Proctor, C., R. Koenig, and W. Johnston. 2010. “Potential for Ammonia Volatilization from Urea in Dryland Kentucky Bluegrass Seed Production Systems.” Communications in Soil Science and Plant Analysis 41: 320–331.
- Qu, C. S., B. Li, H. S. Wu, and J. P. Giesy. 2012. “Controlling Air Pollution from Straw Burning in China Calls for Efficient Recycling.” Environmental Science & Technology 46: 7934–7936.
- Su, W., J. W. Lu, W. N. Wang, X. K. Li, T. Ren, and R. H. Cong. 2014. “Influence of Rice Straw Mulching on Seed Yield and Nitrogen Use Efficiency of Winter Oilseed Rape (Brassica Napus L.) In Intensive Rice-oilseed Rape Cropping System.” Field Crops Research 159: 53–61.
- Sun, L. Y., Z. Wu, Y. C. Ma, Y. L. Liu, and Z. Q. Xiong. 2018. “Ammonia Volatilization and Atmospheric N Deposition following Straw and Urea Application from a Rice-wheat Rotation in Southeastern China.” Atmospheric Environment 181: 97–105.
- Tao, J., J. Gao, L. Zhang, R. Zhang, H. Che, Z. Zhang, Z. Lin, J. Jing, J. Cao, and S. C. Hsu. 2014. “PM2.5 Pollution in a Megacity of Southwest China: Source Apportionment and Implication.” Atmospheric Chemistry and Physics 14: 8679–8699.
- Vitousek, P. M., J. D. Aber, R. W. Howarth, G. E. Likens, P. A. Matson, D. W. Schindler, W. H. Schlesinger, and D. Tilman. 1997. “Human Alteration of the Global Nitrogen Cycle: Sources and Consequences.” Ecological Applications 7: 737–750.
- Wang, J., D. J. Wang, G. Zhang, and C. Wang. 2012. “Effect of Wheat Straw Application on Ammonia Volatilization from Urea Applied to a Paddy Field.” Nutrient Cycling in Agroecosystems 94: 73–84.
- Wang, J., B. Zhu, J. Zhang, C. Müller, and Z. Cai. 2015. “Mechanisms of Soil N Dynamics following Long-term Application of Organic Fertilizers to Subtropical Rain-fed Purple Soil in China.” Soil Biology & Biochemistry 91: 222–231.
- Xia, L. L., S. K. Lam, B. Wolf, R. Kiese, D. L. Chen, and K. Butterbach-Bahl. 2018. “Trade-offs between Soil Carbon Sequestration and Reactive Nitrogen Losses under Straw Return in Global Agroecosystems.” Global Change Biology 24: 5919–5932.
- Yan, L., Z. D. Zhang, Y. Chen, Q. Gao, W. X. Lu, and A. M. Abdelrahman. 2016. “Effect of Water and Temperature on Ammonia Volatilization of Maize Straw Returning.” Toxicological and Environmental Chemistry 98: 638–647.
- Yang, F., J. Tan, Q. Zhao, Z. Du, K. He, Y. Ma, F. Duan, G. Chen, and Q. Zhao. 2011. “Characteristics of PM2.5 Speciation in Representative Megacities and across China.” Atmospheric Chemistry and Physics 11: 5207–5219.
- Zhao, Y. C., M. Y. Wang, S. J. Hu, X. D. Zhang, Z. Ouyang, G. L. Zhang, B. A. Huang, et al. 2018. “Economics- and Policy-driven Organic Carbon Input Enhancement Dominates Soil Organic Carbon Accumulation in Chinese Croplands.” Proceedings of the National Academy of Sciences of the United States of America 115: 4045–4050.
- Zhou, F., P. Ciais, K. Hayashi, J. Galloway, D. G. Kim, C. L. Yang, S. Y. Li, B. Liu, Z. Y. Shang, and S. S. Gao. 2016. “Re-estimating NH3 Emissions from Chinese Cropland by a New Nonlinear Model.” Environmental Science & Technology 50: 564–572.
- Zhou, M., B. Zhu, N. Brüggemann, J. Bergmann, Y. Wang, and K. Butterbach-Bahl. 2014. “N2O and CH4 Emissions, and NO3− Leaching on a Crop Yield Basis from a Subtropical Rain-fed Wheat–Maize Rotation in Response to Different Types of Nitrogen Fertilizer.” Ecosystems 17: 286–301.