ABSTRACT
The potential of fused filament fabrication (FFF) for the administration of active pharmaceutical compounds is a recent approach to develop complex and custom-made drug delivery systems (DDSs). However, the FFF technology is characterized by certain limitations, which are associated with the nature of the process, i.e., the required mechanical properties of the feedstock, as well as the thermal stability of the incorporated polymers, excipients and active compounds. Thus, hybrid DDSs have been recently introduced, to overcome these boundaries. The concept of these systems is defined by the effective coupling of FFF with conventional manufacturing technologies, as a novel pathway to expand the available pool of raw materials and pharmaceutical applications of FFF.
1. Introduction
The potential of additive manufacturing, and especially the use of three-dimensional (3D) printing technologies for the development of novel drug delivery systems (DDSs), has been proven over the past decade [Citation1]. The fused filament fabrication (FFF) technique is the key technology when aiming for designing complex DDSs with unique properties, e.g., various geometries and multilayered structures providing tailored drug release profiles or site specificity [Citation1–Citation3]. A thermoplastic polymer-based filament is the feedstock in FFF. The filaments are either commercially available or developed by the hot melt extrusion (HME) process; thus, the coupling of HME with FFF for on-demand fabrication of DDSs is a common approach [Citation4]. The filament is gear-forced in the heating block of the printhead. This chamber is usually operated at elevated temperatures, above the glass transition, the melting or softening point of the polymer, to increase the mobility of the polymer chains. Subsequently, the melt flows through the nozzle of the printhead and deposits onto the build platform, thereby solidifying at predetermined patterns, according to the digital design of a 3D object [Citation5]. The FFF printing provides the ability to develop versatile and complex structures, and also the implementation of product design principles in a cost-effective and patient-centered fashion [Citation6]. Nonetheless, the nature and process of this technique present limitations to the active pharmaceutical ingredients (APIs) and excipients that can be used. Thermal degradation, a limited choice of printable (thermoplastic) materials suitable for oral uptake, and unique physical characteristics of the feedstock are just some evident shortcomings observed with FFF. Moreover, the scale-up of the printing process, along with the mass production of these products, has yet to be achieved. To overcome these boundaries, a few hybrid DDSs have been recently introduced () [Citation7]. The hybrid systems comprise a primary FFF-printed component, complemented by additional elements. The latter are principally produced via conventional or equally novel methods, e.g., compression, electrospinning, injection molding, and inkjet printing. This hybrid proof-of-concept approach aims to resolve the shortcomings of the FFF process, resulting in the fabrication of DDSs with enhanced functional properties.
Table 1. Hybrid drug delivery systems that incorporate the FFF technology (ABS, acrylonitrile butadiene styrene; EC, ethyl cellulose; HPC, hydroxypropyl cellulose; HPMC, hydroxypropyl methylcellulose; HPMCAS, hydroxypropyl methylcellulose acetate succinate; PCL, poly – ε – caprolactone; PEO, polyethylene oxide; PLA, polylactic acid; PLLA, Poly L-lactic acid; PVA, poly(vinyl alcohol)).
2. Hybrid drug delivery systems
2.1. Filled formulations
Container-like shapes can be effectively manufactured in a tailor-made approach via FFF; an array of filling formulations has been suggested to occupy the interior of the printed object. These formulations are produced by other techniques, and the filling procedure can be realized through (a) manual filling or (b) automated filling.
The produced hybrid DDSs incorporate the advantages of both manufacturing facets. For instance, the manual-filling approach provides the potential to incorporate a thermolabile API in the DDS, avoiding the unnecessary thermal stress to the sensitive API throughout the HME and FFF processing of the primary material. At the same time, this approach provides the possibility to adjust and tightly regulate the targeted delivery and the release mechanism of the API. Floating systems have been developed by combining FFF and tablet compression, exhibiting controlled drug release [Citation8–Citation10]. Furthermore, a floating device was designed and printed by FFF, aiming to host a commercially available drug-loaded capsule [Citation11]. Multicompartmental devices, loaded with SNEDDS [Citation12], drug-loaded hot melt extrudates [Citation13] or bulk APIs [Citation14,Citation15], and suppository dosage forms [Citation16], were also fabricated. Moreover, regular tablet and capsule shapes have been investigated as potential carriers that provide the targeted drug delivery of alginate beads [Citation17] and hydrogels [Citation18]. The possibility of adopting innovative designs in these multicompartmental devices allows achieving completely new types of drug release behavior () [Citation19].
Figure 1. Illustration of the potential of 3D printing for engineering drug release. The rows are representative examples of (from top to bottom): different tablet geometries (tablet length is denoted as L), their respective plots of normalized tablet width (w), and the experimental/theoretical data of drug release (blue/red dotted lines) corresponding to the different tablet shapes. Reprinted with permission from reference [Citation19].
![Figure 1. Illustration of the potential of 3D printing for engineering drug release. The rows are representative examples of (from top to bottom): different tablet geometries (tablet length is denoted as L), their respective plots of normalized tablet width (w), and the experimental/theoretical data of drug release (blue/red dotted lines) corresponding to the different tablet shapes. Reprinted with permission from reference [Citation19].](/cms/asset/7daf4648-1543-4216-b3ad-dfd2e17b1572/iedd_a_1776260_f0001_oc.jpg)
According to additional studies, the one-step fabrication and filling of the object was reported. The development of hollow formulations via both manual and automated filling of capsules with solid granules and a liquid formulation, respectively, has been investigated, including the consideration of an in vitro/in vivo relationship [Citation20]. Such an approach can unite the advantages of the FFF technique with an additional time-saving merit, compared to manual filling. Ordinarily, the automated filling process is realized upon modifying a dual-extruder 3D printer, by replacing a printhead with a syringe [Citation20,Citation21]. Recently, a modular 3D printer, previously used only for tissue applications, provided the ability of both FFF printing and injection volume filling [Citation22]; this device facilitated the development of capsules with unique drug release profiles of solutions and suspensions.
2.2. FFF/electrospinning
One of the earliest adoptions of 3D printing in combinatorial systems for drug delivery was the coupling of FFF with electrospinning (ES) to produce drug loaded scaffolds [Citation23]. The ES process generates fibers of various diameters, inexpensively, with their most crucial advantage being that they can mimic the extracellular matrix. In turn, FFF is utilized both for its rapid structure prototyping capabilities and the mechanical properties of the objects. Thus, a drug-loaded electrospun graft was shielded with a 3D-printed outer layer to improve the mechanical properties and structural integrity of the scaffold. More recently, the reverse approach was implemented by adjusting the pore size of 3D-printed scaffolds to address the needle-and-thread fixing of nasal cartilage implants, and subsequently, overlaying them with an electrospun drug-containing hydrogel to improve the cell adhesion and proliferation properties of the scaffold [Citation24].
2.3. Other systems
Finally, FFF has been coupled with other processes and materials to produce layered formulations, due to the high customizability and rapid prototyping capability of this technique. In an attempt to overcome the limitation of FFF, regarding the possibility for mass production, Fuenmayor and his coworkers have studied a hybrid system, where injection molding (IM) and FFF were employed to develop bilayer dual drug-containing tablets [Citation25]. The small FFF-printed part of the tablet provided a customizable and personalized dosage of the first API. This 3D-printed part was subsequently inserted in the mold, where the larger part of the tablet, containing a non-patient-specific dose of the second API, was produced. Thus, the mass production capabilities of IM were exploited and combined with the patient-centric orientation of FFF. In a recent study, the coupling of FFF with inkjet printing was explored to prepare quick response (QR) codes and data matrices onto 3D printed tablets, as anti-counterfeit measures [Citation26]. An alternative approach, compared to the conventional methodologies in the development of multilayered buccal films, was recently reported. Drug loaded buccal films were fabricated via FFF onto commercial wafer paper, commonly used as a substrate for inkjet printing [Citation27]. The wafer acted as the backing layer and imparted unidirectional release properties to the formulations, by effectively inhibiting the extensive diffusion of the API toward the oral-cavity-simulating environment.
3. Conclusion
The emergence of 3D printing in pharmaceutics is evident by the increasing number of publications over the last decade and the establishment of startup companies moving toward this direction, due to the multifaceted advantages it offers. The recent coupling of 3D printing with several other techniques and approaches was found both successful and promising, regarding the development of hybrid DDSs, by uniting their respective advantages and avoiding limitations of each technique.
4. Expert opinion
The recent expiration of patents related to additive manufacturing devices was a key to the successful introduction of the 3D printing technology in the list of cutting-edge formulation processes [Citation28]. The commercial release of the first 3D-printed tablet (Spritam®, Aprecia Pharmaceuticals), together with the first reported application of FFF in pharmaceutical technology [Citation29], stimulated the academic sector globally. This development was further motivating the emergence of novel formulation approaches for drug delivery by using the FFF technology. Since then, a growing number of printable materials has been reported during the development of FFF-printed DDSs; however, it should be noted that this number is moderate compared to the available thermoplastic polymers, excipients, and APIs used in the development of pharmaceutical products via standard technologies (e.g., granulation, tableting, pelletization, coating). A key aspect in this work is to build the knowledge base for finding out the unique possibilities of FFF that the conventional drug manufacturing solutions lack [Citation30]. Beyond the common drawbacks of additive manufacturing (object anisotropy, mass production issues), the boundaries of FFF are defined by the intimate nature of the process [Citation6]. The required mechanical strength and physical dimensions of the filaments are essential to facilitate the smooth and continuous feeding of the filament to the printhead, which would result in objects with excellent accuracy and repeatability. Another critical attribute that should be considered is the high-thermal processing of the feedstock throughout the build cycle, raising the burden of thermal degradation and rendering the FFF printing of thermosensitive constituents arbitrary. At this point, hybrid DDSs are providing excellent potential to tackle the current limitations of FFF. With global digitalization, other drug manufacturing solutions, e.g., inkjet printing, can be utilized ad hoc to FFF and used to generate drug-containing data matrices that would make the supply chain of pharmaceuticals transparent and protected against counterfeiting [Citation31,Citation32].
The recent FDA- and industry-driven trends are moving toward addressing the current clinical needs via the existing additive manufacturing techniques [Citation33]. On the other hand, there is a constantly growing number of new biologics approved by the FDA, accounting for sixty-nine new biologics approvals in the past few years (2014–2019) and 21% of the total approvals of therapeutics in 2019 [Citation34–Citation36], which are expected to dominate the global pharmaceutical market [Citation37]. Similarly, several life-saving antiviral treatments are based on multi-drug administration, including treatment of, e.g., HIV and hepatitis C. Accordingly, efforts in this era to combat the pandemic COVID-19 are currently (end of March 2020) centered around multi-drug solutions [Citation38]. Additive manufacturing might have a key role to personalize the production for both clinical trials as well as final commercial scale. As thermal degradation at moderate temperatures represents a major issue for most biologics and even small molecules, the development of hybrid DDSs for the effective delivery of these molecules can be a logical solution. Compartmentalized hybrid DDSs allow for personalization of not only the dose of different compounds in the same dosage unit, but also customization of the release behavior for each incorporated drug ().
Figure 2. A conceptualized hybrid DDS with multiple chambers that provide immediate (blue), delayed (red), and sustained (green) release profiles of different APIs, as well as functional elements (white), e.g., mucoadhesive components, permeation enhancers, stabilizers, enzyme inhibitors and pH regulators.
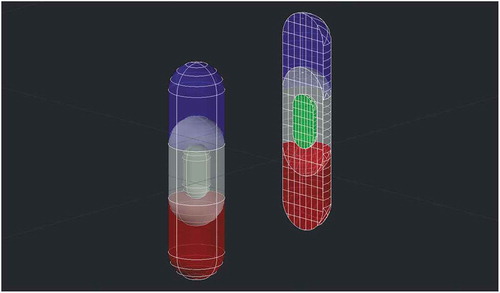
Overall, hybrid DDSs are thoroughly expanding the capabilities of FFF together with other traditional manufacturing processes to a considerable extent of applications in the pharmaceutical and medical fields. Conceptual treatments that are often described in the literature, regarding the incorporation of model drugs, might be eventually translated to clinical practice via hybrid DDSs. Considering the academic effort that has been done in this field during the last years, and the exceptionally broad spectrum of available manufacturing technologies, more combinatorial approaches are expected to emerge in the immediate future. These approaches are believed to provide smart solutions to the current clinical needs from the aspect of personalized treatments.
Declaration of interest
The authors have no relevant affiliations or financial involvement with any organization or entity with a financial interest in or financial conflict with the subject matter or materials discussed in the manuscript. This includes employment, consultancies, honoraria, stock ownership or options, expert testimony, grants or patents received or pending, or royalties.
Reviewer disclosures
Peer reviewers on this manuscript have no relevant financial or other relationships to disclose.
References
- Mathew E, Pitzanti G, Larrañeta E, et al. 3D printing of pharmaceuticals and drug delivery devices. Pharmaceutics. 2020;12(3):266.
- Lim SH, Kathuria H, Tan JJY, et al. 3D printed drug delivery and testing systems — a passing fad or the future?. Adv Drug Deliv Rev. 2018;132:139–168.
- Gioumouxouzis CI, Karavasili C, Fatouros DG. Recent advances in pharmaceutical dosage forms and devices using additive manufacturing technologies. Drug Discov Today. 2019;24:636–643.
- Tan D, Maniruzzaman M, Nokhodchi A. Advanced pharmaceutical applications of hot-melt extrusion coupled with fused deposition modelling (FDM) 3D printing for personalised drug delivery. Pharmaceutics. 2018;10(4):203.
- Alhnan MA, Okwuosa TC, Sadia M, et al. Emergence of 3D Printed Dosage Forms: opportunities and Challenges. Pharm Res. 2016;33:1817–1832.
- Uziel A, Shpigel T, Goldin N, et al. Three-dimensional printing for drug delivery devices: a state-of-the-art survey. J 3D Print Med. 2019;3:95–109.
- Melocchi A, Uboldi M, Maroni A, et al. 3D printing by fused deposition modeling of single- and multi-compartment hollow systems for oral delivery – A review. Int J Pharm. 2020;579:119155.
- Fu J, Yin H, Yu X, et al. Combination of 3D printing technologies and compressed tablets for preparation of riboflavin floating tablet-in-device (TiD) systems. Int J Pharm. 2018;549:370–379.
- Huanbutta K, Sangnim T. Design and development of zero-order drug release gastroretentive floating tablets fabricated by 3D printing technology. J Drug Deliv Sci Technol. 2019;52:831–837.
- Reddy Dumpa N, Bandari SA, Repka M. Novel gastroretentive floating pulsatile drug delivery system produced via hot-melt extrusion and fused deposition modeling 3D printing. Pharmaceutics. 2020;12:52.
- Charoenying T, Patrojanasophon P, Ngawhirunpat T, et al. Fabrication of floating capsule-in- 3D-printed devices as gastro-retentive delivery systems of amoxicillin. J Drug Deliv Sci Technol. 2020;55:101393.
- Markl D, Zeitler JA, Rasch C, et al. Analysis of 3D prints by X-ray computed microtomography and terahertz pulsed imaging. Pharm Res. 2017;34:1037–1052.
- Genina N, Boetker JP, Colombo S, et al. Anti-tuberculosis drug combination for controlled oral delivery using 3D printed compartmental dosage forms: from drug product design to in vivo testing. J Controlled Release. 2017;268:40–48.
- Maroni A, Melocchi A, Parietti F, et al. 3D printed multi-compartment capsular devices for two-pulse oral drug delivery. J Controlled Release. 2017;268:10–18.
- Matijašić G, Gretić M, Vinčić J, et al. Design and 3D printing of multi-compartmental PVA capsules for drug delivery. J Drug Deliv Sci Technol. 2019;52:677–686.
- Tagami T, Hayashi N, Sakai N, et al. 3D printing of unique water-soluble polymer-based suppository shell for controlled drug release. Int J Pharm. 2019;568:118494.
- Gioumouxouzis CI, Chatzitaki A-T, Karavasili C, et al. Controlled release of 5-fluorouracil from alginate beads encapsulated in 3D printed pH-responsive solid dosage forms. AAPS PharmSciTech. 2018;19(8):3362–3375.
- Eleftheriadis GK, Katsiotis CS, Bouropoulos N, et al. FDM-printed pH-responsive capsules for the oral delivery of a model macromolecular dye. Pharm Dev Technol. 2020;25(4):517–523.
- Sun Y, Soh S. Printing tablets with fully customizable release profiles for personalized medicine. Adv Mater. 2015;27(47):7847–7853.
- Smith D, Kapoor Y, Hermans A, et al. 3D printed capsules for quantitative regional absorption studies in the GI tract. Int J Pharm. 2018;550(1–2):418–428.
- Okwuosa TC, Soares C, Gollwitzer V, et al. On demand manufacturing of patient-specific liquid capsules via co-ordinated 3D printing and liquid dispensing. Eur J Pharm Sci. 2018;118:134–143.
- Linares V, Casas M, Caraballo I. Printfills: 3D printed systems combining fused deposition modeling and injection volume filling. Application to colon-specific drug delivery. Eur J Pharm Biopharm. 2019;134:138–143.
- Centola M, Rainer A, Spadaccio C, et al. Combining electrospinning and fused deposition modeling for the fabrication of a hybrid vascular graft. Biofabrication. 2010;2(1):014102.
- Rajzer I, Kurowska A, Jabłoński A, et al. Layered gelatin/PLLA scaffolds fabricated by electrospinning and 3D printing- for nasal cartilages and subchondral bone reconstruction. Mater Des. 2018;155:297–306.
- Fuenmayor E, O’Donnell C, Gately N, et al. Mass-customization of oral tablets via the combination of 3D printing and injection molding. Int J Pharm. 2019;569:118611.
- Trenfield SJ, Xian Tan H, Awad A, et al. Track-and-trace: novel anti-counterfeit measures for 3D printed personalized drug products using smart material inks. Int J Pharm. 2019;567:118443.
- Eleftheriadis GK, Ritzoulis C, Bouropoulos N, et al. Unidirectional drug release from 3D printed mucoadhesive buccal films using FDM technology: in vitro and ex vivo evaluation. Eur J Pharm Biopharm. 2019;144:180–192.
- Araújo M, Sa-Barreto L, Gratieri T, et al., The digital pharmacies era: how 3d printing technology using fused deposition modeling can become a reality. Pharmaceutics. 2019;11(3): 128.
- Goyanes A, Buanz ABM, Basit AW, et al. Fused-filament 3D printing (3DP) for fabrication of tablets. Int J Pharm. 2014;476(1–2):88–92.
- Aho J, Bøtker JP, Genina N, et al., Roadmap to 3D-printed oral pharmaceutical dosage forms: feedstock filament properties and characterization for fused deposition modeling. J Pharm Sci. 2019;108(1): 26–35.
- Edinger M, Bar-Shalom D, Sandler N, et al. QR encoded smart oral dosage forms by inkjet printing. Int J Pharm. 2018;536(1):138–145.
- Nørfeldt L, Bøtker J, Edinger M, et al., Cryptopharmaceuticals: increasing the safety of medication by a blockchain of pharmaceutical products. J Pharm Sci. 2019;108(9): 2838–2841.
- Di Prima M, Coburn J, Hwang D, et al. Additively manufactured medical products – the FDA perspective. 3D Print Med. 2016; 2(1). DOI: 10.1186/s41205-016-0005-9
- Mullard A. 2017 FDA drug approvals. Nat Rev Drug Discov. 2018;17(2):81–85.
- Mullard A. 2018 FDA drug approvals. Nat Rev Drug Discov. 2019;18(2):85–89.
- Mullard A. 2019 FDA drug approvals. Nat Rev Drug Discov. 2020;19(2):79–84.
- Wahlich J, Desai A, Greco F, et al., Nanomedicines for the delivery of biologics. Pharmaceutics. 2019;11(5): 210.
- [cited 2020 Mar 1]. https://clinicaltrials.gov/.