ABSTRACT
The metal oxide surface-functionalized CNTs have attracted the interest of many researchers worldwide because of their different and feast important applications. In this investigation, two different hierarchical metal oxide nanoparticles Zinc oxide (ZnO) and Cobalt oxide (Co3O4) have been loaded on the external surface of multi-walled carbon nano tubes (MWCNTs) following the hydrothermal process by using the cetyltrimethylammonium bromide (CTAB) surfactant. To assess the quality of preparation, the prepared nano composites were verified by utilizing various techniques, including scanning electron microscopy (SEM), X-ray diffraction (XRD), high-resolution transmission electron microscopy (HRTEM), Fourier transform infrared spectroscopy (FTIR), and Raman spectroscopy. It was proven by the obtained results that, ZnO and Co3O4 are uniformly and homogenously loaded on the surface of MWCNTs, as presented in the SEM and HR-TEM images. Also, The FTIR confirms the presence of absorption bands related to the presence of CNTs, ZnO and Co3O4 nano materials. in addition, The HRTEM imgaes and corresponding SAED demonstrate the presence of nano particles (ZnO and Co3O4) on the external surface of MWCNTs. furthermore, ZnO particles have a hexagonal platelet structure, and their thickness increases as the amount of CTAB increases. Moreover, the XRD diffraction pattern confirms the crystalline structure of the loaded nanoparticles.
Introduction
The integration of carbon nanomaterials in numerous applications has increased rapidly over the previous and running decades. The dedication of carbon nanomaterials to these applications comes from their outstanding physical, chemical, and biological properties [Citation1–5]. The carbon nanomaterials family comprises different members differing in their fundamental properties while attaining the same building carbon [Citation6,Citation7]. The most studied carbon nanomaterials are graphene and carbon nanotubes [Citation8]. Graphene is preliminary composed of 2D honeycomb carbon atoms [Citation9,Citation10], while carbon nanotubes are made up of carbon atoms in the form of hollow cylinders [Citation11–13]. Focusing on carbon nanotubes, three main types can be obtained based on the rolling directions of the tubes. These types are chiral, zigzag, and armchair. Another classification could be based on the number of fitted tubes as single-wall or multi-wall [Citation14,Citation15]. However, the outstanding properties of carbon nanomaterials have some drawbacks that are related to their surface properties and functionality. On the other hand, metal oxide nanoparticles have fascinating functional properties that serve in many applications, while their conductivity represents the obstacle against these amazing properties [Citation16–19]. Nanostructured metal oxide exhibited different morphological structures, such as nanowires, nanotubes, spheres, 2D platelets, and hierarchical structures [Citation20]. The combination between these two types of materials will in fact result in a modified functional material that will be used effectively in such recent technological applications [Citation21,Citation22]. Different types of materials have been attached externally on the surface of CNTs such as polymer, ceramics, metals, metal oxides, and even biological molecules [Citation23]. The attachment of metal oxide nanostructures over the external surface of CNTs can make such huge changes due to the remarkable changes of individual components [Citation24–27]. Different methodologies, including physical and chemical routes, were proposed to attach such tiny particles [Citation28]. The most forward techniques are the wet chemistry-based methods [Citation29]. Hierarchically structured materials are characterized by low density, high surface areas, and interlinked hierarchical porosity at various lengths that exhibit outstanding properties for ion and electron transport, light harvesting, mass loading, and diffusion [Citation30]. The combination of hierarchical metal oxide and carbon nanotubes is a brilliant strategy to obtain a composite material with functional properties for smart applications. The aim of this work is to load different hierarchical metal oxide nanoparticles over the external surface of MWCNTs using wet chemical methods. The loading process was executed through two successive steps, including co-precipitation and hydrothermal. The first section of this work is directed to optimize the structure of nano materials and investigate the effect of surfactant on the properties of the resultant nano metal oxides. While the second part was directed to attach the optimized metal oxide on the outer surface of CNTs. The obtained structures were characterized by different techniques, including scanning electron microscopy (SEM), X-ray diffraction (XRD), high-resolution transmission electron microscopy (HRTEM), Fourier transform infrared spectroscopy (FTIR), and Raman. The crystal structure of the composite materials was verified through the XRD measurements, where sharp and defined peaks were recognized indicating the crystalline structure of the prepared materials. The morphological feature examinations conducted by SEM and HRTEM give direct evidence for the presence of hierarchical metal oxides (ZnO and Co3O4) over the external surface of MWCNTs. The methods conducted give a promising route for attaching hierarchical metal oxide over external surfaces of carbon nano-based materials.
Experimental
Materials
Zn(NO3)2.6 H2O, CTAB, cobalt nitrate, and potassium hydroxide were purchased from Sigma Aldrich Germany. The utilized chemicals were used as they were received without any further purification or treatment. Carbon nanotubes were synthesized using atmospheric chemical vapor deposition (APCVD). The MWCNTs were synthesized at Housing and Building National Research Center (HBRC), Giza, Egypt. More detailed information regarding the synthesizing method can be found in our published articles [Citation26,Citation31,Citation32].
Hierarchical MO/MWCNTs synthesizing
The synthesis of MO/MWCNTs has been executed through two successive steps: first, seeding by co-precipitation, followed by hydrothermal conversion-growth method. ZnO nanoparticles were used as a model for MO to optimize all synthesizing parameters, and then the same procedures have been traced for the other composite. 0.01 M of ZnNO3 was dissolved in 50 ml of distilled water under continuous stirrer (at 70°C) until a clear solution was obtained. Different concentrations of 1%, 2%, and 3% (wt/wt) CTAB were dissolved in 50 ml of distilled water under vigorous and continuous stirring (70°C). The CTAB solution was mixed with ZnNO3 solution while continuing to stir and heat for about 30 min. Ten milliliters of 5 M of KOH dissolved in 20 ml of distilled water, then added dropwise to the above-mixed solution until pH reaches 8, while keeping the stirring for additional 1 h to ensure good mixing. After this, upload this solution into a Teflon autoclave and maintain the temperature to 100°C for 24 h. The obtained precipitates were collected by centrifuge and washed several times until neutralization. After optimizing all synthesizing conditions, the same procedures were repeated in the presence of MWCNTs, which have been dispersed in a metal salt solution via ultrasonication for 30 min. For the other composite (MWCNTs-Co3O4), the same procedures were followed while keeping the same molar ratio. The schematic representation of the synthesizing procedures is presented in .
Characterization techniques
Through the current study several characterization techniques were used to investigate the crystal structure and morphological features of the resultant synthesized materials. These techniques include XRD (Malvern Panalytical Empyrean 3 diffractometer), FTIR (Vertex 70, Bruker), SEM (thermo scientific, operated at 20 kV), HR-TEM (JEM-2100 operated at 200 kV), and Raman (Witec Alpha 300 RA, 514 nm excitation).
Results and discussion
Hierarchical ZnO optimization
The composition of the Hierarchical ZnO is verified through using XRD measurements. The main objective of this test is to identify the formation of the required phase and ensure the purity of resultant structure. The XRD patterns of the ZnO nanoparticles before and after calcination are depicted in . The diffraction pattern of the ZnO sample exhibited characteristics peaks at of ZnO nanoparticles in addition to a less intense peak at about 17° that belonging to the carbon residue. After calcination at 350°C, the peak at 17° was diminished. The XRD pattern of the ZnO nano particles demonstrates a well-defined and sharp peak without any noticeable traces of froing inclusions.
SEM analysis was conducted to investigate the effects of CTAB concentration on the morphology (particle size and shape) of the synthesized ZnO NPs. presents the SEM images of ZnO nanoparticles produced at 1%, 2%, and 3% of the CTAB.
Figure 3. SEM images for ZnO assisted CTAB synthesised at various concentrations of (a) 1%, (b) 2%, and (C) 3%.
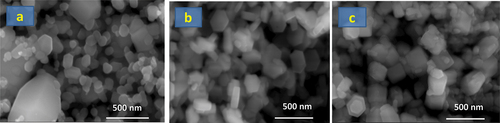
It is clearly seen from SEM images that the CTAB-mediated ZnO NPs exhibit a hexagonal crystalline structure. Although there is no remarkable change in crystalline shape, the average crystallite sizes have been found to range from 75 nm to 200 nm, depending on different lattice planes. The thickness of the produced CTAB-ZnO NPs becomes larger with the increasing CTAB concentration; a remarkable increase in the crystalline size is obvious. The 3% CTAB-mediated ZnO showed a non-uniform grain distribution of NPs. The increased aggregation of ZnO nanoparticles as a result of the increased electrostatic repulsive force between ZnO nanoparticles and CTAB molecules at higher concentrations of CTAB solution is responsible for the thicker crystals [Citation33]. In terms of the uniform morphology and lesser ZnO platelet thickness, the 1% CTAB can be considered as the best concentration. The SEM image of ZnO nano platelets after calcination at 350°C is shown in .
Figure 4. SEM image for ZnO assisted CTAB synthesised at concentration of 1% after calcination at 350°C.
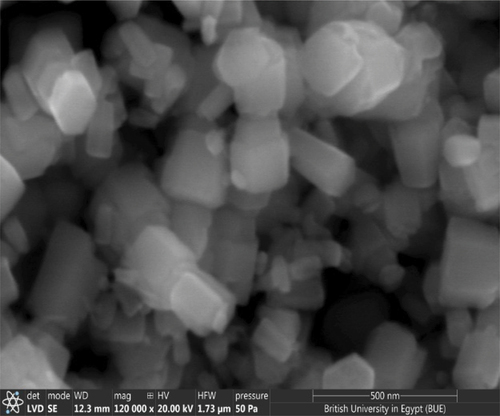
The SEM image of 1% CTAB-mediated ZnO after calcination at 350°C is shown in . It was observed that the calcination of ZnO does not change/alter the morphological features of the synthesized ZnO NPs. This will be confirmed from XRD measurements.
The hierarchical CNTs/metal oxide
The optimized synthesizing parameters, including CTAB ratio and calcination temperature, have been applied to the synthesized nanocomposite. The XRD patterns of CNTs-ZnO and CNTs-Co3O4 are displayed in . The CNTs-Co3O4 nanocomposite demonstrates sharp and intense diffraction peaks at 19°, 31°, 37°, 44.8°, 59.5°, and 65.4°, corresponding to (111), (220), (311), (400), and (511), respectively. The diffraction peaks of ZnO coincide with card No. 01-075-9742 of Wurtzite ZnO nanoparticles.
presents SEM images of CTAB-assisted ZnO-CNT and Co3O4-CNT nanocomposites. All images revealed the typical wire morphology of CNTs with the polyhedral particles of ZnO and Co3O4. CNT wires are better displayed in . It can be observed that CNT wires are bound with ZnO agglomerates; however, the Co3O4 aggregates have their surface coated with CNT wires, as is clear from .
shows the HR-TEM micrograph associated with the selected area electron diffraction (SAED) patterns of CNT-ZnO and CNT-Co3O4 nanocomposites. It could be clearly seen that ZnO and Co3O4 NPs were successfully adsorbed on the CNT. The developed composites possessed d-spacing values ranging between 0.15 and 0.21 nm. The CNT-Co3O4 nanocomposite exhibited the smallest d-spacing. The SAED patterns for both CNT-ZnO and CNT-Co3O4 nanocomposites showed clear diffraction spots, referring to a high quality and hexagonal structure of single crystalline nanoplatelets. While the Co3O4-modified CNT showed a concentric ring-like structure that reveals its polycrystalline nature.
Figure 7. HR-TEM micrograph and the selected area electron diffraction (SAED) patterns of (a) CNT-ZnO, (b) CNT-SnO2, and (c) CNT-Co3O4 nanocomposites.
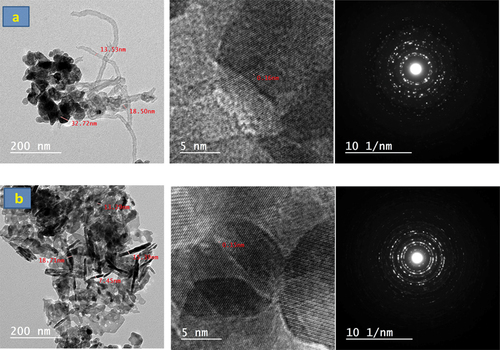
It is known that Raman spectroscopy is a highly sensitive analyzing tool used to study the ordered/disordered crystal structure of carbon nano-materials [Citation38].
The Raman spectra of the hydrothermally prepared CNTs/ZnO and CNTs/Co3O4 nano composites obtained in the range of 50–3500 cm−1 are graphically presented in .
It can be observed from the Raman spectrum the presence of the MWCNTs characteristic bands, these are D (occurred due to the degree of disorder of a structure) at 1345 cm−1, G (caused by the degree of graphitization of the tubes) at 1576 cm−1 and G‵ (originated from stresses) at 2683 cm−1 [Citation39,Citation40]. Furthermore, the intensity ratio (ID/IG) which measures the degree of carbon material crystallinity was calculated to be 0.85. From CNTs/ZnO spectrum the D, G and G‵ bands characterizing MWCNTs are observed at 1345 cm−1, 1576 cm−1 and 2688 cm−1, respectively. In addition to ZnO characterizing bands 2E2 (low), E2 (high)-E2 (low) emerged modes originated from second-order Raman scattering process, E2 (high) assigned to vibration of oxygen, A1 (LO) also related to second-order Raman scattering and two phonon mode (2-LO) which located at 193 cm−1, 321 cm−1, 428 cm−1, 572 cm−1 and 1123 cm−1, respectively [Citation41,Citation42]. Furthermore, the (ID/IG) intensity ratio was found to be the same as that of CNTs/SnO2 spectrum.
Finally, from CNTs/Co3O4 spectrum the A1g, Eg, and F2g vibration modes of Co3O4 are observed, which are related to the combined vibrations of tetrahedral site and octahedral oxygen motions located at 667 cm−1, 508 cm−1, 464 cm−1 and 188 cm−1, respectively [Citation43,Citation44]. While D, G and G‵ bands characterizing MWCNTs are absent which may be because of the surface of CNTs are completely and homogenously covered by Co3O4 particles.
Conclusion
In this manuscript, the loading of different metal oxide nanoparticles has been done on the external surface of MWCNTs via hydrothermal route. The effect of CTAB surfactant on the morphological features of ZnO nanoparticles was investigated. It was demonstrated that the prepared ZnO nanoparticles have a hexagonal platelet structure. Moreover, the thickness of the ZnO platelet increases as the amount of CTAB increases. The XRD diffraction pattern confirms the crystalline structure of the loaded nanoparticles (ZnO and Co3O4). The morphological examination using SEM and HRTEM proves the successful loading of nanoparticles on the external surface of MWCNTs. The well-known characteristic bands of MWCNTs, ZnO, and Co3O4 are clearly observed in the Raman spectra. Finally, the obtained FTIR results confirm the presence of the absorption band of MWCNTs and the loaded metal oxide on their surface. The MO/CNTs nano composites can be directed toward multiple applications including sensors, photo catalysis, composite materials, solar cell, mechatronics, electronics, biological applications, hydrogen storage and many other applications.
Acknowledgments
The authors would like to thank the science, technology, and innovation funding authority (STDF) for financial funding this work through the project ID:37105.
Disclosure statement
No potential conflict of interest was reported by the author(s).
Additional information
Funding
References
- El-Feky MS, El-Khodary SA, Morsy M Optimization of Hybrid Cement Composite with Carbon Nanotubes and Nano Silica Using Response Surface Design . Egypt J Chem. 2019;62(1):57–67. doi: 10.21608/ejchem.2019.12771.1797
- Morsy M, Darwish AG, Mokhtar MM, et al. J Mater Sci. 2022.
- Morsy M, Abdel-Salam AI, Mostafa M, et al. Micro And Nano Eng. 2022;17:100163. doi: 10.1016/j.mne.2022.100163
- Pathaare Y, Reddy AM, Sangrulkar P, et al. Carbon hybrid nano-architectures as an efficient electrode material for supercapacitor applications. Hybrid Adv. 2023;3:100041. doi: 10.1016/j.hybadv.2023.100041
- Siddiqui MTH, Nizamuddin S, Baloch HA, et al. Fabrication of advance magnetic carbon nano-materials and their potential applications: a review. J Environ Chem Eng. 2019;7(1):102812. doi: 10.1016/j.jece.2018.102812
- Porto LS, Silva DN, de Oliveira AEF, et al. 2019; 38(3): doi: 10.1515/revac-2019-0017
- Gaur M, Misra C, Yadav AB, et al., Materials (Basel, Switzerland) 14, (2021). 20 5978 doi: 10.3390/ma14205978
- Zaytseva O, Neumann G, Chemical and biological technologies in agriculture 3, 17 (2016). 1 10.1186/s40538-016-0070-8
- Moustafa H, Isawi H, M S. Abd El Wahab. Environ Nanotechnol, Monit Manage. 2022;18:100696. doi:10.1016/j.enmm.2022.100696
- Zhou Y, Chen R, Gao Z, et al. Greenly growing carbon nanotubes on graphene for high-performance lithium–sulfur batteries. Mater Today Energy. 2023;37:101389. doi: 10.1016/j.mtener.2023.101389
- Yousf N, Elzwawy A, Ouda E, et al. ECS Journal Solid State Sci And Tech. 2021.
- El-Feky MS, El-Khodary SA, Morsy M. Egypt J Chem. 2019;62(1):7–9. doi: 10.21608/ejchem.2019.12771.1797
- Aqel A, El-Nour KMMA, Ammar RAA, et al. Arabian J Chem. 2012;5(1):1. doi: 10.1016/j.arabjc.2010.08.022
- Sidhu JS, Misra A, Bhardwaj A, Materials Today: Proceedings (2023).
- De Luca P, Siciliano C, Nagy JB, et al. Chem Eng Res Des. 2023;197:74. doi: 10.1016/j.cherd.2023.07.016
- Morsy M, Abdel-Salam AI, Rayan DA, et al. Oil/Water separation and functionality of smart carbon nanotube–titania nanotube composite. J Nanopart Res. 2022;24(11):226. doi: 10.1007/s11051-022-05597-y
- Chavali MS, Nikolova MP, SN applied sciences 1, 607 (2019). 6 doi: 10.1007/s42452-019-0592-3
- Alhalili Z, Molecules (Basel, Switzerland) 28, (2023). 7 3086 doi: 10.3390/molecules28073086
- Joshi N, Pandey DK, Mistry BG, et al. edited by. D. K. Singh, S. Singh, and P. Singh. Singapore: Springer Nature Singapore; 2023pp. 103–144
- Negrescu AM, Killian MS, Raghu SNV, et al. J Funct Biomater. 2022;13(4):274. doi: 10.3390/jfb13040274
- Wu F, Wang C, Hu H-Y, et al. One-step synthesis of hierarchical metal oxide nanosheet/carbon nanotube composites by chemical vapor deposition. J Mater Sci. 2019;54(2):1291. doi: 10.1007/s10853-018-2889-9
- Lee JA, Lee WJ, Lim J et al. N-Dopant-Mediated Growth of Metal Oxide Nanoparticles on Carbon Nanotubes. Nanomaterials. 2021;11(8). doi:10.3390/nano11081882
- Moustafa H, Darwish NA, Youssef AM. Facile coating of carbon nanotubes by different resins for enhancing mechanical, electrical properties and adhesion strength of NR/Nylon 66 systems. J Adhes. 2021;97(9):801. doi: 10.1080/00218464.2019.1707674
- Morsy M, Yahia IS, Zahran HY, et al. Hydrothermal synthesis of CNTs/Co3O4@rGO Mesopours nanocomposite as a room temperature gas sensor for VOCs. J Inorg Organomet Polym Mater. 2019;29(2):416. doi: 10.1007/s10904-018-1011-8
- Moustafa H, Ahmed EM, Morsy M. Bio-based antibacterial packaging from decorated bagasse papers with natural rosin and synthesised GO-Ag nanoparticles. Mater Technol. 2022;37(13):2766. doi: 10.1080/10667857.2022.2074939
- Morsy M, Yahia IS, Zahran HY, et al. Low cost alcoholic breath sensor based on SnO2 modified with CNTs and graphene. J Korean Phys Soc. 2018;73(10):1437. doi: 10.3938/jkps.73.1437
- Mallakpour S, Khadem E. Carbon nanotube–metal oxide nanocomposites: fabrication, properties and applications. Chem Eng J. 2016;302:344. doi: 10.1016/j.cej.2016.05.038
- Hu Y, Guo C. edited by. D. M. Naraghi. IntechOpen, Rijeka, 2011 carbon nanotubes and carbon nanotubes/metal oxide heterostructure: synthesis, characterization and electrochemical property. 1. doi:10.5772/16463
- Aseena S, Abraham N, Babu VS. Synthesis of CNT based nanocomposites and their application as photoanode material for improved efficiency in DSSC. Ceram Int. 2020;46(18):28355. doi: 10.1016/j.ceramint.2020.07.339
- Hassan IU, Salim H, Naikoo GA, et al. A review on recent advances in hierarchically porous metal and metal oxide nanostructures as electrode materials for supercapacitors and non-enzymatic glucose sensors. J Saudi Chem Soc. 2021;25(5):101228. doi: 10.1016/j.jscs.2021.101228
- Morsy M, Helal M, El-Okr M, et al. Spectrochimica acta - part A: molecular and biomolecular spectroscopy. 2014;132:594. doi: 10.1016/j.saa.2014.04.122
- Morsy M, Yahia IS, Zahran HY, et al. Portable and battery operated ammonia gas sensor based on CNTs/rGo/zno nanocomposite. J Electron Mater. 2019;48(11):7328. doi: 10.1007/s11664-019-07550-7
- Abdi S, Dorranian D. Effect of CTAB concentration on the properties of ZnO nanoparticles produced by laser ablation method in CTAB solution. Opt Laser Technol. 2018;108:372. doi: 10.1016/j.optlastec.2018.07.009
- Yudianti R, Onggo H, Sudirman S et al. Analysis of Functional Group Sited on Multi-Wall Carbon Nanotube Surface The Open Materials Science Journal . 2010;5:242–247 .
- Ahmaruzzaman M, Mohanta D, Nath A. Environmentally benign fabrication of SnO2-CNT nanohybrids and their multifunctional efficiency as an adsorbent, catalyst and antimicrobial agent for water decontamination. Sci Rep. 2019;9(1):12935. doi: 10.1038/s41598-019-49181-2
- Alias MFA, Abd – Alsada AS, Journal of Physics: Conference Series 2114, (2021).
- Mohammadnavaz A, Beitollahi H, Modiri S. Electro-catalytic determination of l-Cysteine using multi walled carbon nanotubes-Co3O4 nanocomposite/benzoylferrocene/ionic liquid modified carbon paste electrode. Inorganica Chimica Acta. 2023;548:121340. doi: 10.1016/j.ica.2022.121340
- Singh BP, Samal S, Nayak S, et al. Surf Coat Technol. 2011;206(6):1319. doi: 10.1016/j.surfcoat.2011.08.054
- Diéguez A, Romano-Rodríguez A, Vilà A, et al. J Appl Phys. 2001;90(3):1550. doi: 10.1063/1.1385573
- Mariammal RN, Ramachandran K, Renganathan B, et al. Sensors and actuators B: chemical. 2012;169:199. doi: 10.1016/j.snb.2012.04.067
- Song Y, Zhang S, Zhang C, et al. Crystals. 2019;9(8):395. doi: 10.3390/cryst9080395
- Sharma A, Singh BP, Dhar S, et al. Effect of surface groups on the luminescence property of ZnO nanoparticles synthesized by sol–gel route. Surf Sci. 2012;606(3–4):L13. doi: 10.1016/j.susc.2011.09.006
- Diallo A, Beye AC, Doyle TB, et al. Green synthesis of Co3 O4 nanoparticles via aspalathus linearis: physical properties. Green Chem Lett Rev. 2015;8(3–4):30–36. doi: 10.1080/17518253.2015.1082646
- Papers F, Morsy M, Secondary CA, et al., (n.d.).