ABSTRACT
Background: Treating chronic pain using sub-perception Spinal Cord Stimulation (SCS) does not elicit paresthesia but is associated with long analgesic ‘wash-in’ (i.e. duration until maximum pain relief) and prolonged assessment of therapy. We describe the attainment of clinically meaningful and rapid-onset analgesic outcomes using a novel sub-perception SCS approach.
Methods: This observational case-series evaluated patients implanted with an SCS device for chronic pain, who underwent re-programming utilizing a new methodology in which paresthesia was used to guide sub-perception stimulation field targeting at specific parameters including charge-balanced symmetrical pulses at 90 Hz (termed Fast-Acting Sub-Perception Therapy, FAST). Pain scores (NRS) were collected as reported per standard-of-care from patient charts.
Results: Mean overall pain score at baseline was 8.4 ± 0.2 (n = 41). After activation of FAST, a 7.1-point reduction in overall pain score was (1.3 ± 0.2, p < 0.0001) reported within 11.2 ± 1.9 minutes (n = 34). This decrease in pain score was sustained out to 3-month (1.6 ± 0.3, n = 26) and 6-month follow-up (1.7 ± 0.4, n = 18). At last follow up (mean = 223 ± 132 days), a pain score of 1.6 ± 0.3, n = 30 was determined.
Conclusions: After FAST implementation, a profound analgesic response, requiring substantially less energy than conventional sub-perception methodologies, was observed. This rapid analgesic onset achieved with the novel FAST technique suggests the potential for an alternative mechanism of action(s) of sub-perception SCS.
1. Introduction
Paresthesia-based spinal cord stimulation (SCS) has been used for decades to treat chronic pain. Historically, antidromic activation of the nerve fibers in the dorsal columns has been thought by some to be capable of ‘closing the gate’ resulting in analgesia, while orthodromic activation elicits paresthesias [Citation1]. The relationship between the induction of paresthesia and analgesia was therefore assumed to be linked given that the location of pain relief has been demonstrated to correlate with paresthesia overlap [Citation2]. However, nearly 40 years after the inception of SCS, Yearwood, and Foster described SCS cases wherein analgesia was achieved at amplitudes below the paresthesia threshold, and current SCS approaches now exist that do not produce paresthesia, variously described as ‘paresthesia-free’, ‘paresthesia-independent’, or ‘sub-perception’ SCS [Citation3–6]. Intriguingly, sub-perception SCS yields a slower onset of analgesia after turning on stimulation (typically several hours to days) in contrast to paresthesia-based SCS in which analgesia is usually observed within minutes [Citation7–10]. This has contributed to various hypotheses regarding potential mechanisms of action that mediate pain relief produced by sub-perception-based approaches [Citation10–12].
Notwithstanding these mechanistic questions, the consequences for patients of a protracted ‘wash-in’ time (i.e. duration until maximum analgesia) and other drawbacks associated with current sub-perception methodologies are potentially onerous. In particular, therapy program optimization is likely to be prolonged (e.g. evaluating any one parameter set requires ~1-2 days) as well as technically compromised (i.e. the number of programming settings that can be practically tested is limited) [Citation9,Citation11]. Because of these properties, patients utilizing conventional sub-perception techniques do not typically receive pain relief during their programming visit and the effectiveness of the treatment cannot be immediately assured. Moreover, methods that deliver sub-perception SCS at higher frequencies (e.g. 1–10 kHz) often demand more energy and require more frequent recharging [Citation9,Citation10].
In previous studies, we demonstrated that sub-perception SCS can be effective using frequencies down to 10 Hz and when perception thresholds were tested in patients assessed in these analyses, the area of their pain was at times observed to overlap with their sensation of paresthesia [Citation9,Citation13]. This prompted us to consider whether paresthesia, as a physiological response indicative of spinal organization, could serve as a marker for targeting of sub-perception SCS [Citation14]. To evaluate this further, we steered paresthesia at high resolution (~300 µm) on tightly spaced leads at a frequency of 90 Hz using a pulse width (210 ± 50 µs; effective for low-frequency sub-perception SCS) and at the lowest practical amplitude capable of inducing paresthesia with a comprehensive overlap of pain areas [Citation13,Citation15]. Unexpectedly, when the amplitude was reduced below the perception threshold, a majority of patients were observed to achieve pain relief within minutes following SCS therapy activation. In light of these initial observations, we speculated the possibility of a differing mechanism of action versus that which has been classically proposed as the plausible mechanism(s) underlying sub-perception SCS and sought to assess an expanded number of patients using this novel methodology that we henceforth term Fast-Acting Sub-Perception Therapy (FAST). Here, we provide early evidence of FAST therapy outcomes on the basis of our observations of 41 SCS-implanted patients who utilized FAST for the treatment of chronic back and/or leg pain.
2. Patients and methods
2.1. Study design
This multicenter observational case-series (Clinicaltrials.gov ID: NCT01550575) was carried out based on a retrospective chart review of 41 consecutive patients who were previously implanted ‘on-label’ (i.e. FDA approved) with a permanent SCS system (Spectra WaveWriter/Precision Spectra, Boston Scientific, Valencia, CA) using leads with up to 32 tightly spaced electrodes (~1 mm edge-to-edge; InfinionCX/LinearST/CoverEdge, Boston Scientific) for treatment of chronic pain of the lower back and/or lower limbs. Patients who utilized Fast-Acting Sub-Perception Therapy (FAST) to manage their pain were included in this study.
Institutional Review Board (IRB) approval was obtained from each site and study from Western IRB (Reference Number: WIRB/20120371), and the study was conducted in accordance with Good Clinical Practices (ISO14155) guidelines and the Declaration of Helsinki.
2.2. FAST methodology
All patients were asked to turn off their SCS system for at least 12 hours prior to SCS re-programming session, per standard-of-care (as applicable to participating centers). Patients had previously received an SCS system that enabled multiple independent current control (MICC) – i.e. each electrode on the lead has its own dedicated current source, and the system is equipped with a model-based programming algorithm that enables multiple central points of stimulation (CPS) to be moved rostrocaudally and mediolaterally simultaneously at high resolution (~300 µm steps) [Citation15,Citation16]. Patients included in this retrospective analysis were programmed using a frequency of 90 Hz and pulse width of 210 ± 50 µs with a symmetric biphasic waveform. By using symmetric biphasic waveforms two separate CPSs are implemented in the stimulation paradigm, one for each rectangular phase of the charge-balanced stimulation cycle. During the first rectangular phase of the biphasic stimulating waveform, negative current is injected through negatively configured contacts (‘cathodes’), and positive current is injected through positively configured return contacts (‘anodes’). During the second rectangular phase, the polarities are reversed to achieve charge balance (i.e. the current is anodic at the assigned cathode and vice versa) thereby implementing a second CPS in the anodic region (). The settings were selected in accordance with a prior study, per standard-of-care, that identified pulse-width frequency combinations effective for sub-perception SCS, and selection of 90 Hz for physiological (paresthesia) mapping [Citation13]. Leads were placed utilizing standard technique per the preference of implanting physician within the typical range of T7-T10 vertebrae. To identify the electrode configuration and fine-tune the location of stimulation, a neural target search was carried out using two CPSs (8–16 mm apart) steered simultaneously (see ) in the rostro-caudal and medial-lateral dimensions using the ‘fine’ resolution setting of the algorithm (enabled shifts of the neurostimulation field in ~300 µm increments) [Citation15]. Additionally, the spread of paresthesia during targeting was controlled by modulating the distance between anodes and cathodes from 8 to 16 mm. This method allowed a systematic optimization of the stimulating field that provided comprehensive overlap between the area of pain and paresthesia sensation with amplitude just above perception threshold, and where that overlap was maintained under various body postures and common physical activities (e.g. walking, moving limbs). The amplitude was then lowered to approximately 65% of perception threshold. In our experience, there is a range and variability in the effective therapy intensity level for a given subject, therefore all patients were encouraged to evaluate, a daytime (40–70%) and a nighttime (20–40%) intensity level to account for posture and physical activity. All patients verbally communicated that they used their external controller to adjust intensity values within a range of 20–70% of their perception threshold, as recommended (minimum amplitude was locked at 10% and maximum was locked at 100% of perception threshold) to survey which values within this range provided the best pain relief. Lower amplitudes were recommended for use during sleep and higher amplitudes for more physically intense activity. All other stimulation parameters (e.g. frequency, pulse-width) were locked.
Figure 1. Submillimeter targeting with tightly spaced electrodes and multiple current sources
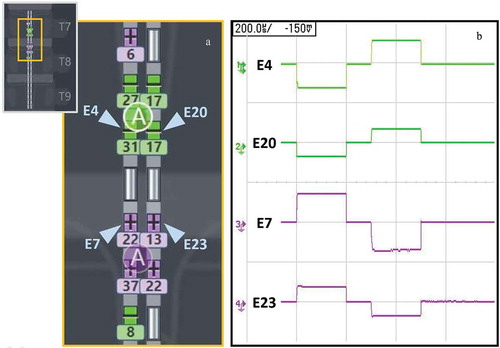
2.3. Data collection and analysis
Data related to demography, medical history, and pain location was collected as part of the chart review. Pain scores (NRS) related to overall pain, low back, and/or leg pain were collected by the site staff as part of their standard-of-care and routine follow-up. This information was thereafter entered by site personnel into the electronic database designed for collection of study data. To minimize potential bias, data collection was performed directly by clinical site personnel with no sponsor involvement. All data were collected by the site staff as part of routine care, both prior to re-programming with FAST and at the end of the visit. The time in which stimulation was turned off was recorded by querying each patient prior to the start of the FAST programming session. Additionally, the time of the activation of the specific FAST program utilized for therapy was recorded. Due to the retrospective nature of this study, no pre-established protocol was defined to measure the specific analgesia onset time following FAST program activation. However, the time difference between the activation of the FAST program utilized for therapy and the recording of the NRS pain score at the end of the visit (post-FAST activation) was calculated. To determine energy usage, mean charge-per-second (millicoulombs per second of charge delivered during the first phase of stimulation pulse) was calculated according to the following: amplitude (mA) × pulse width (μs) × SCS frequency (Hz). Study data collected at 3- and 6-month timepoints were only derived from patients who at the time of the data snapshot had reached these follow-up visits. Hence, the reduced number of those analyzed at 3- and 6-months occurred only because not all of the original 41 patients included at the start of this study had reached these follow-up timepoints, and not due to data that was missing or lost to follow-up. Descriptive statistics were used to define population characteristics (mean, standard deviation [SD], or standard error [SE]). Paired t‐test or Wilcoxon signed ranks test were used to assess differences, and P‐values of 0.05 were considered statistically significant. The normality of the distribution was assessed based on plots and Kolmogorov-Smirnov test.
3. Results
A total of 41 permanently implanted patients (average number of implanted days: 184) were identified and analyzed at Baseline and at Pre-/Post-Activation of FAST. Of these, 26 reached the 3-month follow-up and 18 reached the 6-month follow-up visit as shown in . displays patient baseline demographics. In summary, the mean age of patients was 57.8 ± 11.5 years at pre-implant and 58.5% of patients were female. A majority of patients received SCS for the indications of Failed Back Surgery Syndrome and/or Lumbosacral Radiculopathy (key diagnosis reported in ). Patients were implanted using a variety of available leads; however, all implanted leads were equipped with tightly spaced contacts with ~1 mm rostro-caudal spacing.
Table 1. Baseline demographic characteristics in analyzed patients (n/N)
The pre-implantation baseline overall NRS pain scores were available for all evaluated patients (n = 41) and the mean baseline NRS was 8.4 ± 0.2 (). Prior to activation of FAST and in the stimulation OFF state, the mean overall NRS pain score was 6.5 ± 0.3 ()). After activation of FAST, the overall pain was reduced by a mean 7.1 ± 0.4 points (versus pre-implantation baseline) and 5.2 ± 0.3 points (versus pre-FAST activation) to 1.3 ± 0.2 (n = 41, p < 0.0001). As compared with pre-FAST (stim OFF), a 4.8 ± 0.4 (n = 26, p < 0.0001) and 4.9 ± 0.5 (n = 18, p < 0.0001) NRS improvement in overall pain was noted at 3- and 6-months, respectively. The retrospective nature of this study did not afford the means to provide an exact measurement of the wash-in time. However, the time difference between the end-of-visit time (at which the NRS pain score was recorded) and the time of the initial activation of the FAST program (utilized for therapy) was recorded in each patient. A mean of 11.2 ± 1.9 minutes (n = 34) was noted which demonstrates that patients reported low pain scores (1.3 ± 0.2) rapidly post-FAST activation ()). This average time interval thus represents a likely upper bound value of the actual analgesic onset time which provides initial evidence that sub-perception-based pain relief using FAST can be obtained within a single programming visit. Mean overall pain NRS data at follow-up timepoints suggest that the magnitude of FAST-induced analgesia seen during the initial programming visit was successfully maintained out to 3- and 6-months (p < 0.0001) ()). At last follow up (mean = 223 ± 132 days), a pain score of 1.6 ± 0.3, n = 30 was also noted. Furthermore, the mean back pain NRS and mean leg pain only NRS were found to demonstrate a similar trend ( and ). The calculated mean charge-per-second using FAST (assuming 65% of perception threshold) was determined to be 0.05 ± 0.01 mC/s ()).
Figure 3. Pain reduction at initial programming session using FAST
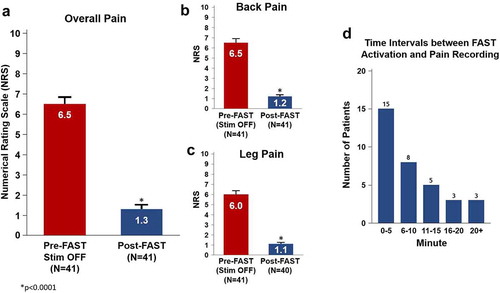
Figure 4. Pain reduction and charge expenditure using FAST
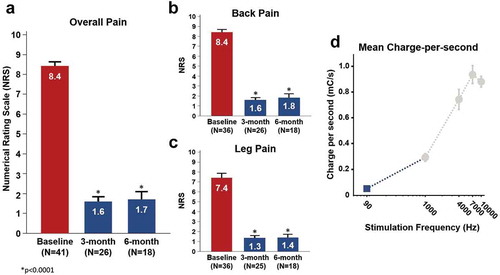
4. Discussion
To our knowledge, this is the first report from a multicenter, observational case-series to describe significant pain relief with rapid-onset using a sub-perception SCS methodology, now designated as Fast-Acting Sub-Perception Therapy (FAST). Importantly, the magnitude of this rapidly induced pain relief was sustained out to 3- and 6-month follow-up or up to patients’ last follow up. Further, we determined in this study that FAST demonstrated a comparatively lower amount of energy expenditure versus that previously reported using conventional sub-perception at 1–10 kHz [Citation9]. Based on our experience, the ability to systematically obtain fast-acting analgesia using a biphasic-symmetric waveform (i.e. active recovery/active recharge) at 90 Hz constitutes a novel observation as it relates to the clinical application of sub-perception SCS.
Paresthesia is a generally observable effect of SCS as traditionally applied at low frequency (<100 Hz), and its correspondence with the painful area has historically been considered a desirable target for such SCS therapy based on the belief that overlap of paresthesia with pain topography realizes engagement with functionally relevant circuits according to the classical ‘gate control’ theory via antidromic Aβ fiber activation [Citation1,Citation2,Citation14,Citation17]. However, this benefit appears to be lost using conventional sub-perception SCS methods, resulting in a time-consuming ‘trial-and-error’ electrode selection process during device programming. Remarkably, FAST was uncovered while attempting to evaluate whether paresthesia, identified using multiple central points of stimulation steered simultaneously at high-resolution (rostrocaudally and mediolaterally), might be an effective physiological response for use in the identification of the optimal site(s) at which to apply sub-perception SCS.
Proposed hypotheses that seek to elucidate the mechanisms of action (MOA) underpinning the pain-relieving effects of SCS are still generally evolving, and clinical observations associated with conventional sub-perception SCS approaches have raised additional mechanistic questions [Citation18,Citation19]. In particular, slower analgesic onset times, higher magnitudes of applied energy, and very low therapeutic amplitudes are aspects suggesting that other targets and mechanisms with longer time constants are likely involved in mediating the outcomes associated with the use of conventional sub-perception techniques [Citation18–20]. Our observations following the activation of FAST seem to provide for new mechanistic possibilities that suggest putative fast-acting mechanisms can be engaged at sub-perception amplitude levels and at comparatively low energies. As such, comprehensive hypotheses regarding candidate mechanisms that govern the therapeutic capabilities of sub-perception SCS must now incorporate and/or synthesize both fast-acting (such as direct neural effects) and slow-acting models (e.g. systemic modulation, gene transcription, protein translation, or glial cell coordinated pathways). Moreover, the observation that fast-acting, sub-perception analgesia can be systematically obtained using a physiologically based targeting methodology suggests that these elicited effects are dependent on the precise location of applied neurostimulation.
In this respect, of particular recent interest is the potential engagement of a mechanism known as ‘surround inhibition,’ which can occur through electrical activation of Aβ fibers or dorsal columns originating from receptive fields immediately surrounding the site of pain. This activation in turn leads to synaptic activation of inhibitory interneurons that suppress the transmission of nociceptive information relayed from the spinal circuits corresponding to the pain center – but, in contrast to Gate Control Theory, without exciting the center WDR neuron [Citation21,Citation22]. The engagement of surround inhibition by FAST offers a promising MOA for the therapy, as selectively engaging dorsal columns from the surround may overcome the excitatory effects of activating dorsal columns originating from the center – inhibition that would not be possible by stimulation of the pain center alone [Citation23]. Intriguingly, recent preliminary computational modeling suggests that capturing fewer dorsal column fibers than necessary for paresthesia may nonetheless still inhibit the center nociceptive projection neuron via inhibitory interneurons if those dorsal column fibers primarily originate from the surrounding receptive field, and this effect could not be reproduced if center dorsal column fibers were preferentially targeted [Citation24].
Groups using other modalities have used paresthesia guidance to achieve a measure of slow-acting sub-perception pain relief [Citation6,Citation25]. Still, other investigations have indicated that paresthesia may not be a requirement or useful for identifying a sub-perception stimulation target [Citation26,Citation27]. In the latter study by De Carolis et al., where the utility of paresthesia guidance was questioned, paresthesia at the location of effective sub-perception SCS was evaluated with very different parameters (60 Hz, ~470 µs) than those used for therapy (10 kHz, 30–40 µs). However, according to previously published reports, it is unlikely large changes in frequency and pulse width engage the same neural elements [Citation27–29]. Furthermore, very notable differences exist between how SCS was implemented using FAST in this study and how paresthesia was evaluated in De Carolis et al., including the following:
high-resolution placement of the stimulating electric field was enabled using tightly spaced electrodes (~1 mm), a multiple current source architecture (MICC), and an algorithm (using a biphasic symmetric waveform) that can incrementally shift stimulation in ~300 µm increments () [Citation15].
frequency (90 Hz) and pulse width (210 ± 50 µs) settings were applied in kind for both physiological mapping and therapy on the basis of prior evaluation of parameter settings found to be effective for sub-perception [Citation13].
shorter pulse widths were used for physiological mapping (210 ± 50 µs versus 470 µs) and these possibly yield a map that is more precise [Citation28,Citation29].
A mathematical modeling study from Lee et al. suggests that greater activation of smaller fibers would occur as pulse-width is increased [Citation29]. Since there is a greater relative fraction of smaller fibers in the medial aspects of the dorsal columns, the modeling results would predict greater paresthesia coverage in the lumbar and sacral dermatomes with increased pulse-width [Citation14]. In a companion clinical study, Yearwood et al. showed that increased pulse width can indeed lead to the recruitment of more caudal dermatomes and greater overall paresthesia coverage [Citation28]. The paresthesia-changing effect of increasing pulse width seems to be a ‘fiber steering’ one, whereby the use of wider pulse width enables smaller, more medial fibers to be recruited along with or prior to larger, more lateral fibers. At relatively short pulse-widths (as used in FAST) the caudal shift and the overall dermatomal ‘spillover’ of stimulation is limited and as postulated by Veizi et al. a neural targeting algorithm enables precise placement of the stimulation field on the patient-specific painful areas/sweet-spot [Citation15]. Additionally, the ability to adjust the spacing between the two CPSs may further increase the targeting precision and specificity by adjusting the ratio of dorsal root threshold to dorsal column threshold.
In this early evaluation, utilization of FAST was observed to circumvent some of the known limitations of conventional sub-perception methodologies. Conventional sub-perception methodologies do not require the patient to feel paresthesia – a property appreciated by some patients, but at the cost of some notable drawbacks compared to paresthesia SCS therapy. Firstly, because of the particularly slow wash-in time (hours to days), patients leave the initial programming session still awaiting relief and can only hope that one of the programs provided to them will be effective. Similarly, because of the lack of physiological feedback, physicians do not have certainty that leads are optimally placed or that programs will work. Secondly, the number of program settings testable is limited by pragmatic considerations, calling into question whether or not the final settings – even if effective to a degree – are truly optimal. Thirdly, in many cases, conventional sub-perception SCS programs require comparatively more energy than paresthesia-based programs, although recent work indicates that high energy is not an inherent property of sub-perception SCS [Citation13,Citation30]. In contrast, our experience using the novel FAST methodology indicates that rapid evaluation of several SCS settings is possible (a property demonstrated to be beneficial for achieving pain-paresthesia coverage in traditional SCS) and can result in effective analgesia during the programming session while substantially lowering charge-per-second usage (95% lower than using conventional sub-perception SCS at 10 kHz [0.88 ± 0.04 mC/s] and 83% lower than 1 kHz therapy [0.30 ± 0.07 mC/s]) [Citation9]. These data are also consistent with recent work evaluating sub-perception SCS across the frequency spectrum [Citation13]. Further, since FAST does not require a special lead placement, but rather uses the same placement that would be typically used for paresthesia-based therapy and other slow-acting sub-perception modalities, patients have access to other SCS modalities if deemed useful or desired.
This study does have limitations given its retrospective design. First, all patients evaluated had access and/or experienced SCS prior to study start (average number of implanted days prior to FAST activation: 184 days), and thus no established procedure was in place to measure any potential ‘carry-over’ based pain relief that may have occurred including after the use of the paresthesia-guided neural targeting procedure. However, given that the magnitude of pain relief was sustained out to 3- and 6-month follow-up, the likelihood of a carry-over effect from pre-FAST programming appears to be minimal. Secondly, there was no set protocol in place to document the exact measurement of the FAST-induced analgesic ‘wash-in’ time even though anecdotally all patients communicated rapid attainment of pain relief, within minutes. Finally, all patients included in this analysis reported a complete (or nearly complete) paresthesia overlap with the targeted area of pain. Whether FAST may still be effective even at sites that may have only partial overlap, and in circumstances in which a combination of other additional programs might be viably implemented, is not clear from our data.
On the basis of the results reported in this study, future clinical studies of FAST are now warranted to further validate and investigate this novel method. In particular, future studies will need to be designed to specifically measure FAST-induced analgesic onset time. Moreover, since the targeting, parameters, onset time, and energy consumption of FAST are very different than conventional sub-perception SCS approaches, it is possible that FAST might involve a different mechanism of action. As such, the clinical observations noted in this study may help to guide future investigations of FAST-specific stimulation parameters and settings, including the role that precise targeting of neurostimulation may have on the electrophysiological transmission of pain.
5. Conclusions
This multicenter, observational study provides the first report of a novel Fast-Acting Sub-Perception Therapy (FAST) observed to induce rapid and sustained analgesia in patients treated with SCS for chronic pain as measured out to 3- and 6-months or up to patients’ last follow up. In addition, FAST was observed to allow for confirmation of pain relief within a single clinic visit instead of lengthy programming optimization associated with conventional sub-perception techniques. This study also indicates that targeted neurostimulation using a high-resolution, paresthesia-guided, biphasic-symmetric waveform can be used to identify a patient-specific target for use as a physiological marker for paresthesia-free therapy. Furthermore, the fast-acting sub-perception nature of the modality and the potential spatial sensitivity raise important insights with regard to the ongoing investigations of mechanisms of action of SCS. These results therefore provide initial evidence that FAST constitutes a new approach in the delivery of sub-perception-based SCS therapy for treating patients with chronic pain.
Authorship contributions and data sharing
C Metzger, Q Doan, R Jain, M Moffitt, and L Annecchino conceived and designed the study. C Metzger, W Newton, JF Paz-Solis, and S Thomson and their staff carried out the study including collecting patient data. Q Doan developed the FAST methodology. L Annecchino and Q Doan conducted SCS programming sessions. Statistical data analysis was performed by Y Pei and R Jain. L Annecchino, M Moffitt, and Q Doan helped prepare the manuscript. All authors critically reviewed and approved the submitted manuscript.
The data, analytic methods, and study materials for this clinical study will be made available to other researchers in accordance with the Boston Scientific Data Sharing Policy (https://www.bostonscientific.com/).
Declaration of interest
C Metzger reports no relevant conflicts of interest. MB Hammond reports personal fees from NeuroMicroSpine and personal fees from Boston Scientific. JF Paz-Solis, W Newton, and S Thomson are consultants for Boston Scientific. All other remaining authors are salaried employees of Boston Scientific.
Reviewer disclosures
One peer reviewer has acted as a consultant for Abbott, Boston Scientific, and Nevro. One peer reviewer has acted as a consultant for Boston Scientific and Nevro. Peer reviewers on this manuscript have no other relevant financial relationships or otherwise to disclose.
Acknowledgments
The authors wish to express their great appreciation to Dr. Daniel Halperin for his substantial contribution to the writing and editing of this manuscript, Dr. Ismael Huertas for provided programming support, and to Drs. Rafael Carbunaru, Rosana Esteller, Tianhe Zhang and Lilly Chen for their intellectual input and editorial review.
Correction Statement
This article has been republished with minor changes. These changes do not impact the academic content of the article.
Additional information
Funding
References
- Melzack R, Wall PD. Pain mechanisms: a new theory. Science. 1965;150(3699):971–979.
- North RB, Ewend MG, Lawton MT, et al. Spinal cord stimulation for chronic, intractable pain: superiority of ‘multi-channel’ devices. Pain. 1991;44(2):119–130.
- Yearwood T, Foster A A prospective comparison of spinal cord stimulation (SCS) using dorsal column stimulation (DCS), intraspinal nerve root stimulation (INRS) and varying pulse width in the treatment of chronic low back pain. In: Digital abstract presented at CNS 56th annual meeting, Chicago, 2006.
- Kapural L, Yu C, Doust MW, et al. Novel 10-kHz high-frequency therapy (HF10 Therapy) is superior to traditional low-frequency spinal cord stimulation for the treatment of chronic back and leg pain: the SENZA-RCT randomized controlled trial. Anesthesiology. 2015;123(4):851–860.
- Pintea B, de Boni L, Kinfe TM. Subperceptional burst versus perceptional tonic spinal cord stimulation waveforms for drug-resistant orthostatic tremor: comparative data of 2 cases. Mov Disord Clin Pract. 2017;4(4):612–615.
- North JM, Hong KJ, Cho PY. Clinical outcomes of 1 kHz subperception spinal cord stimulation in implanted patients with failed paresthesia-based stimulation: results of a prospective randomized controlled trial. Neuromodulation. 2016;19(7):731–737.
- Shealy CN, Mortimer JT, Hagfors NR. Dorsal column electroanalgesia. J Neurosurg. 1970;32(5):560–564.
- Al-Kaisy A, Palmisani S, Smith T, et al. The use of 10-kilohertz spinal cord stimulation in a cohort of patients with chronic neuropathic limb pain refractory to medical management. Neuromodulation. 2015;18(1):18–23.
- Thomson SJ, Tavakkolizadeh M, Love-Jones S, et al. Effects of rate on analgesia in kilohertz frequency spinal cord stimulation: results of the PROCO randomized controlled trial. Neuromodulation. 2018;21(1):67–76.
- Lee KY, Bae C, Lee D, et al. Low-intensity, kilohertz frequency spinal cord stimulation differently affects excitatory and inhibitory neurons in the rodent superficial dorsal horn. Neuroscience. 2020;428:132–139.
- Chakravarthy K, Richter H, Christo PJ, et al. Spinal cord stimulation for treating chronic pain: reviewing preclinical and clinical data on paresthesia-free high-frequency therapy. Neuromodulation. 2018 Jan;21(1):10–18.
- Linderoth B, Foreman RD. Conventional and novel spinal stimulation algorithms: hypothetical mechanisms of action and comments on outcomes. Neuromodulation. 2017;20(6):525–533.
- Paz-Solis J, Thomson S, Jain R, et al. Exploration of high and low frequency options for sub-perception spinal cord stimulation using neural dosing parameter relationships: The HALO study. Neuromodulation. [In press].
- Feirabend HK, Choufoer H, Ploeger S, et al. Morphometry of human superficial dorsal and dorsolateral column fibres: significance to spinal cord stimulation. Brain. 2002;125(Pt 5):1137–1149.
- Veizi E, Hayek SM, North J, et al. Spinal cord stimulation (SCS) with anatomically guided (3D) neural targeting shows superior chronic axial low back pain relief compared to traditional SCS-LUMINA study. Pain Med. 2017;18(8):1534–1548.
- Bradley K. The technology: the anatomy of a spinal cord and nerve root stimulator: the lead and the power source. Pain Med. 2006;7(s1):S27–S34.
- Oakley JC, Prager JP. Spinal cord stimulation: mechanisms of action. Spine (Phila Pa 1976). 2002;27(22):2574–2583.
- Sdrulla AD, Guan Y, Raja SN. Spinal cord stimulation: clinical efficacy and potential mechanisms. Pain Pract. 2018;18(8):1048–1067.
- Jensen MP, Brownstone RM. Mechanisms of spinal cord stimulation for the treatment of pain: still in the dark after 50 years. Eur J Pain. 2019;23(4):652–659.
- Ji RR, Chamessian A, Zhang YQ. Pain regulation by non-neuronal cells and inflammation. Science. 2016;354(6312):572–577.
- Fan W, Sdrulla AD. Differential modulation of excitatory and inhibitory populations of superficial dorsal horn neurons in lumbar spinal cord by Aβ-fiber electrical stimulation. Pain. 2020;161(7):1650–1660.
- Lee KY, Ratté S, Prescott SA. Excitatory neurons are more disinhibited than inhibitory neurons by chloride dysregulation in the spinal dorsal horn. Elife. 2019;8:e49753.
- Zhang TC, Janik JJ, Grill WM. Modeling effects of spinal cord stimulation on wide-dynamic range dorsal horn neurons: influence of stimulation frequency and GABAergic inhibition. J Neurophysiol. 2014;112(3):552–567.
- Gilbert JE, Titus ND, Zhang TC, et al. “Computational modeling predicts dorsal columns are involved in fast-acting sub-perception spinal cord stimulation (SCS)” [Abstract P166.01]. Society for Neuroscience Global Connectome: A Virtual Event, Jan 11–13, 2021.
- Wolter T, Kiemen A, Porzelius C, et al. Effects of sub-perception threshold spinal cord stimulation in neuropathic pain: a randomized controlled double-blind crossover study. Eur J Pain. 2012;16(5):648–655.
- Kent AR, Weisshaar CL, Venkatesan L, et al. Burst & high-frequency spinal cord stimulation differentially effect spinal neuronal activity after radiculopathy. Ann Biomed Eng. 2020;48(1):112–120.
- De Carolis G, Paroli M, Tollapi L, et al. Paresthesia-independence: an assessment of technical factors related to 10 kHz paresthesia-free spinal cord stimulation. Pain Physician. 2017;20(4):331–341.
- Yearwood TL, Hershey B, Bradley K, et al. Pulse width programming in spinal cord stimulation: a clinical study. Pain Physician. 2010;13(4):321–335.
- Lee D, Hershey B, Bradley K, et al. Predicted effects of pulse width programming in spinal cord stimulation: a mathematical modeling study. Med Biol Eng Comput. 2011;49(7):765–774.
- Vesper J, Slotty P, Schu S, et al. Burst SCS microdosing is as efficacious as standard burst SCS in treating chronic back and leg pain: results from a randomized controlled trial. Neuromodulation. 2019;22(2):190–193.