ABSTRACT
Introduction
In hydrocephalus treatment, ventriculo-peritoneal shunts (VPS) have become the most relevant therapy for seven decades among other treatment options. Due to the hydrostatic pressure in vertical position, CSF diversion is somehow non-physiological. The integration of gravitational valves in VPS was established to counteract the hydrostatic draining force and to approach a physiological condition of the cerebrospinal diverting system. Numerous clinical studies have shown that gravitational valves are able to reduce secondary complications of VPS treatment. It remains a challenge for the treating neurosurgeon to select the correct valve resistance based on individual anatomies and different etiologies of hydrocephalus as well as varying levels of activity of the patient.
Areas covered
This review covers the development of gravitational shunt valves from historical, theoretical and clinical aspects for pediatric and adult etiologies of hydrocephalus. We discuss the role of gravitational shunt valves in preventing over-drainage issues and present the state-of-the-art literature. Furthermore, ongoing prospective trials are presented.
Expert opinion
Counteracting the hydrostatic force by selecting the correct valve in a VPS system to achieve physiological balance in CSF diversion during vertical and horizontal body changes has become the current standard for hydrocephalus management. Gravitational shunt valves reliably address this need to minimize over-drainage events in the vertical position without affecting the CSF flow in the horizontal position. The results of ongoing prospective studies on the safety and efficacy of adjustable gravitational valves are still pending. Due to the complexity of the CSF flow, lifelong follow-up care for patients with VPS is critical.
1. Introduction
The treatment of hydrocephalus is one of the least loved but maybe most effective duties of a neurosurgeon. Since the introduction of CSF shunts in the early 1950s, hydrocephalus treatment was revolutionized as mortality rates dropped from about 70% to less than 1% in the developed world until today. Medical care for patients with all kinds of hydrocephalus requires straightforward surgeries, but fundamental knowledge about valve types and a certain enthusiasm for life long follow-up of the patients. Half a century after introduction of the first ventriculo-peritoneal shunts (VPS), the three possible results of CSF diversion still remain: too little, too much or optimal drainage. The CSF diverting system is trying to imitate the impaired physiological function of CSF resorption which might still be dependent on variable CSF production. A VPS is a system of communicating tubes connecting the intraventricular cavity with the peritoneal cavity over differing distances depending on the patient’s height, thus creating an additional dependency on body position. Among different forces, the intraventricular pressure activates drainage of CSF while the resistance in the shunt system as well as the pressure in the peritoneal cavity are counteracting forces. In addition, the hydrostatic difference between cerebral ventricles and abdominal cavity is about zero in a lying patient. However, depending on the height, hydrostatic forces of 20–60 cmH2O exist in a standing patient which plays an important role for CSF drainage (). The physiological intracranial pressure lies between 0 to 15 cmH2O but may decrease during body erection toward an upright position from 0° to about 20° [Citation1,Citation2]. Further elevation of the upper body between 20° and 90° results in stable intracranial pressures since the blood outflow is restricted by collapsing jugular veins of the neck. Thus, a valve system, which regulates the CSF drainage in a shunt system, faces the following challenges: 1. adjusting the needs of CSF drainage dependent on the varying CSF production as well as residual CSF resorption. 2. Acknowledging influences of body weight and fat distribution on the intraperitoneal cavity pressure levels. 3. Adapting to differing activity levels, which might influence differential pressure changes between the ventricles and the peritoneal cavity. 4. Counteracting hydrostatic pressure in an upright position, under the influence of the patient’s growth (). Optimally a valve automatically regulates CSF diversion through an automatic feedback loop for CSF production and resorption, the measurement of pressure changes, and the body position. Indeed, these requirements might be complicated to manage without electronic regulation. Thus, the mechanical solutions have introduced adjustable differential pressure valves which address the hydrostatic problem to enable physiological CSF diversion in shunt systems at affordable costs.
Figure 1. Forces that are influencing CSF diversion through a ventriculo-peritoneal shunt system. (a) CSF draining forces and resistances are given in different body positions. In the lying position, the major draining force for CSF is the intraventricular pressure (green arrow), while resistances are defined by the intraperitoneal pressure (red arrow) and the resistance of the shunt system and the integrated valve (orange arrow). In the standing position the height level differences between the head and the peritoneum is creating communicating system by the shunt tubing resulting in a hydrostatic force driven by gravity (long green arrow). This may be counteracted by gravitational shunt valves creating an additional resistance in an upright position (long orange arrow). (b) The different forces are dependent on variable factors. The ratio between CSF and brain volume somehow defines the amount of CSF which is able to be drained as well as the underlying diagnosis of hydrocephalus determines the amount of CSF which cannot be resorbed naturally. Thee intraperitoneal pressure is influenced by the body weight, body mass index and the fat distribution, which might be different in females compared to males after puberty. The hydrostatic force is determined by growth and the differential pressure between the intraventricular and the intraperitoneal cavity and is dependent on the activity level of the patient which is certainly age dependent as well
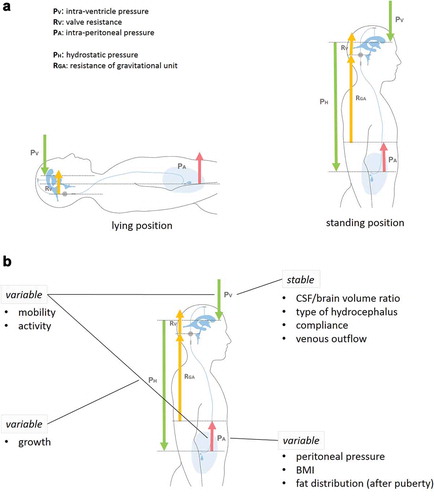
Table 1. Clinical studies on gravitational valves
Table 2. In vitro studies on gravitational valves
The aim of this work was to systematically review gravitational valves for the therapy of hydrocephalus. There are several other technical principles to prevent overdrainage in CSF shunts. These devices are mentioned as far as they are important for the understanding of the development of gravitational devices. The intention of this review neither was to compare the efficacy of gravitational valves and other anti-siphoning devices nor to propagate the superiority of gravitational valves over other devices. Additionally, the authors acknowledge and value endoscopic treatment options, which have become increasingly important in non-communicating hydrocephalus, such as aqueductal stenosis, or in the heterogeneous etiologies of pediatric hydrocephalus. It does not lie within the scope of this review to cover all other treatment alternatives of hydrocephalus. These topics will have to be addressed in other reviews.
1.1. Historical overview of gravitational shunt valves
The treatment of hydrocephalus started in the very early times of the neurosurgery with short-term implants, e.g. external ventricular drainages by Wernicke 1881 [Citation3] or surgical techniques without implants, e.g. plexectomia and third ventriculostomy i.a. by Dandy, 1918 [Citation3]. The breakthrough for the treatment introduced permanent implants 1952 on the basis of new materials which allowed the production of elastic drainage systems and of the first slit valves [Citation4,Citation5]. From this time on, simple shunt valves solved basic problems of hydrocephalus, but new problems emerged simultaneously. Among others, it was recognized that individual patients needed different drainage volumes over time. Thus, several valve types with different opening pressures (low-, medium- or high-pressure valves) were put on the market, which resulted in difficulties to select the right valve for each patient. Furthermore, it became obvious, that valves might need an adaptable opening pressure in order to be adjusted for varying patient needs. At that time VP shunts were equipped with slit valves and membrane valves which were inapt to address these issues. The subsequently developed ball in cone-valves, showed the potential for the integration of adaptive resistance levels. The adjustability of valves was at first referring to differential pressure units which were called ‘programmable valves’ as of then. Even though this description sounds more sophisticated and meets marketing needs, the term was misleading and did not refer to an actual programming process, but rather an adjusting procedure. One of the most widespread adjustable differential valves on the market was the Codman–Hakim programmable valve without a gravitational functionality. It was constructed with a silicone housing and the micro-mechanics were able to respond to the adjustment of the resistance between 30 and 200 mmH2O through a magnetic mechanism. In addition, this mechanism was also able to adjust the resistance at different body positions, since the closing mechanism is essentially initiated by gravity. The gravity dependence is a function of the weight of the closing ball (). Hence, technical constructions could further develop this effect in order to design an orientation-dependent valve. Since hydrocephalus valves have to control very low flow rates, the closing mechanism warrants high precision using closing balls with extremely low dimensional tolerances. This demand is met by ruby balls. Ruby balls are convenient for the valve assembly by hand but have the ‘disadvantage’ of a low weight, which is why the gravitational effect has to be amplified. The first construction with marketability was the Cordis–Hakim lumbar valve (CHLV, Cordis, Santa Clara, CA, USA). It was first to realize the construction principle of metal balls, which can move within a cylindric housing. Then, the sinus function of the angle of the housing and the weight of the metal balls act as the opening pressure of the valve. With little exceptions, this concept has been transferred to almost all gravitational valves later on: The Chhabra-Z-flow (Surgiwear Ltd., Shahjahanpur, India) was a low-cost variant with a housing of silicone [Citation6]. The Cordis gravity compensating accessory (GCA) was an additional module connectable with differential pressure valves analogous to the CHLV. The Miethke Dual-switch valve (MDSV, Miethke GmbH & Co. KG, Potsdam, Germany) used the principle in order to switch between two different chambers of the valve with different opening pressures within a single housing [Citation7,Citation8]. The Miethke Shunt Assistant (SA) is a technically improved additional valve like the GCA which can be combined with other differential pressure valves but has mostly been implanted in a preconfigured combination with a programmable differential pressure valve (proGAV) [Citation9–11]. The Sophysa SiphonX (Sophysa SA, Orsay, France) is an analogous construction [Citation12]. The Miethke Gravity-assisted valve (GAV) was a former variant of a combination of the SA and a nonprogrammable differential pressure valve in one small housing [Citation13,Citation14]. ()
Figure 2. Schematic drawing of a gravitational valve which includes a heavy ball putting varying weight on a small ball which is part of the ball in cone mechanism establishing the valve function. In a lying position the heavy ball will not influence the ball in the cone valve resulting in a free flow of CSF (white arrows). During erection of the valve from horizontal to vertical position the gravity associated weight of the heavy ball will occlude the ball in the cone valve with gradually increasing force (red arrows). The same force is needed by CSF to open the valve to establish flow, which is defined by the differential pressure between inflow and outflow water column
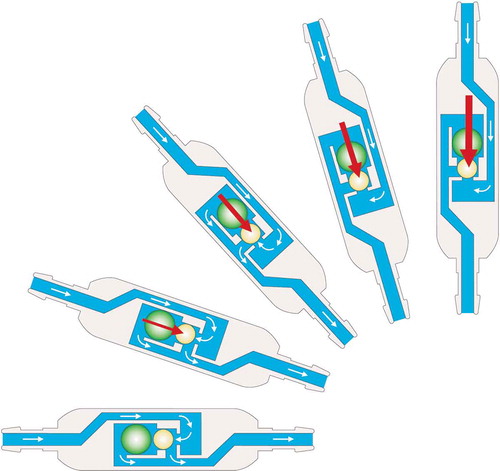
Figure 3. Schematic drawings of all available gravitational valve types: The Z flow valve (Chabra) is not available in Europe and the U.S. The gravity compensating accessory and the H-V lumbar valve (Integra) exert the closing force by adding the weight of three balls. The siphon X (Sophysa) and the shunt assistant (Miethke) contain a ball made of tantalum (the heaviest inert metal). In the dual switch valve (Miethke) a tantalum ball is used as a switch in order to channel the CSF either through a valve with a lower or a valve with a higher opening force
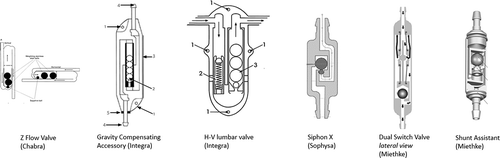
The limitation of the construction with additional metal balls as gravity bodies was adjustability. The ideas of a controllable dispensation of the metal balls like in a gumball machine have never been realized [Citation15]. Another concept bore the potential of a programmable construction: a weight mounted on a lever arm which on its part increases the closing force of a ball in cone valve. This principle was unsuccessful at first due to design defects of the non-programmable Sophysa AS (anti-siphon) valve which disappeared from the market without a citable reference. Inside the Miethke programmable shunt assistant (proSA), the lever weight is modified by a spring, which can be variably preloaded by an externally movable eccentric using a magnetic tool. The proSA was then the first truly programmable gravitational device [Citation16]. The latest gravitational valve is the Miethke M.blue, which represents a combination of the proSA and a fixed differential pressure valve in one housing.
1.2. In vitro-testing of gravitational valves
In vitro analyses of gravitational units allow investigation of flow and pressure characteristics mimicking in vivo performance [Citation12,Citation17]. The results of in vitro tests of all historically developed gravitational valves are extensively described by Aschoff [Citation15]. More recently published data investigated anti-hydrostatic units available on the market today [Citation12] (). Interestingly, relevant differences in the flow characteristics were observed in the gravitational units from different manufacturers. Their described function was verified by checking if the added resistance in an upright position shifted the flow along a linear differential pressure relation. However, the flow curves of the different valves differed significantly from a linear function, the least of which was the Miethke SA. In addition, membrane-regulated anti-hydrostatic units (Anti-siphon device and Delta Chamber) as well as a spiral flow-reducing device (SiphonGuard) were tested. Those valves showed different flow patterns compared to the gravitational units, shifting the curves toward lower flow values as being dependent mainly on hydrostatic pressure in the membrane-regulated devices. Still, two disadvantages have been discovered; first, the flow was not necessarily lower compared to the SA at related differential pressure (DP) levels and second, the mechanism takes place on the surface of the device itself, thus being influenced by the external pressure acting on the membrane. The flow regulating device, SiphonGuard, actually reduced the flow to a minimal level after being activated. However, no clinical experiences have been published so far, reporting if the device works properly in vivo when exposed to elevated protein levels in the CSF or if the device has ever caused underdrainage [Citation12].
In another study the impact of different motion patterns on the flow performance were analyzed [Citation18]. Kimura et al. used the SA and proSA in an in vitro laboratory setup (see [Citation12] for detailed description) to measure the draining volume over time at a hydrostatic DP between 0 and 60 cmH2O. The valves were tested at angles between 0° (horizontal position) and 90° (vertical position). With the help of an electric motor oscillation frequencies of 2, 3, and 4 Hz were simulated, respectively. First, the investigators examined the flow in the horizontal position. The SA and proSA were open to flow in the horizontal position regardless of the resistance values. Kimura et al. [Citation18] showed that there was significantly less flow through the SA (from 2 ± 0.0 ml/min at 10 cmH2O to a flow of 16.4 ± 0.2 ml/min at 60 cmH2O) then the proSA (3.8 ± 0.0 ml/min to 19.6 ± 0.4 ml/min). In the vertical position (90°) both devices create an added resistance through the weight of a tantalum ball in the SA and through a tantalum pendulum in the proSA. The flow measurements in the vertical position detected significantly lower flow values compared to the horizontal position regardless of the DP used [Citation18]. At valve angulation of 30° and 60°, the SA showed no flow at DP lower than the predefined/adjusted resistance while proSA still showed minimal flow. These findings confirm the results of the in vitro tsets of Czosnyka et al. [Citation19]. The authors explained these findings with a higher resistance due to the smaller housing design of the SA [Citation18]. The resistance in the SA increases almost immediately in the vertical position because the tantalum ball falls onto the outlet of the valve abruptly. The resistance in the proSA is established later due to the tantalum pendulum, which is activated in relation to the spring’s tension, especially when the valve settings are low. At these settings, the syringe’s tension is high, and the pendulum is hindered to fall in the upright position.
The in vitro testing showed that 3- and 4-Hz motion (equivalent with running) overcame the gravity resistance and flow rates of up to 30 ml/h were detected even if DP was lower than the predefined/adjusted resistance. This motion-induced additional CSF flow could lead to activity-related overdrainage, especially in children. Interestingly, in the proSA at lower settings (0–20cmH2O) the flow was reduced with motion, but it was increased in higher settings (20–40cmH2O), which is again most likely related to the different tension preload in the spring acting on the tantalum pendulum.
Hydrodynamics were not altered by temperature change and therefore fever or low ambient temperature seems not to have any influence on CSF drainage [Citation19]. Any time-related trends in flow-rates during the 3 months of testing were not observed [Citation19]. Similar findings have been reported by Allin et al. [Citation20] for the proGAV, which was tested for temperatures between 34° and 40°C and during a long term in vitro analysis over a 28-day test period without any decrease in the performance of the valve. In vitro MRI safety to avoid unintended readjustments of the valve could be confirmed [Citation20]. Due to a low hydrodynamic resistance of the valve, the authors mentioned that care must be taken when shortening the peritoneal catheter, which is normally responsible for a relevant amount of the total shunt resistance [Citation20]. For patients with intermittent intracranial hypertension, the proGAV valve is reliable due to low resistance and the ability to compensate for an acute ICP elevation [Citation20–23].
Gehlen et al. [Citation21] compared three different types of anti-siphon devices (ASD) in a bench test simulating natural posture changes of a patient with a VPS. The authors described posture-related flow-reducing effects with different characteristics in all devices. Nevertheless, the increase of the opening pressure in gravitational ASDs was lower than expected when the system was brought into the upright position. Further, the authors mentioned that head tilt of more than 20° in the upright or sitting position leads to a significant increase of the CSF drainage and a consecutive risk of over-drainage. This problem has already been addressed by Park et al. [Citation23] in vivo for gravitational valves implanted with an anterior axis deviation of 20° or more. Gehlen et al. [Citation21] reported ‘audible vibrations’ of open gravitational valves. Stockhammer and Sprung [Citation22] mentioned this phenomenon in vivo in four patients and related it to vibrations of the closing ball caused by CSF flow peaks.
The results of the in-vitro testing and the knowledge of the specific flow characteristics of each device should be carefully studied since it enables neurosurgeons to choose the appropriate device for each hydrocephalus patient. This essential knowledge can offer neurosurgeons’ the ability to act appropriately if cases of over- or under-drainage are observed, which are related to incompatibilities of the implant in relation to the patient’s needs rather than to secondary shunt complications
1.3. Clinical evidence
The clinical publications cover pediatric patients, patients with idiopathic normal-pressure hydrocephalus and patient collectives with heterogeneous origins of secondary hydrocephalus ().
1.3.1. Pediatric patients
The relevant problem of over-drainage in children with shunted hydrocephalus was already described in the 1970s [Citation24–26]. One of the specific problems of over-drainage is that it is not well defined in the pediatric literature. However, it is well known that over-drainage rarely results in acute symptoms but frequently causes chronic anatomical changes, which might be discovered only after several years of shunting. Those include slit ventricle syndrome, proximal catheter obstruction, restriction in head growth, microcephaly, and secondary Chiari malformation. If over-drainage rates are reported in the literature, they are not necessarily described in a standardized fashion and must be compared cautiously among the studies. The first attempts to overcome over-drainage by different mechanic solutions for shunting were the Delta anti-siphon device, the so-called flow-regulated valve system Orbis Sigma, and the differential pressure valve Medos-Hakim programmable valve system [Citation27–29]. The first series of pediatric hydrocephalus patients treated with gravity-assisted valves was published in 2005 by Meling and coworkers [Citation30]. They used the peadiGAV as a salvage valve for patients with over-drainage problems in need for shunt revision. The selected opening pressures were 9/24 or 9/29 cmH2O. The group observed a revision-free survival rate of 53.1% after 12 months and 44% after 2 years with a mean follow-up of 20.8 months. They observed a remaining over-drainage rate of 9.4%. Two years later Eymann and colleagues published their single-center experience in a prospective study using the paediGAV as standard valve in children with shunt-dependent hydrocephalus. They used valve opening pressures of 9/19 cmH2O or 9/29 cmH2O and observed over drainage rate of 3.6%. Still, they observed decreasing head circumferences over time after shunt implantation, which implicates higher subclinical over-drainage rate. The revision-free survival rates of 75% and 68% for 12 and 24 months with a mean follow up of 218 ± 468 days were positive results [Citation31]. A prospective multi-center observational study published by Haberl and coworkers offered a more realistic experience with fixed gravitational valves in children. One hundred and sixty-nine patients were included in the study treated with a wide range of different opening pressures of the peadiGAV between 4/14 and 9/29 cmH2O. The over-drainage rate was reported at 4.7% and the revision-free survival was 72.6 and 62% at 12 and 24 months of the follow-up. Other complications were valve obstruction in 4.7% and proximal catheters obstructions in 10% [Citation14]. Especially the latter one can also be interpreted as secondary sign of over-drainage in some of the cases. In a more recent study from the United Kingdom the long-term experience with paediGAV in pediatric hydrocephalus was described to be favorable. Most of the valves used were paediGAV 9/29 cmH2O in 162 patients with an observed over-drainage rate of 1.6%. The five-year revision-free survival rate was 57.9%, with 62.6% at 1 year and 59.1% at 2 years. Valve survival rate was 88.5%, 86.4% and 85.5% at one, two and 5 years of follow-up, respectively [Citation32]. Overall, the paediGAV seems to represent a reliable valve implant for the treatment of pediatric hydrocephalus. Nevertheless, it does not offer the possibility to further adapt the opening pressure individually without additional surgery.
In more recent years, adjustability became a useful adjunct in shunted hydrocephalus children. The different etiologies of hydrocephalus, different ages during which hydrocephalus is treated, and different levels of activity during the life stages challenge neurosurgeons to predict the optimal resistance in the shunt system to mimic the most physiological condition for the individual patient. The combination of an adjustable differential pressure unit with a fixed gravitational unit became a wider spread treatment in a lot of pediatric neurosurgical divisions. Weinzierl and coworkers reported the use of a Medos-Hakim programmable valve combined with a gravitational shunt assistance, in order to reduce the differential pressure and to increase the resistance by a fixed gravitational unit in the upright position in parallel. They supposed that a more physiological CSF drainage should be achieved by inhibiting the hydrostatic force of drainage in standing position and preventing further ventricular collapse [Citation33]. The first experience with the proGAV was published in 2012 in a bicenter study. 59 valves were reported to be reliable for the treatment of individual conditions of pediatric hydrocephalus. Although the follow-up time was limited, an overall success rate of 88.7% and a shunt revision-free survival at 12 months of 75.5% justified further use of this valve in children [Citation34]. In a larger cohort of 203 patients, 237 valves were followed up over 21.9 ± 10.3 months. The overall shunt survival rate was 64.3%, while the valve survival rate was 83.8%, respectively [Citation35]. The experience with shunt valve adjustments using the proGAV was further refined after observing that adjustments were necessary in about half of the patients without having an adjustment protocol. Moreover, valve adjustment made it possible to correct head circumference growth over time of follow up, reaching for a more physiological CSF drainage and overcoming chronic over-drainage problem. Therefore, it was concluded that valve adjustment can be used as treatment option for all hydrocephalic children by developing a protocol, accordingly. Thus, a guideline for proGAV adjustments in children was proposed according to age and activity levels dependent resistance levels and further adjustments according to clinical and imaging follow-up results [Citation36]. Although results could not be proven scientifically, first trends are observed that revision rates might be lower with adjustable differential pressure valves and gravitational units compared to fixed gravitational valves as well as simple differential pressure valves as reported in the literature [Citation35]. Long-term survival rates for infant children using the proGAV showed that a gravitational unit is well tolerated from early age and that adjustability opened up possibilities to adapt to a certain unpredictability of the correct valve’s resistance level during treatment of this fragile condition [Citation13]. A more recent Chinese study confirmed these observations [Citation37]. Alavi and coworkers observed that a proGAV, when implanted instead of a simple differential pressure valve, led to lower amount of shunt revisions in follow-up as well as a more physiological size of the ventricles [Citation38]. For complex conditions of myelomeningocele patients with spina bifida and hydrocephalus, the proGAV reached lower revision rates compared to other hydrocephalus entities, which has never been reported before for this clinical condition [Citation39]. The latest development of an adjustable gravitational valve (proSA) was evaluated either in mixed pediatric-adult cohorts or in single-center studies with limited information [Citation16,Citation40–42]. In general, the published literature offers some evidence that gravitational valves are feasible to use in the treatment of pediatric hydrocephalus and deliver more physiological characteristics of CSF diversion to overcome chronic problems of over-drainage more easily. It will remain a challenge for future investigator to define the optimal protocol for the most effective adjustments either within the differential pressure or the gravitational unit in pediatric hydrocephalus. Another remaining problem are the occlusion rates of shunt valves due to CSF protein overload as well as CSF and foreign material interaction [Citation43]. Future adaptations of the inner valve surface might reduce protein adhesion and thereby avoid one of the relevant but unsolved issues of valve complications.
1.3.2. Secondary hydrocephalus/NPH
This heterogeneous group includes disorders caused by subarachnoid hemorrhage, intracranial hemorrhage, tumor, traumatic brain injury, and infection [Citation44,Citation45].These patients are high-risk for revision surgery. The leading reasons for shunt-revision are infection (1.7 to 34%) and shunt obstruction (3.1 to 21%) [Citation46–49]. A higher incidence of shunt issues is predominantly seen in pediatric patients [Citation50,Citation51]. Studies on secondary hydrocephalus were mainly conducted to compare adjustable and non-adjustable valves [Citation47,Citation48,Citation50,Citation52]. Programmable valves allow adjustments of the opening resistance, but do not take the patient’s posture into account [Citation53]. Thus, these patients are at risk of developing clinical or radiological signs of overdrainage [Citation54]. Increasing the opening resistance of the programmable valve can be a treatment option for overdrainage, but it can result in underdrainage in horizontal position [Citation47,Citation55]. Studies addressing the overdrainage issue during therapy with gravitational valves are still under-represented. Overdrainage of up to 70% is described in the literature, but a rate of 20% appears to be more reliable [Citation54,Citation56].
Hertel et al. [Citation53] reported their clinical experience with 169 adult patients and different kinds of hydrocephalus treated with a Miethke Dual Switch valve (DSV). The primary end point was shunt-related surgery due to shunt obstruction, catheter dislocation or disconnection, under-/overdrainage and shunt infection. The opening pressure of the valve was chosen individually by each surgeon and the opening pressure of the gravitational unit was chosen according to the body height of the patient [Citation53]. The overall survival rate of the shunt was comparably favorable at 86% after 1 year [Citation10,Citation53]. Four patients (2.4%) developed overdrainage requiring valve replacement, three of them having been patients with idiopathic normal-pressure hydrocephalus (iNPH). Merely one single overdrainage was associated with secondary hydrocephalus. Valve obstructions were not observed even in the occlusive hydrocephalus subgroup with high CSF protein content. Twelve patients (7,1%) showed a displacement of the abdominal or ventricular catheter. The shunt system was explanted in ten patients (5,9%) due to a shunt infection. These shunt revisions were not valve associated. In this study, the DSV showed a favorable performance in the treatment of various types of hydrocephalus and showed the lowest complication rate due to overdrainage and obstruction. CSF with a high-protein content benefits from the large CSF contact area of the valve [Citation53]. The design of the valve creates sufficient flow of CSF to prevent the valve from obstruction on the one hand, and on the other hand, the valve’s gravitational unit counteracts overdrainage in the vertical position. However, the challenge of the DSV is to select the optimal opening pressure of the low pressure unit for the needs of the different hydrocephalus etiologies to avoid underdrainage [Citation9]. Müggenburg et al. [Citation57] showed in their investigation that multiple valve setting adjustments with a valve pressure adjustment rate of 38.3% are common.
A prospective, non-controlled, multicenter, observational study initiated by Sprung et al. [Citation10] evaluated the safety and reliability of the proGAV. The study included patients with hydrocephalus of all etiology. Out of the 165 enrolled patients, 21% were shunted because of secondary hydrocephalus. The opening pressure of the gravitational unit was chosen according to the body height of the patient and ranged from 20 to 35 cm H2O. Overall, the shunt survival rate of the proGAV shunt system in this study was favorable with a survival rate of 83% after 12 months. Infections (6.3%) and catheter revisions (7.2%) were leading reasons for surgical interventions. During the follow-up examinations overdrainage was diagnosed in 35 patients (21%). With the exception of one patient in whom ligation of the system became necessary, the remaining patients could be managed by valve readjustment. The authors concluded that the addition of the gravitational valve resulted in a low frequency of overdrainage and that a noninvasive readjustment of the valve is sufficient in most cases. However, the study did not target over-/underdrainage rates and the clinical outcome, particularly in patients with secondary hydrocephalus.
Ringel et al. [Citation48] compared programmable with standard valves for communicating hydrocephalus of adults in a retrospective study. Two hundred forty-seven programmable Codman–Hakim valves (CHP) (Codman & Shurtleff Inc./Johnson & Johnson Co, Raynham, MA) and 160 Hakim differential pressure valves (H) (NMT Neurosciences GmbH, Frankfurt, Germany) were implanted each without gravitational or anti-Siphon unit. The CHP group noted subdural fluid collections due to overdrainage in 8% (21/247) of the cases, and in the H group 3% (5/169) subdural fluid collections were observed. Surgical revision rates in the CHP and H groups were 2.5% (n = 6) and 2.5% (n = 4), respectively. The presented study focused on shunt revisions and valve reprogramming due to radiological signs of overdrainage, disregarding clinical symptoms of overdrainage. This could have resulted a lower number of cases with overdrainage being detected.
In contrast, Pollack et al. [Citation47] compared the CHP without siphon control mechanism and conventional valves (Delta (Medtronic PS Medical, Goleta, CA), other Medtronic PS Medical, other Codman/Johnson & Johnson, Orbis Sigma (Cordis, Miami Lakes, FL), other not specified valves) in a prospective randomized study including patients with communicating and noncommunicating hydrocephalus. The main endpoints of the study were survival of the shunt system and valve, under-/overdrainage, neurological deficit, foreign body reaction and infection. Three hundred seventy-seven patients (194 experimental, 183 control) were randomized into the clinical study. Only 48% of the shunts in both groups survived the 2-year study interval without replacement of any component [Citation47]. However, the study discovered no major differences in shunt survival among these heterogenous groups of valves. Infection occurred in the experimental and control group with 10,8% and 8.7%. Other complications were reported in 1% or less for both groups. The authors concluded that due to the lack of significant differences between this heterogenous group of shunt systems, the impact of valve selection is probably not essential [Citation47,Citation58].
Farahmand et al. [Citation58] implanted ligated VPS with membrane controlled ASDs together with an ICP probe in 15 patients with communicating hydrocephalus. The authors opened the ligation and stepwise adjusted the valve from performance level 2.5 to 0.5 in 4 h intervals. Simultaneously, the ICP was monitored in supine, sitting and walking position, respectively. The authors measured ICP changes into the expected direction but the absolute ICP differences between the pressure settings were lower than expected.
In a retrospective investigation, the authors [Citation41] followed a different strategy regarding the use of adjustable and nonadjustable gravitational shunt units. Non-gravitational shunt systems were used primarily for all hydrocephalus patients. Gravitational shunt units were only been implanted as part of the shunt revision management policy. The most commonly etiologies of hydrocephalus included werepediatric and idiopathic intracranial hypertension (IIH). Accordingly, the results showed high rates of revision and hospitalization [Citation41]. For this subgroup of hydrocephalus patients, the authors observed a significant reduction of surgical revisions and a decrease in the rate of hospitalizations after the implantation of proGAV with a fixed SA or proSA. However, they observed no impact of the gravitational units on clinical outcome or shunt survival for the general hydrocephalus group. The authors concluded that the proSA – valves should be considered in the case of complex shunt patients and not as a superior first-line shunt valve for a broad mix of patients [Citation41].
Tschan et al. [Citation42] reported on the clinical experience of the proSA in 64 patients (aged 1.8–41.4 years) with manifest overdrainage and unsuccessful conservative treatment efforts in the past. A total of 136 adjustments were required to achieve good clinical outcomes. Fourteen complications were observed, however, none of them were associated with the proSA valve. Ninety-one percent of patients reported that their symptoms had completely resolved after 1 year of proSA augmentation despite the heterogeneous group of hydrocephalus etiologies and their complicated shunt history.
There is a lack of prospective trials comparing the adjustable DP valves with additional gravitational units for patients with secondary hydrocephalus. Usually, in the case of overdrainage the opening levels of the adjustable DP valves were increased. This maneuver can overcome the overdrainage situation but leads to insufficient drainage that affects the clinical outcome. An additional gravitational unit could be a solution to the problem but there is a need for future studies. At this point, adjustable gravitational valves appear to be reserved for patients with complicated shunt histories.
1.3.3. Idiopathic normal-pressure hydrocephalus
In contrast to secondary hydrocephalus, the use of gravitational valves in iNPH has been well investigated by prospective and retrospective studies. Confirmed iNPH is preferably treated using VP shunting [Citation59–61]. The success rate after implantation of a VP shunt is up to 90% [Citation62,Citation63]. However, the success rate seems to decrease over the long term [Citation62,Citation64].
The Dutch Hydrocephalus Study showed that for iNPH patients low-pressure valves lead to better neurological outcomes than medium-pressure valves but are associated with a higher risk of chronic subdural effusions [Citation65]. In patients with an overdrainage event, however, no difference was found in the outcome. The authors advocated to use low- pressure valves for iNPH patient’s in combination with anti-siphon devices to reduce CSF flow in the upright position.
Therefore, a multicenter randomized study (SVASONA) on VP shunts either with gravitational or without gravitational valves was conducted in patients with iNPH [Citation66]. Based on the highly significant risk difference seen in overdrainage events, the investigators decided to discontinue the study at the time of 145 enrolled patients. The overall overdrainage rate was 3%. The authors therefore concluded that the option of choice for patients with iNPH is a gravitational valve. Additionally, the authors mentioned that the choice of the appropriate opening pressure of the gravitational unit remains crucial and should allow to minimize the overdrainage rate. The authors argued that the level of compensation for hydrostatic pressure depends on the height of the upper body and the peritoneal pressure. However, the peritoneal pressure is difficult to predict and may change over time.
Suchorska et al. [Citation67] compared in a retrospective analysis differential pressure valves (Codman Medos programmable valve, CMPV) and gravitational devices (proGAV) for the treatment of patients with iNPH. They exclusively implanted differential pressure valves from 1995 to 2005, then switched to gravitational devices since 2005. Four (8,1%) of the 49 included patients in the gravitational valve group developed subdural hygroma, which could be remedied by increasing the valve pressure without further surgery. In the differential pressure valve group, ten (25%) of 40 patients developed subdural hygroma, five of whom required surgery. However, no significant difference in the complication rate was found between the gravitational and differential pressure valve groups (p = 0.07). The clinical outcome measured using the Kiefer score and the black scale significantly favored of the gravitational valve group (p < 0.0001; p = 0.002).
Clinical studies about non-gravitational anti-siphon-devices are very rare. The investigators [Citation68] of a prospective double-blinded, randomized, controlled, dual-center study reevaluated the findings of the Dutch NPH study questioning how to use adjustable valves safely with optimal clinical effect. Whilst CMPV were implanted in all patients, one of the two study centers (Gothenburg) used the SiphonGuard antisiphon device and the other center (Oslo) did not use any ASD. Four (11,7%) of 34 patients with a gradual decreasing valve setting regime and five (14,7%) of 34 patients with fixed valve settings developed SDH. Surgical treatment of SDH was necessary in four of the patients. Five patients were treated by increasing the valve setting. Overdrainage symptoms were reported without significant difference in seven (23%) patients in the adjusted setting valve group and four (12%) in the control group. The results showed that the regime of gradually reducing valve pressure in terms of complication rates from an initially fixed setting was not more favorable. With a valve setting of ≤ 12 cm H2O compared to> 12 cm H2O, more overdrainage symptoms were observed (p = 0.016). However, the clinical outcome of the patients in the group with gradual deceased valve pressure improved significantly (p = 0.032) [Citation68]. The coauthor Farahmand et al. [Citation69] from Gothenburg published the results of the prospective trial which was the second study center and differed in methods. These results showed no clinical improvement after gradual reduction of the valve setting from 20 to 4 cmH2O compared with a fixed valve setting of 12 cm H2O [Citation69]. The SiphonGuard showed no protection against subdural hematomas (16%) compared to valves without an ASD (17%). More overdrainage symptoms were observed in patients without an ASD (50% vs. 9%; p = 0.002) [Citation68]. It should be noted that the main goal of this study was not to examine the effect of ASD on the complication rate and clinical outcome.
A prospective multicenter registry investigating the programmable gravitational unit (proSA) was initiated by Kehler et al. [Citation16] to evaluate the safety and reliability of the valve. One-hundred and twenty patients with hydrocephalus were included and followed up for 12 months. The heterogeneous group included hydrocephalus etiologies such as idiopathic normal-pressure hydrocephalus (n = 30), chronic communicating hydrocephalus (n = 47), obstructive hydrocephalus (n = 17), hydrocephalus due to CNS malformations (n = 12), pseudotumor cerebri (n = 9), and others (n = 5) [Citation16]. In 38 patients (32%), the pro SA was inserted during the first shunt implantation, in 82 patients (68%) the proSA was implanted during a shunt revision surgery [Citation16]. At the end of the study, the outcome was considered improved in 87% of the patients. The shunt survival rate was 89%. In total 13 patients had to undergo revision surgery due to infection (n = 2), CSF underdrainage (n = 3) and overdrainage (n = 3), and others (n = 5). Eighty-two readjustments were performed in 46 patients. Subdural effusions or hematomas were observed in six patients (7.9%), whereas only 76 patients had a postoperative CT/MRI scan due to complaints. Overdrainage-related shunt revision was necessary in three patients in whom readjustment of the proSA unit was not sufficient [Citation16]. The study showed that the proSA is a safe gravitational valve with a low complication rate. However, the study design does not adequately answer the question of the clinical benefit of such a complex gravity valve as the proSA for patients with iNPH nor whether the valve remains reserved for complex hydrocephalus with a previous unsuccessful shunt performance.
Scientific evidence is sufficient to clearly recommend implantation of gravitational valves as first-line treatment of iNPH. So far, it is uncertain whether the use of adjustable gravitation valves (proSA) is required for the initial treatment of iNPH or if it is reserved for revision surgery in complex hydrocephalus cases.
1.3.4. Ongoing clinical trials
A multicenter randomized trial about the efficacy and safety of programmable compared with fixed anti-siphon devices for treating idiopathic normal-pressure hydrocephalus (iNPH) in adults (SYGRAVA) is ongoing. Three-hundred and six patients are planned to be recruited. Patients are randomly selected 1: 1 to receive either a proGAV plus proSA or a Medtronic PS Medical Strata II with Delta Chamber or a Codman Certas plus with SiphonGuard. The follow-ups are planned for 3, 6 and 12 months. The main endpoints of the study are over-and underdrainage. An interim analysis is planned after recruitment of half of the patients, during which the investigators can decide about necessary protocol adjustments or the premature termination of the study. Currently, the recruitment of participants is ongoing.
2. Conclusion
The results of the in-vitro testing and the knowledge of the specific flow characteristics of each gravitational device give the neurosurgeon the opportunity to choose an appropriate device for each hydrocephalus patient.
For patients with secondary hydrocephalus, there is a lack of prospective trials comparing solely adjustable DP valves and with additional gravitational units. Usually, in the case of overdrainage the opening levels of the adjustable DP valves were increased. This maneuver can overcome the overdrainage situation but leads to insufficient drainage that affects the clinical outcome. An additional fixed gravitational unit (proGAV) could be a solution to the problem but there is a need for future studies. At this point, adjustable gravitational valves (proSA) appear to be reserved for patients with complicated shunt histories due to secondary hydrocephalus.
The evidence for patients with iNPH is sufficient to clearly recommend implantation of gravitational valves (proGAV) during the first-line treatment. So far, it is uncertain whether the use of adjustable gravitation valves (proSA) is required for the initial treatment of iNPH or if it is reserved for revision surgery in complex hydrocephalus cases.
Our review focuses on gravitational valves available on the market as represented in the published literature. In addition to gravitational valves, flow-regulated and membrane-controlled anti-siphon devices are used to treat hydrocephalus and to prevent over-drainage in an upright position through different mechanism. There is no consensus as to which concept of anti-siphoning by the various manufacturers is superior and should be the primary treatment option for patients with hydrocephalus.
3. Expert opinion
Shunt surgery remains a strikingly effective therapy in comparison to the understanding of the pathology of hydrocephalus. The rate of general surgical complications has been lowered to neglectable values in our days and the efficacy of shunt surgery for the treatment of hydrocephalus at all has been proven on a high methodological level [Citation61]. The goal of shunt surgery is to simulate the physiological CSF drainage characteristics. A major characteristic is the maintenance of a physiological ICP adapted to different body positions. Various solutions are developed in order to fulfill this goal. Gravitational valves are excellent engineering results since the hydrostatic effect in the patient is counteracted in the device by the same vector. Safety and efficacy of gravitational valves have been proven soon after its commercial availability [Citation10]. The superiority of gravitational valves has been demonstrated for the therapy of Normal-Pressure Hydrocephalus and at least in Europe, gravitational valves have more and more become the standard therapy for NPH [Citation66]. For pediatric patients high-level evidence publications proving that gravitational valves improve the quality of treatment as well as the potential for neurocognitive development are missing. These results are more difficult to accomplish since long-term follow-up studies are warranted. Nevertheless, also in pediatric patients gravitational valves have gained a high level of acceptance among treating neurosurgeons, which is expected to further increase in the coming years. Possible limitations of gravitational valves may include comatose patients with hydrocephalus after e.g. traumatic brain injury in which clinical symptoms of underdrainage are difficult to evaluate, as well as patients with low compliance and long-lasting treatment of overdrainage, who are adapted to low ICP pressure conditions. In those patients, the adjustability of the gravitational unit may play a bigger role in carefully adapting gravitational valves at low settings.
In general, gravitational valves have achieved a significant shift in market share for one manufacturer in Europe. This development can also be attributed to the effect of a relatively small selling market, high development and regulatory costs, as well as the engineering expertise developed for the complexity of hydrocephalus pathophysiology and its mechanical solution. For future development, the traditional understanding of hydrocephalus valve systems will further change. This may include that gravitational valves with the option of resistance adjustability will reach further acceptance in the treatment of hydrocephalus. The remote future of hydrocephalus valve technology will be represented by control loop systems with ICP monitoring and consecutive regulation of the CSF diversion. The initial technology for suchlike systems is available with telemetric ICP sensors and telemetric sensor shunt reservoirs. At the moment, electronic controlled valves integrating ICP measures are still missing, which may be expected as the next step of valve evolution. However, with the complexity of valve systems, the expenses will increase along, which will result in increasing challenges to apply sophisticated treatment options in a broad number of patients.
Declaration of interest
J Lemcke and U Thomale have previously received institutional grants from Aesculap-Miethke. The authors have no other relevant affiliations or financial involvement with any organization or entity with a financial interest in or financial conflict with the subject matter or materials discussed in the manuscript apart from those disclosed.
Reviewer disclosures
Peer reviewers on this manuscript have no relevant financial or other relationships to disclose.
Additional information
Funding
References
- Bradley KC. Cerebrospinal fluid pressure. J Neurol Neurosurg Psychiatry. 1970;33:387–397. PubMed PMID: 5431727.
- Petersen LG, Petersen JC, Andresen M, et al. Postural influence on intracranial and cerebral perfusion pressure in ambulatory neurosurgical patients. Am J Physiol Regul Integr Comp Physiol. 2016;310(1):R100–4. Epub 2015/ 10/16. PubMed PMID: 26468260.
- The scientific history of hydrocephalus and its treatment. 1999.
- Boockvar JA, Loudon W, Sutton LN. Development of the Spitz-Holter valve in Philadelphia. J Neurosurg. 2001;95(1):145–147. Epub 2001/ 07/17. PubMed PMID: 11453388.
- GROTTE G, NG LUNDBERG. Preliminary experiences with the Spitz‐Holter ventriculo‐caval shunt in the treatment of hydrocephalus. Acta Pædiatrica. 1961;50:617–628.
- The saga of the ‘Chhabra’ shunt, 2019.
- Czosnyka ZH, Czosnyka M, Richards HK, et al. Evaluation of three new models of hydrocephalus shunts. Acta Neurochir Suppl 2005;95:223–227. DOI:10.1007/3-211-32318-x_46.
- Sprung C, Miethke C, Shaken K, et al. The importance of the dual-switch valve for the treatment of adult normotensive or hypertensive hydrocephalus. Eur J Pediatr Surg. 1997 Dec;7 Suppl 1:38–40. DOI:10.1055/s-2008-1071208.
- Sprung C, Miethke C, Schlosser HG, et al. The enigma of underdrainage in shunting with hydrostatic valves and possible solutions. Acta Neurochir Suppl. 2005;95:229–235. DOI:10.1007/3-211-32318-x_47.
- Sprung C, Schlosser HG, Lemcke J, et al. The adjustable proGAV shunt: A prospective safety and reliability multicenter study. Neurosurgery. 2010;66:465–474.
- Meier U, Lemcke J. Is it possible to optimize treatment of patients with idiopathic normal pressure hydrocephalus by implanting an adjustable Medos Hakim valve in combination with a Miethke shunt assistant? Acta Neurochir Suppl. 2006;96:381–385. Epub 2006/ 05/05. PubMed PMID: 16671490.
- Freimann FB, Kimura T, Stockhammer F, et al. In vitro performance and principles of anti-siphoning devices. Acta Neurochir (Wien). 2014;156:2191–2199.
- Gebert AF, Schulz M, Schwarz K, et al. Long-term survival rates of gravity-assisted, adjustable differential pressure valves in infants with hydrocephalus. J Neurosurg Pediatr. 2016;17(5):544–551. Epub 2016/ 01/23. PubMed PMID: 26799410.
- Haberl EJ, Messing-Juenger M, Schuhmann M, et al. Experiences with a gravity-assisted valve in hydrocephalic children. Clinical article. J Neurosurg Pediatr. 2009;4(3):289–294. Epub 2009/ 09/24. PubMed PMID: 19772417.
- Aschoff A, Kiefer M, Kehler U, et al. Adjustable gravitational valves. From the conception in 1996 to first implantations 2008. Cerebrospinal Fluid Res. 2009. DOI:https://doi.org/10.1186/1743-8454-6-s2-s22
- Kehler U, Kiefer M, Eymann R, et al. PROSAIKA: a prospective multicenter registry with the first programmable gravitational device for hydrocephalus shunting. Clin Neurol Neurosurg. 2015;137:132–136. Epub 2015/ 07/22. PubMed PMID: 26196478.
- Czosnyka M, Czosnyka Z, Whitehouse H, et al. Hydrodynamic properties of hydrocephalus shunts: united kingdom shunt evaluation laboratory. J Neurol Neurosurg Psychiatry. 1997;62:43–50. PubMed PMID: 9010399.
- Kimura T, Schulz M, Shimoji K, et al. In vitro performance of the fixed and adjustable gravity-assisted unit with and without motion—evidence of motion-induced flow. Acta Neurochir (Wien). 2016;158:2011–2018.
- Czosnyka M, Czosnyka ZH, Pickard JD. Programmable shunt assistant tested in Cambridge shunt evaluation laboratory. Acta neurochirurgica/Supplementa. 2011;2011:77–81.
- Allin DM, Czosnyka ZH, Czosnyka M, et al. In vitro hydrodynamic properties of the Miethke proGAV hydrocephalus shunt. Cerebrospinal Fluid Res. 2006. DOI:https://doi.org/10.1186/1743-8454-3-9
- Gehlen M, Eklund A, Kurtcuoglu V, et al. Comparison of anti-siphon devices-how do they affect CSF dynamics in supine and upright posture? Acta Neurochir (Wien). 2017;159(8):1389–1397. Epub 2017/ 07/01. PubMed PMID: 28660395.
- Stockhammer F, Miethke C, Knitter T, et al. Flow-related noise in patients with ventriculoperitoneal shunt using gravitational adjustable valves. Acta Neurochir (Wien). 2014;156(4):761–765. Epub 2013/ 09/21. PubMed PMID: 24048819.
- Park J, Kim GJ, Hwang SK. Valve inclination influences the performance of gravity-assisted valve. Surg Neurol. 2007;68(1):14–8;discussion 8. Epub 2007/ 06/26. PubMed PMID: 17586212.
- Faulhauer K, Schmitz P. Overdrainage phenomena in shunt treated hydrocephalus. Acta Neurochir (Wien). 1978;45(1–2):89–101. Epub 1978/ 01/01. PubMed PMID: 742440.
- Gruber R. The relationship of ventricular shunt complications to the chronic overdrainage syndrome: a follow-up study. Zeitschrift fur Kinderchirurgie: organ der Deutschen, der Schweizerischen und der Osterreichischen Gesellschaft fur Kinderchirurgie = Surgery in infancy and childhood. 1981;34(4):346–352. Epub 1981/ 12/01. PubMed PMID: 7331544.
- Hoppe-Hirsch E, Sainte Rose C, Renier D, et al. Pericerebral collections after shunting. Child’s Nerv Syst. 1987;3(2):97–102. Epub 1987/ 01/01. PubMed PMID: 3621234.
- Belliard H, Roux FX, Turak B, et al. The Codman Medos programmable shunt valve. Evaluation of 53 implantations in 50 patients. Neurochirurgie. 1996;42(3):139–45;discussion 45–6. Epub 1996/ 01/01. PubMed PMID: 9084740.
- Serlo W. Experiences with flow-regulated shunts (Orbis-Sigma valves) in cases of difficulty in managing hydrocephalus in children. Child’s Nerv Syst. 1995;11(3):166–169. Epub 1995/ 03/01. PubMed PMID: 7773977.
- Watson DA. The delta valve: a physiologic shunt system. Child’s Nerv Syst. 1994;10(4):224–230. Epub 1994/ 05/01. PubMed PMID: 7923231.
- Meling TR. Gravitational shunt. J Neurosurg. 2007;107(4Suppl):342;author reply −3. Epub 2007/ 10/19. PubMed PMID: 17941503.
- Eymann R, Steudel WI, Kiefer M. Pediatric gravitational shunts: initial results from a prospective study. J Neurosurg. 2007;106(3Suppl):179–184. Epub 2007/ 05/01. PubMed PMID: 17465381.
- Sokratous G, Hadfield O, Van Tonder L, et al. Management of paediatric hydrocephalous with Miethke fixed pressure gravitational valves. The Alder Hey children’s hospital experience. Child’s Nerv Syst. 2020;36:2021–2025. Epub 2020/ 02/06. PubMed PMID: 32020268.
- Weinzierl MR, Hans FJ, Stoffel M, et al. Experience with a gravitational valve in the management of symptomatic overdrainage in children with shunts. J Neurosurg Pediatr. 2012;9(5):468–472. Epub 2012/ 05/02. PubMed PMID: 22546023.
- Rohde V, Haberl EJ, Ludwig H, et al. First experiences with an adjustable gravitational valve in childhood hydrocephalus. J Neurosurg Pediatr. 2009;3(2):90–93. Epub 2009/ 03/13. PubMed PMID: 19278305.
- Thomale UW, Gebert AF, Haberl H, et al. Shunt survival rates by using the adjustable differential pressure valve combined with a gravitational unit (proGAV) in pediatric neurosurgery. Child’s Nerv Syst. 2013;29(3):425–431. Epub 2012/ 11/09. PubMed PMID: 23135777.
- Gebert AF, Schulz M, Haberl H, et al. Adjustments in gravitational valves for the treatment of childhood hydrocephalus-a retrospective survey. Child’s Nerv Syst. 2013;29(11):2019–2025. Epub 2013/ 05/30. PubMed PMID: 23715809.
- Xinxing L, Hongyu D, Yunhui L. Using individualized opening pressure to determine the optimal setting of an adjustable proGAV shunt in treatment of hydrocephalus in infants. Child’s Nerv Syst. 2015;31(8):1267–1271. Epub 2015/ 07/06. PubMed PMID: 26143276.
- Alavi S, Schulz M, Schaumann A, et al. Valve exchange towards an adjustable differential pressure valve with gravitational unit, clinical outcome of a single-center study. Child’s Nerv Syst. 2017;33(5):759–765. Epub 2017/ 03/24. PubMed PMID: 28332153.
- Al-Hakim S, Schaumann A, Schneider J, et al. Experience in shunt management on revision free survival in infants with myelomeningocele. Child’s Nerv Syst. 2018;34(7):1375–1382. Epub 2018/ 03/28. PubMed PMID: 29582171.
- Bock HC, Kanzler M, Thomale UW, et al. Implementing a digital real-time hydrocephalus and shunt registry to evaluate contemporary pattern of care and surgical outcome in pediatric hydrocephalus. Child’s Nerv Syst. 2018;34(3):457–464. Epub 2017/ 11/11. PubMed PMID: 29124391.
- Månsson PK, Hansen TS, Juhler M. The applicability of fixed and adjustable gravitational shunt valves in two different clinical settings. Acta Neurochir (Wien). 2018;160(7):1415–1423. Epub 2018/ 05/29. PubMed PMID: 29804178.
- Tschan CA, Antes S, Huthmann A, et al. Overcoming CSF overdrainage with the adjustable gravitational valve proSA. Acta Neurochir (Wien). 2014;156(4):767–76;discussion 76. Epub 2013/ 12/03. PubMed PMID: 24292775.
- Ludwig HC, Reitemeyer M, Bock HC, et al. Hydrocephalus shunt therapy: current titanium shunt valve implants obstructed by internal tissue proliferations identified as extracellular matrix membranes. Childs Nerv Syst. 2020 Nov;36(11):2717–2724. DOI:10.1007/s00381-019-04467-8 . Epub 2019/ 12/18. PubMed PMID: 31845028.
- Bir SC, Patra DP, Maiti TK, et al. Epidemiology of adult-onset hydrocephalus: institutional experience with 2001 patients.
- Connolly ES, Rabinstein AA, Carhuapoma JR, et al. Guidelines for the management of aneurysmal subarachnoid hemorrhage: A guideline for healthcare professionals from the american heart association/american stroke association. Stroke. 2012;43:1711–1737. PubMed PMID: 22556195.
- Kay AD, Fisher AJ, O’Kane C, et al. A clinical audit of the Hakim programmable valve in patients with complex hydrocephalus. Br J Neurosurg. 2000;14:535–542.
- Pollack IF, Albright AL, Adelson PD. A randomized, controlled study of a programmable shunt valve versus a conventional valve for patients with hydrocephalus. Neurosurgery. 1999;45:1399–1411.
- Ringel F, Schramm J, Meyer B. Comparison of programmable shunt valves vs standard valves for communicating hydrocephalus of adults: A retrospective analysis of 407 patients. Surg Neurol. 2005;63:36–41.
- Yamashita N, Kamiya K, Yamada K. Experience with a programmable valve shunt system. J Neurosurg. 1999;91:26–31.
- Kestle JRW, Walker ML. A multicenter prospective cohort study of the Strata valve for the management of hydrocephalus in pediatric patients. J Neurosurg. 2005;102 PEDIAT:141–145.
- Koschnitzky JE, Keep RF, Limbrick DD, et al. Opportunities in posthemorrhagic hydrocephalus research: outcomes of the hydrocephalus association posthemorrhagic hydrocephalus workshop. Fluids Barriers CNS. 2018;15:1–22.
- Katano H, Karasawa K, Sugiyama N, et al. Clinical evaluation of shunt implantations using Sophy programmable pressure valves: comparison with Codman-Hakim programmable valves. J Clin Neurosci. 2003;10:557–561.
- The Miethke dual switch valve: Experience in 169 adult patients with different kinds of hydrocephalus: An open field study, 2008.
- Kiefer M, Eymann R, Meier U. Five years experience with gravitational shunts in chronic hydrocephalus of adults. Acta Neurochir (Wien). 2002;144:755–767.
- Freimann FB, Sprung C. Shunting with gravitational valves - Can adjustments end the era of revisions for overdrainage-related events? Clinical article. J Neurosurg. 2012;117:1197–1204.
- Pudenz RH, Foltz EL. Hydrocephalus: overdrainage by ventricular shunts. A review and recommendations. Surg Neurol. 1991;35:200–212.
- Müggenburg L, Behmanesh B, Dinc N, et al. Prevalence and indication for changing the primary valve opening pressure in ventriculoperitoneal shunts – A single center five years overview. Clin Neurol Neurosurg. 2019;186:105523.
- Farahmand D, Qvarlander S, Malm J, et al. Intracranial pressure in hydrocephalus: impact of shunt adjustments and body positions. J Neurol Neurosurg Psychiatry. 2015;86(2):222–228. Epub 2014/ 06/26. PubMed PMID: 24963125.
- Halperin JJ, Kurlan R, Schwalb JM, et al. Practice guideline: idiopathic normal pressure hydrocephalus: response to shunting and predictors of response: report of the guideline development, dissemination, and implementation subcommittee of the American academy of neurology. Neurology. 2015;85(23):2063–2071. Epub 2015/ 12/09. PubMed PMID: 26644048; PubMed Central PMCID: PMCPMC4676757.
- Hebb AO, Cusimano MD. Idiopathic normal pressure hydrocephalus: a systematic review of diagnosis and outcome. Neurosurgery. 2001;49:1166. PubMed PMID: 11846911.
- Kazui H, Miyajima M, Mori E, et al. Lumboperitoneal shunt surgery for idiopathic normal pressure hydrocephalus (SINPHONI-2): an open-label randomised trial. Lancet Neurol. 2015;14:585–594. PubMed PMID: 25934242.
- Kahlon B, Sjunnesson J, Rehncrona S. Long-term outcome in patients with suspected normal pressure hydrocephalus. Neurosurgery. 2007;60:327–332.
- Razay G, Vreugdenhil A, Liddell J. A prospective study of ventriculo-peritoneal shunting for idiopathic normal pressure hydrocephalus. J Clin Neurosci. 2009;16:1180–1183. PubMed PMID: 19497748.
- Gutowski P, Rot S, Fritsch M, et al. Secondary deterioration in patients with normal pressure hydrocephalus after ventriculoperitoneal shunt placement: A proposed algorithm of treatment. Fluids Barriers CNS. 2020;17:1–9.
- Boon AJW, Joseph TJ, Delwel EJ, et al. Dutch normal-pressure hydrocephalus study: randomized comparison of low- and medium-pressure shunts. J Neurosurg. 1998;88:490–495.
- Lemcke J, Meier U, Müller C, et al. Safety and efficacy of gravitational shunt valves in patients with idiopathic normal pressure hydrocephalus: A pragmatic, randomised, open label, multicentre trial (SVASONA). J Neurol Neurosurg Psychiatry. 2013 Aug;84(8):850–857. DOI:10.1136/jnnp-2012-303936. Epub 2013 Mar 1.
- Suchorska B, Kunz M, Schniepp R, et al. Optimized surgical treatment for normal pressure hydrocephalus: comparison between gravitational and differential pressure valves. Acta Neurochir (Wien). 2015;157:703–709.
- Sæhle T, Farahmand D, Eide PK, et al. A randomized controlled dual-center trial on shunt complications in idiopathic normal-pressure hydrocephalus treated with gradually reduced or fixed pressure valve settings. J Neurosurg. 2014;121:1257–1263.
- Farahmand D, Sæhle T, Eide PK, et al. A double-blind randomized trial on the clinical effect of different shunt valve settings in idiopathic normal pressure hydrocephalus. J Neurosurg. 2016;124:359–367.