ABSTRACT
Introduction
Patients with brief arrhythmias are a challenging group to treat effectively with catheter ablation. Current standard approaches for the localization and treatment of brief arrhythmias suffer from several limitations, including the lack of spatiotemporal stability and adequate resolution. Recently, novel methods became available that open new perspectives and can be implemented both on the atrial and ventricular level to approach the diagnosis and treatment of these arrhythmias.
Areas covered
In this paper, we demonstrate in each section a novel mapping modality that has a potential to approach arrhythmias considered unmappable in the past. After describing the method, we focused on the most important features of each system that makes mapping of short arrhythmias feasible. At the end of each section, we gave a short overview about necessary developments to improve the utility of these systems in the near future.
Expert opinion
Treating brief episodes of tachycardias remains a challenge and can cause significant frustration for electrophysiologists. Although the broadening of the indication is clearly visible, currently available sequential mapping techniques often fail to map short-lived arrhythmias. New beneficial technological features permit the mapping of these previously considered unmappable arrhythmias, and offer a new perspective in their management.
1. Introduction
Brief episodes of cardiac arrhythmias encompass a heterogeneous group of sustained or non-sustained arrhythmias, which include atrial tachycardias (ATs), ventricular tachycardias (VTs), premature atrial contractions (PACs) and premature ventricular contractions (PVCs) [Citation1,Citation2]. We defined brief episodes of ATs and VTs as arrhythmias unfeasible to map utilizing standard sequential mapping techniques due to their short duration. Patients can be highly symptomatic with brief episodes of ATs and VTs, and these arrhythmias can have a significant negative impact on cardiac function [Citation1,Citation2].
Although brief episodes of ATs and VTs are commonly seen in general population, the management of these arrhythmias is still suboptimal [Citation2]. Currently, the standard approach in AT and VT therapy is catheter ablation (CA), in which the localization by electroanatomical mapping of the chamber of origin plays a key role [Citation3,Citation4]. The main issue with short-lived arrhythmias is that patients are not always considered suitable candidates for CA, which is related to the limitations of sequential 3D mapping. Furthermore, in patients with sustained arrhythmias referred for CA, only short-lived arrhythmias can be induced which are difficult to map with sequential mapping techniques. Although extensive research has been carried out on sustained arrhythmia mapping and ablation, very few studies exist, which provide available data on brief arrhythmias. This indicates the need to provide a broad description about currently available mapping technologies and explore their feasibility in the treatment of short-lived arrhythmias.
Substantial improvements have been achieved over the last couple of years in the development of new mapping techniques of cardiac arrhythmias [Citation3]. These improvements aimed to advance the precision and speed of mapping [Citation5]. Additionally, some of the new possibilities may have the potential to offer a better understanding of arrhythmia mechanisms [Citation5]. A broad spectrum of new techniques is available in the global mapping of the cardiac chambers, such as dipole density, and single beat mapping techniques [Citation6]. Alternatively, noninvasive methods either simulation based such as View into Ventricular Onset (VIVO, Catheter Precision Inc., Mt. Olive, NJ), or body surface mapping like CardioInsight (Cleveland, OH, United States) and the noninvasive epi- and endocardial electrophysiology system (NEEES) became available [Citation7–9].
The aim of this methodological review is to provide a systematic overview about mapping techniques that can be implemented in the diagnosis and treatment of highly symptomatic brief episodes of ATs and VTs. In this review, we emphasize the description of mapping methods and their clinical use. We structured our paper as follows: after a short description of current approaches for brief arrhythmias, we describe four novel mapping technologies. Some of those can be used in both the atria and ventricles, others are chamber specific (). After describing the unique feature that makes it feasible for mapping, we provide a short overview about possible future developments that can make the specific technology widely adopted. To the best of our knowledge, this is the first paper aiming to provide a guide for more effective mapping of ATs and VTs with a short duration, considered unmappable and untreatable using previously employed mapping systems.
Table 1. Characteristics
Table 2. Strengths and limitations
2. Diagnosis and management
The diagnosis of short-lived arrhythmias is difficult; therefore, it can be established with certainty only by an electrophysiology (EP) study. The current mapping approaches are described in the next section. Data for an evidence-based choice of drugs for the pharmacological therapy of supraventricular arrhythmias are deficient. However, current guidelines recommend initiation of therapy with beta-blockers or calcium channel blockers. In the case of nonsustained or short-lived arrhythmias guidelines recommend no drug therapy but targeted CA [Citation10]. At the ventricular level, guidelines recommend beta-blocker therapy for PVCs and nonsustained VTs as pharmacological approach. Catheter ablation, however, is an important treatment option for patients with ventricular arrhythmias when antiarrhythmic medication is ineffective, not tolerated or not desired by the patient [Citation11].
3. Current approaches for brief arrhythmias
3.1. Pace mapping
Pace mapping is a technique generally used to identify the site of origin of mostly focal ventricular, and sometimes for atrial tachycardias. A recorded electrocardiogram (ECG) during the clinical tachycardia is conventionally used as reference. Pace mapping is performed by visually or automatically comparing the QRS or P wave morphology during overdrive pacing to the recorded reference ECG [Citation12,Citation13]. Anatomic reconstruction of the atrium or ventricle is initially performed using a three-dimensional electroanatomical mapping system during sinus rhythm. Bipolar pace mapping is then systematically performed at the areas of interest based on the algorithm of QRS or P wave morphology of the tachycardia on surface ECG. Later, the expected area and surrounding area are paced, according to specific structure. If one of these areas obtains a good match of the QRS complex or P wave, denser pace mapping is performed close to the good-paced match site to obtain best-paced match site. The CA target of the tachycardia is defined as best-paced site [Citation13].
Previous studies show that pace maps can be performed objectively and can be used for mapping brief episodes of atrial and ventricular tachycardias. In the atria, pace mapping can be useful for identifying focal AT origins by matching P wave morphology and intracardiac atrial activation sequence [Citation13]. In the ventricles, PVCs can be identified and successfully ablated using the pace mapping technique resulting in high acute and 1-year success rates [Citation12]. According to a study conducted by Bogun et al., pace mapping has a lower spatial resolution than activation mapping and fails to reproduce the morphology of arrhythmias arising in the right ventricular outflow tract in 20% of patients; therefore, it is not considered a reliable guide for ablation in these arrhythmias [Citation14]. Another limitation of pace mapping is that it cannot reproduce deep source arrhythmias due to cancellation effects and identifies the breakout or exit of the activation front. Summarizing, pace mapping is an elegant technique and it can be used as confirmation after ablating short-runs of atrial and ventricular tachycardias, however its endpoints are questionable when arrhythmias are non-inducible.
3.2. Anatomical or substrate-based ablation
Substrate-based ablation is defined as performing an ablation set directed toward low-voltage regions within the chamber of interest. This approach is useful when atrial and ventricular arrhythmias originate from scarred regions with abnormal myocardial conduction [Citation15]. Strategies include linear ablation, scar homogenization, scar dechanneling, core isolation, ablation of late potentials and local abnormal ventricular activity. All these strategies have their limitations; however, high-density mapping techniques may offer important features to improve efficacy and efficiency of substrate-based ablation. The value of this method can be applied in patients with NSVTs associated with structural heart disease, however NSVTs in non-structural heart disease patients remain unapproachable.
4. Novel cardiac mapping systems
Cardiac mapping has evolved in the last few years from a direct single point-by-point registration of electrical activity to furthermost complex multimodal real-time techniques [Citation5,Citation16]. The improvements of the technology have offered electrophysiologists a better understanding of cardiac arrhythmias and more precise ablation procedures resulting in higher success rate in arrhythmia management. Activation mapping is the cornerstone of mapping technologies in CA therapy [Citation5]. With improved understanding of cardiac arrhythmias, the concept of combining the electrical activation data obtained using mapping catheters with anatomical data guided by catheter position and contact, has been crucial in the successful outcome of CA procedures. Multiple mapping systems have been developed for this purpose. The most frequently used 3D mapping systems are the CARTO system (Biosense Webster, Diamond Bar, CA, USA), the EnSite Precision system (Abbott Laboratories, Chicago, IL, USA), and the Rhythmia HDx mapping system (Boston Scientific, Cambridge, Massachusetts) [Citation17].
Cardiac mapping systems record and analyze the electrical activity of the heart either invasively or noninvasively. The noninvasive surface ECG used by CardioInsight and VIVO systems can provide clues to the location of the arrhythmias, but one will not be able to determine the precise anatomic position of the origin of abnormal activity sites. Current invasive electroanatomical mapping systems use contact or noncontact mapping. Contact mapping relies on the invasive mapping catheter getting in contact with the cardiac tissue and sequentially acquiring location data points over many cardiac cycles. Noncontact mapping is based on a concept of simultaneous global acquisition of electrical activation data of an entire chamber without making physical contact with the endocardial surface. Global mapping of the cardiac chambers using noncontact mapping technique has the potential to map short runs of arrhythmias even from a single-beat registration, which is not feasible using sequential mapping techniques.
4.1. EnSite array
4.1.1. EnSite array technology
As far as we know, the EnSite multielectrode array (MEA) mapping system, introduced in 2009, was the first clinically available noncontact mapping technique in the history of 3D mapping. The system provides a detailed representation of electrical activation in a beat-to-beat registration for ATs and VTs; therefore, this ‘one-beat’ feature enables the MEA system to map non-sustained tachyarrhythmias. The EnSite multielectrode array catheter (St. Jude Medical, Saint Paul, Minnesota, USA) consists of a 7.5-mL ellipsoid balloon mounted on a 9 Fr catheter around which is woven a braid of 64 insulated 0.003-mm diameter wires. Each wire has a 0.025-mm break in insulation that serves as a noncontact unipolar electrode (). The system acquires more than 3000 noncontact unipolar electrograms from all points in the chamber simultaneously. The unipolar signals are recorded using a ring electrode located on the shaft of the array catheter as a reference. Recorded raw data is transferred to a silicon graphics workstation via digitized amplifier system. Endocardial unipolar electrograms can be calculated with the use of a mathematical inverse algorithm based on Laplace’s equation. The calculated electrograms are visualized on a surface mesh with approximately 3000 rectangular facets, which represents the geometry of the endocardial surface that is physically traced by a conventional catheter [Citation18,Citation19]. Isopotential maps with a range of colors are corresponding to voltage amplitudes.
Figure 1. Representation of the features utilized in the above described four mapping technologies. (A) Balloon catheter utilized in EnSite Array technology. (B) The AcQMap basket catheter. The distal end of this catheter is formed by six splines, each spline has eight ultrasound transducers interspersed between eight biopotential electrodes. (C) 252-electrode vest placed on the patient’s chest, utilized in the CardioInsight technology. (D) Patches placed on the patient’s chest, the 12-lead ECG and the camera, utilized in the VIVO technology.
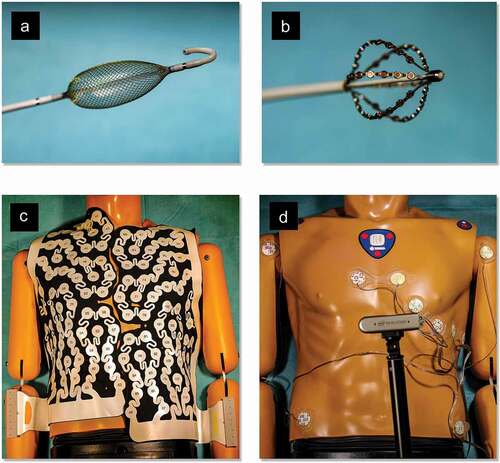
4.1.2. Clinical use for brief arrhythmias
With its global mapping and single-beat mapping features, the MEA mapping system provides the possibility of mapping brief episodes of atrial and ventricular tachycardias, too. In a study published in 2011 a group of 9 patients with brief episodes of ATs were mapped using MEA noncontact mapping system and treated with CA [Citation20]. Over a mean follow-up of 16 ± 11 months, eight out of nine patients remained free from symptoms, although three of these patients presented an ECG revealing single PACs at each follow-up visit. One of these patients had a redo procedure after 7 months. Assumingly, the MEA noncontact mapping system can be implemented in the treatment of short-lasting atrial arrhythmias. According to a study conducted in Japan in 2009, MEA mapping system has a significant accuracy in identifying VTs and PVCs. Miyamoto et al. described the safety and efficacy of MEA guided radiofrequency ablations for VTs and PVCs irrespective of their origin, mechanism, or sustainability [Citation21].
4.1.3. Limitations and necessary improvements
Whereas the MEA mapping technique is contributing to approximately 85% success rate and a 76% long-term success rate in inducible, sustained AT ablations, it presents several limitations [Citation13,Citation15]. It is important to mention the fact that MEA mapping system does not incorporate a 3D anatomical reconstruction, which is a major disadvantage regarding the temporospatial stability of the system. The computerized virtual image of the endocardium used by MEA can be a cause for possible errors in identifying the exact location and mechanism of the arrhythmia. Another limitation is that it is not consistently used for catheter ablation, and due to the difficulties of manipulating the ablation catheter with the balloon mapping catheter in the atria the universal use of this mapping technology is restricted [Citation22]. Patients with a dilated atrium or ventricle were excluded from mapping with the MEA system, due to the inaccuracy of the local activation, because its large distance from the center (>40 mm) [Citation13]. Improvements in 3D anatomical representation and a simplified user platform could increase the universal use of MEA mapping system.
4.2. The AcQMap high resolution imaging and mapping system
4.2.1. AcQMap technology
The AcQMap High-Resolution Imaging and Mapping System (Acutus Medical, Carlsbad, USA) provides maps of electrical activation across an ultrasound-acquired cardiac chamber surface [Citation6]. The system includes an invasive diagnostic recording catheter (AcQMap catheter) that is inserted into either the left or the right atrial chambers via the AcQGuide 12.8 F steerable sheath. The distal end of the catheter is deployed into a 25 mm diameter spheroid, formed by six splines. Each spline has eight ultrasound transducers interspersed between eight biopotential electrodes (inter-electrode distance 2.56 mm), resulting in a total of 48 sensors of each type (). The catheter and system are designed to acquire data without the need to contact the chamber surface. The endocardial surface is reconstructed based on point-sets generated with the use of ultrasound imaging techniques. To ensure the entire endocardial surface is sampled, the user needs to continuously rotate the catheter approximately 60 degrees in both directions. The system samples up to 115,000 surface points per minute. From this ultrasound point-set, the 3D surface is algorithmically reconstructed (). A minimal postprocessing is needed to remove outlier points and add definition to anatomical structures. The resulting chamber anatomy corresponds to the end-diastolic size and shape.
Figure 2. Reconstruction of the left atrium. (A) Start collecting points with the basket catheter. (B) End of collecting points. (C) Minimal editing – surface mesh. (D) Final reconstructed LA anatomy. (E) Example of a patient with focal atrial tachycardia originating in the left atrium. A modified Lao view of the LA representing the propagation history maps with breakout on the lateral wall. The color red is used to indicate the leading edge of the wave front with the trailing color bands showing earlier locations of the wave front. The activation wave front continues spreading to the anterior and posterior wall. CD1 represents the local charge density signal at the breakout point with a QS morphology. CS activation and leads III and V1 are also represented.
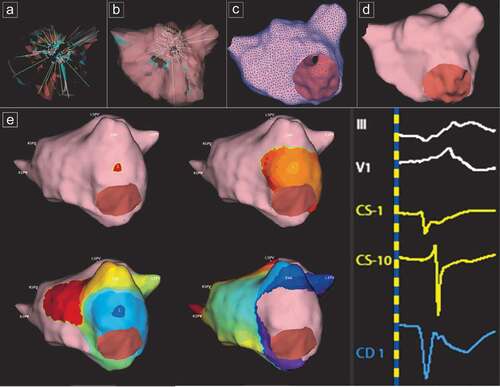
Cardiac voltage has served as the gold standard in electrical analysis of cardiac signals in the past. It appears as a spatially broad summation of local electrical charge sources generated by the action of cellular ion channels throughout the myocardial tissue. Charge density (CD), expressed in units of coulombs/cm, represents the magnitude of these sources, with a reduced contribution from far-field sources on the endocardial surface of the chamber and with a view of cardiac electrical activity that is at least 4 times sharper and narrower than voltage [Citation6,Citation22]. The major difference between voltage and dipole CD lies in the averaging effect of spatial summation and in the volume of space occupied by each. Spatial averaging causes the distribution of voltage to surround the charges and extend beyond the physical boundary of the charges. The AcQMap system uses an inverse solution to derive the dipole charge sources measured as dipole CD on the endocardium. To solve the inverse solution, the AcQMap system requires three key inputs: multiple simultaneous measurements of the potential field, which can be measured by the 48 biopotential electrodes on the AcQMap catheter, an accurate anatomic surface and a common coordinate system in which the AcQMap catheter is localized with 3D coordinates [Citation22]. The activation wave front can be displayed in its rawest form as either a charge- or voltage-based map of depolarization. A propagation-history map uses bands of color to show the location and velocity of the leading edge of the wave front over a set duration of time ().
The non-contact module of the AcQMap system consists of two different mapping modalities that both are unique in the way they record and process the unipolar voltage measured with the AcQMap catheter. Single position is mainly used for the global mapping of atrial fibrillation. Besides, mapping of AF, single position maps can also be used for single-beat analysis to map short non-sustained ATs or PACs. SuperMap is an algorithm designed to enable mapping of stable or transient rhythms with multiple morphologies. Due to the diagnostic error caused by signal attenuation over distance inherent to single position mapping, the SuperMap mapping modality was developed in order to acquire points in closer proximity to the endocardial wall. For the SuperMap algorithm, non-contact unipolar voltage electrograms are acquired by hovering the AcQMap catheter in the chamber of interest. A visual guided interface of surface illumination, when the non-contact electrodes are within 10 mm distance, will assist the operator in the data acquisition process. After roving around the AcQMap catheter, multiple non-contact catheter positions are time aligned based on unipolar CS activation. The CS channel with the least signal variability and largest peak-to-peak amplitude was automatically selected as the default primary reference. Subsequently, unipolar electrograms from CS were analyzed and binned based on morphology into beat groups. Selected beat groups were processed through the charge density inverse solution and presented as propagation-history map. Simultaneously, relative unipolar charge amplitude maps can be generated from the same data acquisition and displayed side-by-side.
4.2.2. Clinical use for brief arrhythmias
The AcQMap basket catheter is a key feature that allows the system to acquire global left atrial (LA) and right atrial (RA) maps. By rotating the basket catheter in the middle of the chamber, a global 3D anatomical map can be obtained. After obtaining the anatomical map, a noncontact single-beat single-position map of electrical activation can be acquired. These features allow the AcQMap mapping technique to identify any stable and unstable atrial arrhythmia, including brief episodes of ATs, which were previously unmappable using contact-based EAMS or other noncontact mapping modalities. This system has a major advantage compared to other noncontact mapping systems (e.g. EnSite Array) by offering a real-time anatomical map combined with high-resolution charge density maps of electrical activation.
4.2.3. Limitations and necessary improvements
A possible limitation of this technology is related to the size of the cardiac chamber and characteristics of the basket catheter. For accurate reconstructed atrial electrogram measurements the anatomical site of interest has to be within a radial distance of 40 mm from the center of the noncontact mapping catheter. On the other hand, the size and shape of the basket catheter does not allow mapping inside the pulmonary veins. Further, the AcQMap technology is limited to comparing post-processed voltage-based electrograms to corresponding contact electrograms at the same location on the atrial wall. However, voltage mapping is considered a gold standard in cardiac electrophysiology; dipole density is shown to be superior over standard voltage maps due to the four-times higher resolution [Citation6]. The lack of voltage map in the AcQMap technology is considered a limitation in the therapy of patients presenting with atrial flutter, post-PVI/box lesion. Another limitation of this technique is that it currently cannot be used for mapping of ventricular arrhythmias. A next step for this technology would be the mapping of VTs and PVCs. It is important to mention that this technology is cost intensive and may have significant economic impact.
5. Electrographic imaging (ECGI)
Electrocardiographic Imaging (ECGI) techniques are imaging technologies that noninvasively map cardiac electrical activity using the body surface ECG. They have been used to detect several ventricular and atrial arrhythmias, as potential targets for further invasive mapping before CA.
5.1. CardioInsight
5.1.1. CardioInsight technology
The CardioInsight (Cleveland, OH, United States) is a noninvasive ECGI mapping system that uses body surface ECG signals and a patient-specific anatomical model based on a CT scan input. The mapping procedure usually takes place on the same day of the CA procedure. A 252-electrode vest is first placed on the patient’s chest and a non-contrast chest CT scan is performed to obtain the patient-specific heart and torso geometry (). Atrial and/or ventricular geometry is reconstructed in order to obtain a 3D mesh. This model serves for the projection of unipolar signals represented by virtual nodes of the epicardial surface. ECGs are acquired during the patient’s sinus rhythm and arrhythmias pre-procedurally or during the EP study. For non-fibrillatory rhythms, mapping can be accomplished in a single beat, which is beneficial in non-sustained or short-lasting arrhythmias. Activation maps are computed using intrinsic deflection-based methods from unipolar electrograms. The CardioInsight system uses reconstructed signals to calculate the phase of each signal. Phase mapping permits identification of different activation patterns. In focal breakthrough, where activation starts from a discrete point and has a centrifugal spread, and in reentry, where a wave front rotates on itself within a localized area [Citation23].
5.1.2. Clinical use for brief arrhythmias
With the global mapping and the single-beat mapping features, CardioInsight technology can be used in mapping of both atrial and ventricular arrhythmias. With the 252-electrode vest, the CardioInsight noninvasively collects signals of electrical activation from the surface of the body and successfully locates the sources of ATs and VTs. Atrial signals are of lower amplitude compared with ventricular signals, therefore in atrial arrhythmias cumulative maps are used to locate the areas of focal breakthrough and reentrant drivers. In a study conducted by Cakulev et al. the CardioInsight technology could accurately identify the chamber of origin and mechanism of ATs in 10 of 10 cases. In the same study, they identified the PVC or VT origin in 9 of 11 cases using CardioInsight technology [Citation24]. In another study conducted by Erkapic and Greiss in 2014, a 95.2% accurate localization was documented in patients with PVCs. Failure to localize PVCs mainly occurred in sites near the septum [Citation25]. This difficulty to localize septal arrhythmias may come as no surprise as the calculated epicardial surface potentials do not allow for the visualization of the septum.
5.1.3. Limitations and necessary improvements
A major limitation of the CardioInsight is represented by the lack of a 3D-mapping navigation system, which causes an imperfect agreement between driver areas and ablation sites [Citation8]. Although image integration with the CARTO system is possible, it is not performed in all cases. Another possible limitation of the system is its inability to provide mapping of the septal area, therefore it is not feasible in localizing septal-source ATs and VTs [Citation26]. The CT imaging and body surface mapping is coupled with the CardioInsight technology; therefore, it has to be acquired on the same day as the CA procedure. For an accurate result, the mapping period must not exceed 2–3 hours prior to CA procedure.
According to Duchateau et al., agreement of ECGI activation and contact mapping is poor and heterogeneous. The between-map correlation is good for paced rhythms and wide QRS patterns, but it has shown to be inaccurate for narrow QRS rhythms. Lines of block and epicardial breakthrough sites imaged using ECGI are also inaccurate, especially in patients with multiple breakthroughs [Citation27]. Further work on inverse problem resolution and solution postprocessing is required to improve the accuracy of the technique. Obviously, the major pitfall of body surface mapping is that there is hesitation by its owner to further develop it. It is unclear what the future of this otherwise great technique is. As it is clear from the description of the technique, decoupling of the mapping moment from the imagining could be an important step toward its success. Moreover, as last it will be necessary to solve the issue of mapping either the interatrial septal tachycardias either the ventricular septal tachycardias. Those represent significant number of the arrhythmias and particularly difficult to ablate [Citation28–30]. Therefore, a preprocedural analysis would be a great asset to implement the right strategy as well as to improve outcome. Another problem with this approach is that it could be time-consuming due to the complex logistics of imaging.
5.2. The view into ventricular onset (VIVO) system
5.2.1. VIVO technology
VIVO is a noninvasive ECG imaging (ECGI) mapping system that uses a patient-specific myocardial model from contrast enhanced computed tomography scan (CT) or magnetic resonance imaging (MRI), a 3D photograph, and a standard 12-lead ECG to localize the origin of PVC and/or VT prior to CA procedure (). Shortly, the DICOM images (CT or MRI) of the thorax are imported in the VIVO system. Using a semi-automated morphology of a triangulated reference model, a patient-specific model of the ventricles and torso is created, which includes the septum as well as accurate wall thickness. In the next step, a three-dimensional photograph is taken of the thorax of the patient after placement of a 12-lead ECG (). This 3D image is then aligned with the patient-specific model of ventricles and torso. Activation sequences and the resulting ECGs from all cross points of the triangulated ventricular model are simulated. A recording of a PVC or VT on the 12-lead ECG is then imported into the system. The VIVO system than matches these recorded PVCs or with the simulated ECGs [Citation31].
Figure 3. VIVO workflow. In step one creation of patient-specific heart and torso model from MRI or CT (DICOM images). Step two is determining patient-specific electrode positions from 3D image acquired using the Intel RealSense camera, by merging the torso model and 3D image. In the next step, the 12-lead ECG is imported, and a single arrhythmia beat is analyzed. The white arrow is pointed toward the earliest activation site.
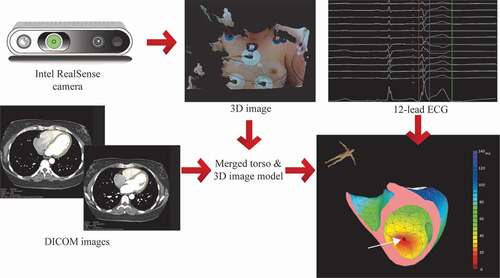
5.2.2. Clinical use for brief arrhythmias
The View into Ventricular Onset (VIVO) system offers a unique approach to localization of ventricular arrhythmias. Different from the CardioInsight technology, the de-coupling of the acquisition from the time of imaging provides extreme long theoretical acquisition time. This system uses stroke simulation to predict the localization of PVCs and VTs during EP studies. In the initial validation paper of the VIVO system 20 patients were mapped and underwent CA for the treatment of their PVCs or VTs. In 10 of 12 patients with PVCs the ablation procedure was successful. Seven of the eight patients with VTs had a successful CA procedure with non-inducibility of VT. According to this study, the VIVO platform accurately predicted the location of PVC and VT foci in 85% and 88% of patients [Citation31]. By analyzing ventricular activation vectors, rather than simulated surface potentials, the VIVO system may offer an advantage in identifying low-voltage pre-systolic signals that are frequently targeted during CA of VTs and PVCs [Citation31].
5.2.3. Limitations and necessary improvements
A major limitation of this mapping technique is that it has a complex workflow due to the required cardiac imaging. Secondarily, it cannot detect fusion of the propagation wavefronts; therefore, it can only map focal arrhythmias with homogenous propagation in the ventricles. Another limitation is lack of atrial mapping possibilities. Future developments are required in order to improve prediction accuracy for PVC localization. Modifications in the utilized software could be addressed, in order to further refine modeling. To optimize accuracy, improvements in myocardial segmentation and registration of surface electrodes to myocardial models should be considered.
6. Conclusions
This methodological review provides the first comprehensive evaluation of currently available novel mapping technologies in the treatment of brief episodes of atrial and ventricular tachycardias. Mostly, these arrhythmias were considered unmappable, therefore untreatable in clinical cardiology. Until recently, there has been little interest in the evaluation of standard therapeutic approaches for short runs of tachycardias and the improvement of their mapping possibilities. In the last few years, new mapping techniques became available with promising technological features that may offer new possibilities in cardiac mapping. These beneficial technological features permit the mapping of challenging and previously considered unmappable tachycardias such as PACs, brief episodes of ATs, PVCs and NSVTs. However, these techniques offer satisfying short-term and long-term outcomes, further developments are required in order to improve accuracy and efficacy.
7. Expert opinion
Treating brief episodes of tachycardias remains a challenge and can cause significant frustration for the practicing electrophysiologist. In the early years of electrophysiology, these arrhythmias were simply not attempted with interventions. In recent years, however, due to the increased number of patients and the improvement of mapping speed many more patients are scheduled for catheter ablation. Despite this, it remains a difficult issue due to the lack of dedicated mapping systems designed to treat this unique and slowly growing patient population. Although the broadening of the indication is clearly visible, currently available sequential mapping techniques often fail to map short-lived arrhythmias. Most importantly, most noninvasive mapping techniques suffer from the lack of a real-time anatomical map and adequate spatiotemporal resolution.
Currently, we implemented the above described techniques in our routine by taking advantage of their global mapping capabilities. All patients undergoing CA for possible short-lived arrhythmias are currently planned by using one of the above mentioned technologies. For unusual PVCs the major advantage is at the outpatient setting. For atrial tachycardias the AcQMap technology is exclusively used since there is no available alternative. Beyond this obvious indication we always consider this for ablation persistent atrial fibrillation since during the ablation it can convert into unstable ATs. Post-MAZE patients are also planned with AcQMap almost exclusively at our center due to the high likelihood of multiple short tachycardias during the procedure.
The mapping techniques used to guide catheter ablation procedures depend on the characteristics of the arrhythmias, with each technique having its own strengths and limitations. Choosing an appropriate mapping and ablation system for every patients may effectively ameliorate clinical results. However, in order to perform a personalized ablation, the chosen mapping system must incorporate all needed features for the mapping of the particular arrhythmia. Currently, the majority of patients with short-lived arrhythmias are initially planned for EP study using conventional mapping systems, but due to the non-inducible characteristics of their arrhythmias they are often rescheduled for a second procedure using a more adequate mapping system. Ideally, a complete mapping system should embody all available techniques, with the possibility of rapid strategy changing, in order to effectively treat patients with various arrhythmias. With such kind of system, we could reduce procedure times and significantly lower procedural costs. Furthermore, invasive electroanatomical mapping systems are limited by long mapping times (in contact mapping) or chamber specificity (in non-contact mapping). Up to now, there is no existing technology, which can be implemented universally and efficiently in both atrial and ventricular short-lived arrhythmias. Although the number of patients suffering from brief episodes is increasing, it is still very unlikely that any manufacturer will develop such a dedicated system incorporating all necessities to effectively treat these patients. Obviously, it would require enormous amount of investment for this niche patient population. Another option is – and the authors of this review think this is the best way to go – is to create a highly versatile mapping system that can be implemented on a very flexible way during the treatment of arrhythmia patients. This so-called universal mapping device should be able to incorporate all the useful features such as non-contact mapping, global chamber mapping, real-time anatomical reconstruction, pace mapping, single-beat mapping, and electrical beat simulation; and eliminate all the present limitations. With such a versatile technology, the interventional electrophysiologist will be able to map, identify, and efficiently ablate substrate-based or non-structural, sustained or non-sustained brief episodes of tachycardias in the atria and ventricles, too. We strongly believe the development of a complete system with all of these features available will provide the opportunity for a quick and effective intraprocedural strategy changing when necessary. With such an investment, not only ablation of the short-lived arrhythmias, but also actually many other aspects of the mapping process can be facilitated.
Article highlights
Brief atrial and ventricular arrhythmias are challenging to treat using standard sequential electrophysiology mapping techniques
New beneficent technological features permit the mapping of these previously considered unmappable arrhythmias
The MEA mapping system was the first non-contact technology able to map brief arrhythmias in the atria and ventricles too, however, due to the numerous limitations of this technique it is no longer used in clinical electrophysiology
The AcQMap high-resolution mapping system is a promising technique, which allows global chamber mapping in the atria, however it cannot be utilized in the mapping of brief ventricular arrhythmias
Due to the global mapping and single-beat mapping features, the noninvasive CardioInsight mapping system can be utilized in both atrial and ventricular arrhythmias, however this system is limited by the lack of an accurate 3D representation of chamber anatomy
The VIVO technique is a simulation-based mapping system, which offers a unique approach to localization of ventricular arrhythmias exclusively
Future developments are needed to create a universal device incorporating all the useful features and eliminating all the present limitations
Declaration of interest
The authors have no relevant affiliations or financial involvement with any organization or entity with a financial interest in or financial conflict with the subject matter or materials discussed in the manuscript. This includes employment, consultancies, honoraria, stock ownership or options, expert testimony, grants or patents received or pending, or royalties.
Reviewer disclosures
Peer reviewers on this manuscript have no relevant financial or other relationships to disclose.
Acknowledgments
The authors thank Emile Peters (Erasmus MC, University Medical Center Rotterdam) for providing professional images for the paper.
Additional information
Funding
References
- Calkins H. The 2019 ESC guidelines for the management of patients with supraventricular tachycardia. Eur Heart J. 2019;40(47):3812–3813.
- Kusumoto FM, Bailey KR, Chaouki AS, et al. Systematic review for the 2017 AHA/ACC/HRS guideline for management of patients with ventricular arrhythmias and the prevention of sudden cardiac death: a report of the american college of cardiology/American heart association task force on clinical practice guidelines and the heart rhythm society. Circulation. 2018;138(13):e392–e414.
- Friedman PA. Novel mapping techniques for cardiac electrophysiology. Heart. 2002;87(6):575–582.
- Cronin EM, Bogun FM, Maury P, et al. 2019 HRS/EHRA/APHRS/LAHRS expert consensus statement on catheter ablation of ventricular arrhythmias. Heart Rhythm. 2020;17(1):e2–e154.
- Shenasa M, Al-Ahmad A. Historical perspectives on cardiac mapping and ablation. Card Electrophysiol Clin. 2019;11(3):405–408.
- Grace A, Willems S, Meyer C, et al. High-resolution noncontact charge-density mapping of endocardial activation. JCI Insight. 2019; 4(6). DOI: https://doi.org/10.1172/jci.insight.126422.
- Dubois R, Shah AJ, Hocini M, et al. Non-invasive cardiac mapping in clinical practice: application to the ablation of cardiac arrhythmias. J Electrocardiol. 2015;48(6):966–974.
- Yamashita S, Shah AJ, Mahida S, et al. Body surface mapping to guide atrial fibrillation ablation. Arrhythm Electrophysiol Rev. 2015;4(3):172–176.
- Revishvili AS, Wer E, Lebedev DS, et al. Validation of the mapping accuracy of a novel non-invasive epicardial and endocardial electrophysiology system. Europace. 2015;17(8):1282–1288.
- Brugada J, Katritsis DG, Arbelo E, et al., 2019 ESC Guidelines for the management of patients with supraventricular tachycardiaThe Task Force for the management of patients with supraventricular tachycardia of the European Society of Cardiology (ESC). Eur Heart J. 41(5): 655–720. 2020.
- Al-Khatib SM, Stevenson WG, Ackerman MJ, et al. 2017 AHA/ACC/HRS guideline for management of patients with ventricular arrhythmias and the prevention of sudden cardiac death: executive summary: a report of the American college of cardiology/American heart association task force on clinical practice guidelines and the heart rhythm society. Circulation. 2018;138(13):e210–e271.
- Capulzini L, Vergara P, Mugnai G, et al. Acute and one year outcome of premature ventricular contraction ablation guided by contact force and automated pacemapping software. J Arrhythm. 2019;35(3):542–549.
- Hayashi K, Mathew S, Heeger CH, et al. Pace mapping for the identification of focal atrial tachycardia origin: a novel technique to map and ablate difficult-to-induce and nonsustained focal atrial tachycardia. Circ Arrhythm Electrophysiol. 2016;9(7). DOI:https://doi.org/10.1161/CIRCEP.116.003930.
- Bogun F, Taj M, Ting M, et al. Spatial resolution of pace mapping of idiopathic ventricular tachycardia/ectopy originating in the right ventricular outflow tract. Heart Rhythm. 2008;5(3):339–344.
- Ellis ER, Shvilkin A, Josephson ME. Nonreentrant ventricular arrhythmias in patients with structural heart disease unrelated to abnormal myocardial substrate. Heart Rhythm. 2014;11(6):946–952.
- Shah A, Hocini M, Haissaguerre M, et al. Non-invasive mapping of cardiac arrhythmias. Curr Cardiol Rep. 2015;17(8):60.
- Heist EK, Perna F, Chalhoub F, et al. Comparison of electroanatomical mapping systems: accuracy in left atrial mapping. Pacing Clin Electrophysiol. 2013;36(5):626–631.
- Narita S, Miyamoto K, Tsuchiya T, et al. Radiofrequency catheter ablation of atrial tachycardia under navigation using the EnSite array. Circ J. 2010;74(1):59–65.
- Schilling RJ, Peters NS, Davies DW. Feasibility of a noncontact catheter for endocardial mapping of human ventricular tachycardia. Circulation. 1999;99(19):2543–2552.
- Wieczorek M, Salili AR, Kaubisch S, et al. Catheter ablation of non-sustained focal right atrial tachycardia guided by virtual non-contact electrograms. Europace. 2011;13(6):876–882.
- Miyamoto K, Tsuchiya T, Narita S, et al. Radiofrequency catheter ablation of ventricular tachyarrhythmia under navigation using EnSite array. Circ J. 2010;74(7):1322–1331.
- Shi R, Norman M, Chen Z, et al. Individualized ablation strategy guided by live simultaneous global mapping to treat persistent atrial fibrillation. Future Cardiol. 2018;14(3):237–249.
- Cheniti G, Puyo S, Martin CA, et al. Noninvasive mapping and electrocardiographic imaging in atrial and ventricular arrhythmias (cardioinsight). Card Electrophysiol Clin. 2019;11(3):459–471.
- Cakulev I, Sahadevan J, Arruda M, et al. Confirmation of novel noninvasive high-density electrocardiographic mapping with electrophysiology study: implications for therapy. Circ Arrhythm Electrophysiol. 2013;6(1):68–75.
- Erkapic D, Greiss H, Pajitnev D, et al. Clinical impact of a novel three-dimensional electrocardiographic imaging for non-invasive mapping of ventricular arrhythmias-a prospective randomized trial. Europace. 2015;17(4):591–597.
- Shah AJ, Hocini M, Pascale P, et al. Body surface electrocardiographic mapping for non-invasive identification of arrhythmic sources. Arrhythm Electrophysiol Rev. 2013;2(1):16–22.
- Duchateau J, Sacher F, Pambrun T, et al. Performance and limitations of noninvasive cardiac activation mapping. Heart Rhythm. 2019;16(3):435–442.
- Graham AJ, Orini M, Zacur E, et al. Evaluation of ECG Imaging to map hemodynamically stable and unstable ventricular arrhythmias. Circ Arrhythm Electrophysiol. 2020;13(2):e007377.
- Kaneko Y, Kato NS. Characteristics and catheter ablation of focal atrial tachycardia originating from the interatrial septum. Heart Lung Circ. 2015;24(10):988–995.
- Guo XG, Liu X, Zhou GB, et al. Clinical, electrocardiographic, and electrophysiological characteristics of left upper septal fascicular ventricular tachycardia. Europace. 2018;20(4):673–681.
- Misra S, van Dam P, Chrispin J, et al. Initial validation of a novel ECGI system for localization of premature ventricular contractions and ventricular tachycardia in structurally normal and abnormal hearts. J Electrocardiol. 2018;51(5):801–808.