Abstract
Due to the unique characteristics of nanomaterials (NM) there has been an increase in their use in nanomedicines and innovative medical devices (MD). Although large numbers of NMs have now been developed, comprehensive safety investigations are still lacking. Current gaps in understanding the potential mechanisms of NM-induced toxicity can make it challenging to determine the safety testing necessary to support inclusion of NMs in MD applications. This article provides guidance for implementation of pre-clinical tailored safety assessment strategies with the aim to increase the translation of NMs from bench development to clinical use. Integrated Approaches to Testing and Assessment (IATAs) are a key tool in developing these strategies. IATAs follow an iterative approach to answer a defined question in a specific regulatory context to guide the gathering of relevant information for safety assessment, including existing experimental data, integrated with in silico model predictions where available and appropriate, and/or experimental procedures and protocols for generating new data to fill gaps. This allows NM developers to work toward current guidelines and regulations, while taking NM specific considerations into account. Here, an example IATA for NMs with potential for direct blood contact was developed for the assessment of haemocompatibility. This example IATA brings together the current guidelines for NM safety assessment within a framework that can be used to guide information and data gathering for the safety assessment of intravenously injected NMs. Additionally, the decision framework underpinning this IATA has the potential to be adapted to other testing needs and regulatory contexts.
Keywords:
Introduction
Nanomaterials (NMs) have at least one dimension in the nanoscale (1–100 nm) and can have strikingly altered properties compared to the bulk biomaterial, such as silver (Ag) or gold (Au) NMs which have been exploited in cancer treatments such as photothermal therapy and diagnostic imaging (Rawal and Patel Citation2019). As such, NMs are increasingly being used in medical devices (MD) (termed NM-MD) to improve treatment and diagnosis (Ratner and Bryant Citation2004; Osorio et al. Citation2019). Indeed, NM-MDs have already been used in sutures to promote healing, in dental implants, to replace damaged tissue in joint replacements, as theragnostic agents, and as biosensors (Edwards‐Jones Citation2009; Yang, Zhang, and Webster Citation2011; Lim et al. Citation2015; Thiagarajan et al. Citation2016; European Commission Citation2017; Houacine et al. Citation2018). To continue the exploitation of NMs, their safety and biocompatibility must be considered, as the potential adverse health effects of NMs are not yet fully understood (Accomasso, Cristallini, and Giachino Citation2018; Williams Citation2019).
The safety assessment of MDs is strictly regulated, with internationally accepted standards such as the International Standards Organization (ISO) standard series 10993, which is closely aligned with the European Commission regulation for MD, 2017/745 (European Commission Citation2017; International Organization for Standardization Citation2017a). The ISO 10993 series provides guidance on the testing that should be performed to evaluate MD safety and includes guidance (in Part 22) on the assessment of MDs that incorporate (or may generate) NMs. Safety testing of MDs for regulatory purposes has traditionally largely relied on in vivo models, which uses significant numbers of animals, and requires a considerable investment of resources and time (Johnston et al. Citation2018). As the numbers and complexity of NMs used in MD increases, it will be increasingly difficult to justify the large number of animals and the resources needed for testing to support regulatory acceptance. In addition, the relevance of in vivo animal data for predicting potential effects on human physiology is often challenged. ISO 10993 aims to support a potential reduction in animal testing by promoting non-animal testing throughout, allowing incorporation of available literature/data and the use of non-testing methods into the safety screening of the NMs. In addition, regulations, such as 2017/745/EC, promote alternative testing methods (European Commission Citation2017) and thus provide an opportunity to improve safety testing of NMs by making data gathering more ethical and hopefully financially viable. However, the rapid development of increasingly complex NMs means that industries find it challenging to develop appropriate safety testing strategies, especially difficult in non-animal testing, which tends to be associated with a higher degree of uncertainty than animal testing (Kroll et al. Citation2009; International Organization for Standardization Citation2017b).
A potential solution to help focus and streamline the safety assessment of NMs, to reduce the reliance on animal testing and to aid risk-benefit analysis is via the development and application of Integrated Approaches to Testing and Assessment (IATAs). IATAs, as proposed by the Organization for Economic Cooperation and Development (OECD) (Citation2017a), are decision trees that logically order questions to guide the identification of the most relevant information required to assess a specific hazard endpoint associated with exposure to potentially toxic substances.
Within the EU Horizon 2020 project BIORIMA (BIOmaterials RIsk MAnagement; Grant Agreement No: 760928), a risk management framework (RMF) has been developed that guides stakeholders (e.g. manufacturers, regulators, scientists, consultants) to identify data and tools that are useful to support risk assessment of NMs in an occupational or environmental setting (Giubilato et al. Citation2020). The BIORIMA RMF employs IATAs to guide the identification and generation of human and ecological hazard information to support risk assessment. Whilst the RMF focuses on the safety of NMs within occupational and environmental settings, the IATAs are not limited to these settings and can be applied to a wide range of exposure scenarios, including those in consumer and medical settings.
In the European Commission Horizon 2020 project GRACIOUS (Grant Agreement ID: 760840; Website: https://www.h2020gracious.eu/), several IATAs have been developed according to exposure context, for inhalation (Braakhuis et al. Citation2021; Murphy et al. Citation2021), ingestion (Di Cristo et al. Citation2021) and dermal routes (di Cristo et al., manuscript in preparation) of exposure to non-medical NMs. GRACIOUS has termed the questions of these decision trees as ‘decision nodes’ (Murphy et al. Citation2021). Each decision node is supported by a tiered testing strategy, structured to consider existing data and to guide testing using simple tier 1 methods (e.g. chemical reactions, assessing responses of monocultures of cell lines or modeling), tier 2 methods (e.g. more complex co-culture models) or tier 3 methods (in vivo). The GRACIOUS IATAs do not directly support risk assessment of individual NMs, but instead are formulated to support grouping and read-across of different NMs in order to streamline risk assessment (Stone et al. Citation2020). However, the GRACIOUS IATAs provide a useful tool to inform the generation of IATAs for other purposes such as risk assessment and to inform a risk-benefit analysis.
Introducing new approaches, such as IATAs, will allow NM safety assessments to be conducted while taking into consideration the need to reduce animal testing. Each IATA addresses a specific route of exposure thereby allowing a user to conduct targeted hazard assessment which they may wish to limit to the route of exposure of most concern in the first instance. However if multiple exposure scenarios apply additional IATA may need to be followed to fully assess the potential hazards posed by a NM. By prompting the user to perform safety testing in a tiered manner, the number of animals used for in vivo studies could be reduced, for example by identifying relevant exposure concentration ranges, by prioritizing relevant endpoints and by screening out highly toxic NMs at an early stage prior to animal testing.
The use of IATAs is not restricted to informing regulatory testing. Ideally, NM would be designed with safety aspects considered from the start of the development process, for example, by adopting safe(r)-by-design (SbD) strategies, with the physicochemical characteristics of the NM taken into account (Park et al. Citation2017). Therefore, the use of IATAs could allow industry to more clearly identify unsuitable candidates at an earlier stage in NM development, saving vital time and finances (Nieskens and Sjögren Citation2019).
This article aims to provide a methodology and guidance on the generation of IATAs to support the safety testing strategies for NMs. As a case study to illustrate the process, we will demonstrate the generation of an IATA intended to be used in the SbD process (e.g. by the developer) of NMs intended for intravenous (IV) injection into blood, that could be used to assess the haemocompatibility of the NM. The example NM investigated in this case study is a dispersion of Fe3O4 (magnetite) NMs used in magnetic hyperthermia for the treatment of solid tumors. The presented IATA could be applied to a wider range of IV administered NMs (e.g., different iron oxides NMs) to assess their effects on blood. The IV IATA could also be used to guide safety data acquisition for NMs that translocate into blood from other locations (e.g. lungs or gastrointestinal tract), but it does not cover the assessment of effects in other tissues (e.g. systemic toxicity). This article also aims to demonstrate the benefit of IATAs in promoting streamlined testing, through clearly structured research questions and application of tiered testing strategies to support decision making.
Guidance on the design and generation of an IATA for assessing the hazards of NMs
IATA structures
IATAs can be organized either as a sequential testing strategy such as a step wise linear decision tree, as outlined in (adapted from OECD. Citation2017a), or as an integrated testing strategy that assesses multiple sources of information simultaneously, as outlined in (adapted from OECD Citation2017a) (Horev-Azaria et al. Citation2013; OECD Citation2017a; Labouta et al. Citation2019). The choice between a linear versus an integrated testing strategy depends upon the hazards to be considered. Known or predicted toxicokinetics can also play a role in determining the IATA structure and the prioritization of decision nodes in a sequential IATA format or the decision to give equal weighting to decisions nodes if the integrated approach is followed. We will return to this discussion later.
Figure 1. IATA structures: sequential testing strategy and integrated testing strategy overview. A sequential testing strategy is shown in A, where the results obtained in one step of the IATA may generate adequate evidence for safety assessment, or the results can be used in conjunction with other information within the IATA. The integrated testing strategy shown in B demonstrates that results can be combined to generate sufficient evidence for safety assessment or can be used individually if sufficient alone. Adapted with approval from OECD (Citation2017a).
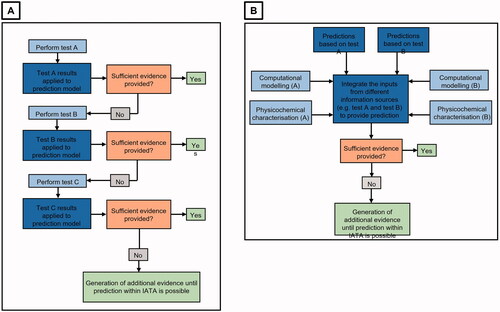
The generic IATA decision tree structure put forward here () follows a sequential strategy which uses decision nodes (orange square) containing specific questions that identify the information needed to make a decision regarding NM safety. To answer the questions presented, each decision node is associated with suggested testing methods and relevant guidelines/regulations (where available) related to these methods.
Figure 2. Basic building blocks of an IATA. The orange boxes contain decision nodes, whilst the red boxes contain outcomes based upon the decision made. The blue boxes contain information to provide the user with more context on the question or decision made. At the end of the IATA, the safety assessment may be complete, or the user may be directed to a subsequent IATA for further assessment.
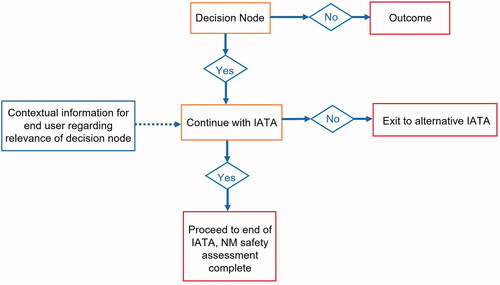
Design of an IATA
The key information required before a user can generate the appropriate decision nodes and outcomes presented within an IATA is highlighted in . This information includes details of the intended user of the IATA (e.g. industry, academic research, regulators), the purpose of the safety information gathering (e.g. for SbD, or to follow regulatory guidelines), the administration route (e.g. IV, inhalation), as well as their physicochemical characteristics (e.g. composition, size).
Figure 3. Types of information and data required to generate a tailored IATA for NM. A. When generating the IATA, the author must identify the end user of the IATA and the purpose of the IATA. In addition, information regarding the NM should be gathered, for example the route of NM administration and the primary system expected to be exposed, as well as the physicochemical characteristics of the NM. B. In this example, the information required to generate an IATA that will be used by a developer to design an intravenously injected NM containing 100 nm iron oxide NMs has been identified.
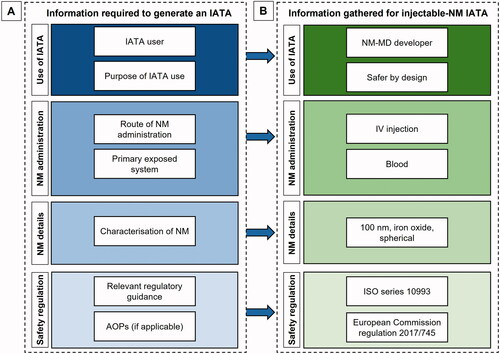
The IATA is designed so that the user can insert either estimated information (e.g. during the design phase), modeled information (e.g. during the early innovation phase) or real data (e.g. if testing information has already been generated). The goal is to use this information to focus the direction and level of detail needed in the subsequent IATA. This information equates to the ‘basic information’ that is also used in the GRACIOUS grouping framework, where it is used to generate a grouping hypothesis and subsequent IATA design (Murphy et al. Citation2021). If development of the NM proceeds, then any estimated or modeled information used in the basic information step can be replaced by measured values. Measured values will be essential for regulatory requirements.
When generating an IATA, the regulatory requirements and recommended methods available for the specific NM and administration (and therefore exposure) route must be identified (). This will feed into the IATA design and the information requested for specific decision nodes. The IATA design could also be influenced by any physicochemical characteristics of the particle under investigation, for example information regarding the endotoxin contamination of the NM should be gathered at this stage, as endotoxin contamination will affect the results of the safety assessment of the NM (OECD Citation2016). Furthermore, existing understanding of the mechanism of toxicity or an Adverse Outcome Pathway (AOP) that identifies molecular initiating events between a toxin and cell and the key events that result in an adverse outcome in the target tissue, can be used to inform the selection of pertinent IATA decision nodes questions (Willett Citation2019). The scheme outlined in , can be used to inform a literature and data search for existing information that allows design of a focused and relevant IATA and associated tiered testing strategies for each decision node. The information gathered should include, where available, human, in vivo, in vitro and in silico studies, as suggested in . The relevance, reliability, and adequacy of such studies can also be assessed using methods such as the Klimisch scoring system (Klimisch, Andreae, and Tillmann Citation1997; European Chemicals Agency Citation2017), or a data quality scoring system developed specifically for NMs (Fernández-Cruz et al. Citation2018). A similar approach could be used when identifying suitable data sources to support use of the IATAs for the risk assessment of NMs.
Figure 4. A diagrammatic representation of the approach used to generate an IATA. IATA generation is based on information gathering, assessment of the quality of data and identification of data gaps; this information is used to generate specific actions within the IATA that allow safety decisions to be made regarding NMs.
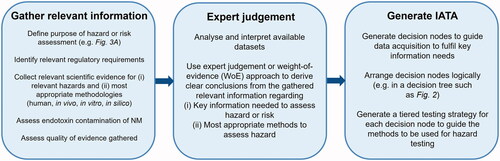
For some NMs, very little information may be available to directly inform IATA design. In this case, information from other materials used for similar applications, or the same materials used for different applications could be used. Where data are used for similar NMs, this can be informed via expert judgment or by grouping approaches as developed in GRACIOUS (Stone et al. Citation2020). The information required here is for informing the initial design of the IATA and so it does not need to be a full data set. When designing the IATA, it is useful to place more weight on the studies of highest scientific relevance, e.g. human or in vivo data, as these are more likely to generate relevant results. At this information gathering stage, expert judgment is required when identifying existing relevant data, and suitable weigh-of-evidence (WoE) approaches may be used.
Knowing the purpose of testing allows the user to tailor the IATA ( – IATA role), to deliver the level and type of detail required. For example, for the product developer considering SbD applications, new testing would focus on integrating existing data with in silico and in vitro approaches. In comparison, for regulatory purposes (for either a product developer or regulator), data generation from a wider array of sources, such as in vivo and human/clinical studies, may need to be considered. In the regulatory context, the use of existing information combined with in silico, simple in vitro testing and more advanced in vitro 3D models should decrease the amount of additional animal testing required, for example, by reducing the need for animal studies or by refining animal study design. In addition, from consideration of the potential uses of NM, the most relevant administration route(s) will be identified, allowing the IATA to be streamlined to that administration route (e.g. injection, inhalation, ingestion, or dermal) (Dai et al. Citation2018; Hamad et al. Citation2018).
The final IATA structure is therefore informed by combining the purpose of the NM risk assessment (considering risk assessment regulations), the type of application/administration, route of exposure, the physicochemical characteristics of the NM and any pertinent existing information relating to mechanisms of toxicity. This information allows specific tailored questions/actions to be generated and organized into the decision tree structure of the IATA that will allow decisions to be made regarding endpoints to prioritize for NM safety assessment. For example, the questions asked could relate to the physicochemical properties of the NM, such as whether the NM dissolution is fast or slow.
At this point, the IATA designer has a series of questions structured as decision nodes, which are designed so that ‘yes’ answers move down through the diagram, leading to an outcome where hazard is considered low, and ‘no’ answers move to the right. A ‘yes’ to the hazard endpoints does not mean there is risk, it means that an assessment of risk is required that considers exposure dose and hazard, and is tailored to the purpose of considering risk. It is likely that the IATA design is an iterative process, with the initial design edited to improve relevance and usability as different case studies are assessed. To answer the questions in each decision node, specific methods are required which are provided by the tiered testing strategy.
Development of tiered testing strategies to address individual decision nodes
Tiered testing strategies are needed to allow the required information to be compiled in order to address the questions of each decision node. The use of existing information is prioritized, with testing only conducted if data gaps exist, or if the existing data are insufficient in terms of quantity, quality or type (OECD Citation2017b) (see below).
If additional data are required, the tiered testing strategy for each decision node guides the acquisition of these data in a focused and progressive manner. Existing data also enable the identification of suitable controls and benchmark materials. Early tiers adopt alternative approaches such as in silico computer modeling and simple acellular or in vitro models, before leading to more complex 3D or ex vivo models, and finally to in vivo models, but only if the lower tiers do not provide sufficient certainty to support decision making or if required by regulations (OECD Citation2017a). Standardized methods (e.g. OECD test guidelines) are prioritized where available in order to increase data quality, to help reduce uncertainty and to support the mutual acceptance of data (Rasmussen et al. Citation2016).
A generic outline of the structure and use of a tiered testing strategy is provided in . Examples within the figure relate to the safety testing of an NM intended for intravenous (IV) injection, which will be discussed in greater detail in the following sections.
Figure 5. Tiered Testing Strategy (TTS) proposed for the risk-benefit analysis of NMs intended for administration via intravenous injection. In the first instance, all existing relevant peer-reviewed data should be considered. In tier 1, physicochemical characterization data and simple in vitro data should be used to guide computational evidence-based modeling regarding the NM safety. If additional data are required, progress is then made to tier 2 where complex in vitro based analysis takes place. Following tier 2, if additional data are required, in vivo analysis should be performed as per tier 3. At all stages, the existing peer-reviewed data and administration route should be considered.
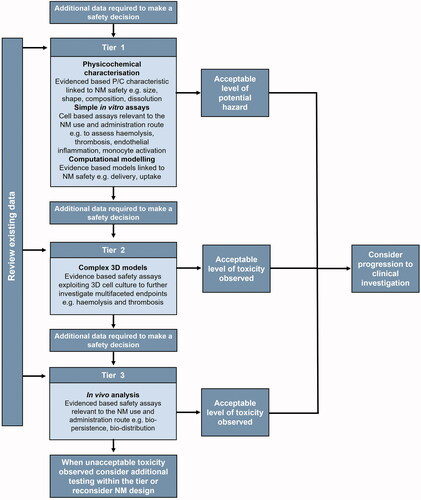
The tiered testing strategy does not have to follow a linear pattern. For example, if existing data for an in vivo model are sufficient to support decision making for that decision node, then no further testing is required. Some decision nodes may require threshold values that allow the user to make a decision (e.g. a percentage change in a blood clotting parameter which is considered pathophysiological relevant) (International Organization for Standardization Citation2017a). These thresholds need to be evidence based, where a specific magnitude of parameter (or biomarker) change is associated with an adverse outcome.
All outcomes from all decision nodes need to be compiled into a matrix of evidence in order to support the decision-making process. There are three possible outcomes of adopting the tiered testing strategy: 1) insufficient information is available and so more testing is required, 2) the level of toxicity is unacceptable thereby preventing progression to clinical testing, or 3) the toxicity observed is sufficiently acceptable to allow progression to clinical trial. No decision regarding progression to a clinical trial would be generated from a single decision node. Conversely, if one decision node generates an outcome that the level of toxicity is unacceptable to allow progression to a clinical trial, then the testing and IATA use could be stopped immediately, thereby saving time and money.
Use of quality data to support decision making
Data quantity and quality are essential to support the decision making described above. Data quality can be assessed by the Klimisch scoring system (Klimisch, Andreae, and Tillmann Citation1997; European Chemicals Agency Citation2017). The suitability and quality of generated data may require use of expert judgment, which can be assisted by WoE approaches when different types of data need to be combined to make an assessment. WoE can be performed via a simple structured scoring system, where the quality of the information is scored according to relevance, reliability, and strength such as those used by the United States Environmental Protection Agency (US EPA) or by multi-criteria decision analysis methodologies (U.S. Environmental Protection Agency (U.S. EPA) Citation1992; Hristozov et al. Citation2014; Suter, Cormier, and Barron Citation2017; Rocca et al. Citation2018).
Applying the guidance for designing an IATA to assess the safety of a specific NM case study
Based on the guidance above, an IATA was developed that is intended to be used for the safety assessment of an NM designed for IV injection to assess the effects of the NM on the blood only (haemocompatibility).
Starting with the information requirements outlined in , the case study includes the NM developer as the user, and their purpose of using the IATA; to incorporate safety into the design process. The selected NM for the presented case study is a dispersion of spherical and uncoated magnetite (Fe3O4) NMs with a particle size of 100 nm, used as hyperthermia agent in the treatment of solid tumors and administered via IV route. This is the bare minimum information needed to initiate the IATA development. No relevant AOP was identified to direct the hazard assessment.
According to and , an assessment of the identified regulatory guidelines is required. A number of ISO standards and European Medicines Agency (EMA) recommendations were therefore identified and incorporated into the IATA () and tiered testing strategies () below. Each are presented in a logical format in order to promote accessibility to a wide range of stakeholders.
Figure 6. IATA for the safety assessment of NM intended for intravenous injection. A streamlined IATA that focuses on safety endpoints associated with intravenous injection and subsequent blood contact of NM.
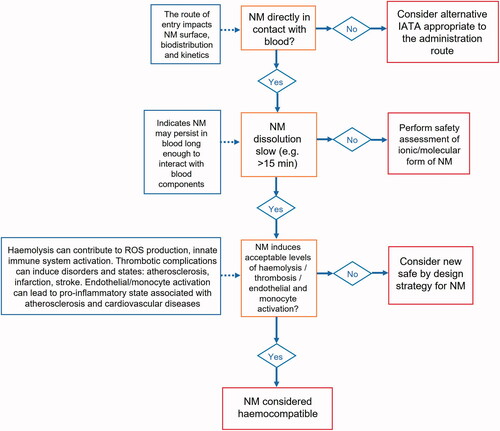
Figure 7. Proposed tiered testing strategy for intravenously injected NM safety assessment. This figure accompanies the IATA to guide the end user through the relevant guidelines, and where applicable, the appropriate tests that should be used to assess NM safety. The TTS provided guides the user through the assessment of haemocompatibility only.
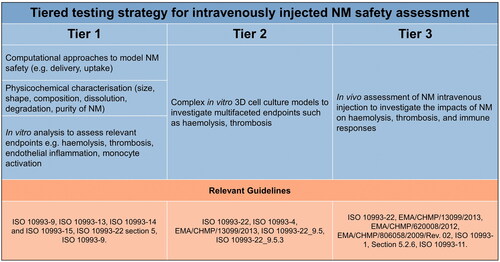
As a case study to assess the suitability of the IATA generation process, we followed the process outlined in , to use the IATA to gather relevant existing information on the haemocompatibility of Fe3O4 NMs, to highlight potential data gaps and thus guide NM safety assessment. To do this, a literature search was conducted using the search terms ‘Fe3O4 OR iron oxide OR magnetite’ AND ‘nanoparticle OR nanomaterial OR nanoform’ AND ‘IV OR intravenous OR blood’. The literature search was performed in June 2021 using the Heriot-Watt University library resources (e.g. Discovery, Web of Science). The papers obtained were organized into in vivo and in vitro studies, in order to allow appropriate weight to be given to the information provided. The quality of each paper and the major findings of each paper were summarized by means of a table which captured information on the particle physicochemical characteristics, the hazard model used, the dose range tested, the endpoints assessed, and the hazards identified (see supplementary information). Expert judgment was used to combine the information, along with the regulatory requirements and the route of administration to inform the IATA design. The information gathered from the published literature highlights that there are currently substantial data gaps regarding the haemocompatibility of Fe3O4 NMs. Furthermore, the existing data show that the safety of Fe3O4 NMs may be altered by the physiochemical properties of the Fe3O4 NM, such as the coating and particle size.
presents the resulting IATA, which adopted the sequential IATA format and follows the logical biokinetic path from the administration route to potential adverse outcome i.e. administrated by IV leading to particle-driven toxicity in blood cells resulting in adverse effects in the circulatory system. The IATA starts with a decision node, asking the user to confirm that the NM comes into contact with blood. If the answer is ‘no’ then the user is directed to consider other administration routes and targets. If the answer is ‘yes’ an information box is provided that outlines the need to consider changes to the surface of the NM through interaction with the blood, as well as biodistribution and kinetics of the NM.
The next decision node addresses dissolution of the NM. This is driven by the observation that metal oxide NMs can dissolve in biological media, and that the release of ions can contribute to their toxicity (Tombácz et al. Citation2015; Wang et al. Citation2016; Vakili-Ghartavol et al. Citation2020). If the Fe3O4 NM demonstrates substantial dissolution (e.g. in biological medium) then the user is prompted to conduct safety assessment based upon the soluble ions. When an NM dissolves quickly (e.g. in blood) there may be potential for the constituent ions to reprecipitate into the particle form, however, the user would still need to assess the safety of both the particle and ionic form of the NM and thus the safety assessment would proceed in the same way.
If dissolution is regarded as sufficiently low to prevent Fe2+/Fe3+ contribution to hazard, then an information box prompts the user to consider an NM that can persist for long enough in the blood to interact with blood components. Identification of the thresholds for dissolution of Fe3O4 NM to support decision making are beyond the scope of this case study and paper. If the end user continues with the IATA at this stage, they are then asked to consider three main potential biological responses to NM-blood interactions to determine haemocompatibility: hemolysis (disruption of red blood cells), thrombosis (blood clotting), and endothelial or monocyte activation (inflammation of blood vessels) (Ghasemi-Mobarakeh et al. Citation2019). These particular endpoints are based upon the recommendations within ISO 10993-4, which provides details on the types of testing that should be performed to identify MD interactions with blood (ISO 2017). As each of these biological responses are distinct potential hazards, the three endpoints are equally weighted as a ‘yes’ to any of them will result in the need to consider risk. Within this ISO standard, the relevant experimental procedures are also recommended. If significant hemolysis, thrombosis, endothelial or monocyte activation occur, then the user is prompted to reconsider the design of the NM in order to reduce its hazard. If the hazards are sufficiently low according to expert judgment, then the NM is considered haemocompatible. However, the ISO 10993-4 guidelines do not suggest suitable thresholds of safety. For example, the guideline states that acceptable and unacceptable amounts of hemolysis cannot be defined for all MDs and applications. Therefore, as part of NM safety assessment, it is always important to perform a benefit-risk analysis (BRA) related to patient exposure, as an adverse outcome may not prevent the progression of the NM, since the benefit to the patient may outweigh the risks of the treatment (Giubilato et al. 2020). Therefore, the IATA relies on the user to define the acceptable level of potential risk. For example, before the user can determine the haemocompatibility of the NM, they must determine acceptable levels of hemolysis, thrombosis, and endothelial/monocyte activation based upon a BRA.
In addition to the presented IATA, a tiered testing strategy table was also generated ().
When assessing the haemocompatibility of NMs using a tiered testing approach, data generated in earlier tiers may not be sufficient to make a safety decision, due to the complexities involved in the formation of blood clots and activation of endothelial cells/monocytes. Thus, for SbD purposes, where a full safety assessment is not necessary, it will be crucial to understand the limitations of the lower tier testing to interpret the results appropriately and to make an informed safety decision (e.g. to proceed with product development).
The standard operating procedures selected to be included within the IV IATA tiered testing strategy are validated and have been derived from the regulatory guidelines such as the ISO 10993 series (on the biological evaluation of medical devices). It is important that standardized tests are used within toxicity studies to reduce uncertainty and to promote the mutual acceptance of data. However, the IATA could be adapted to include additional endpoints and testing methods, e.g. depending on the needs of the user, as they become more widely utilized and validated. A key role of the IATA in the safety assessment of NMs is to highlight the testing guidelines required for regulatory purposes so that they are easily accessible within a framework that can be accessed and used, for example, during NM research and development.
Discussion
The aim of this article was to develop guidance for the generation of IATAs for NM safety assessment, and then to demonstrate the process by building an IATA using as a case study to determine their effects on blood. We exemplified the generation of an IATA for NM safety assessment with a case study based on the IV administration of a dispersion of magnetite (Fe3O4) used for magnetic hyperthermia in the treatment of solid tumors. The resulting IATA takes into account the relevant regulations, existing information/data related to the toxicity of the NM, the route of NM administration, the physicochemical characteristics of the NM, the intended applications for the NM and the purpose of the NM safety assessment. This IATA and tiered testing strategy identified the data needs for the hazard assessment of Fe3O4, and provided a strategy to generate new datasets to support the assessment of Fe3O4 haemocompatibility.
This guidance document highlights the types of information required and the approaches necessary to generate IATAs for NM safety assessment in a user-friendly structure. The structure of the IATA and prioritization of decision nodes are critical to making the hazard assessment of NMs more efficient. For example in the IATA presented here we prioritize examining the dissolution of the NM as an indicator of biopersistence before progressing to hazard assessment. If a user started with hazard assessment rather than dissolution testing for a NM that rapidly dissolved in biological fluids they may have invested significant time and effort in the assessing the toxic response of cells to exposure to a NM that they are unlikely to encounter in a real-life scenario. Conversely the real hazard posed by the ions or degradation products of the non-biopersistent NM may be missed. Therefore applying the IATA presented here may save the user time and resources while also ensuring the hazard assessment is conducted on the exposure-relevant form (NM particle, constituent ions, mixture) of the substance under investigation.
Knowledge of the regulatory guidelines combined with existing peer-reviewed published data that exist for NM are needed to both formulate the IATA questions, and to guide testing should the generation of data be required. Alignment with the current regulatory guidance will ensure the IATA users make decisions that in the future will align the NM with clinical testing requirements. Following the IATA process will ensure users who may not be familiar with the current guidance or best practice approaches are including these requirements in the design of their hazard assessment studies, ensuring the required endpoints are sufficiently addressed while minimizing unnecessary testing.
The route of administration information ensures the IATA focuses on the most immediate tissues of exposure. Additions to the IATA, or linking to other IATAs will be required to address hazards in other target sites should the NM be shown to translocate from the blood. Biodistribution and kinetics studies will be essential to address this information gap. The GRACIOUS IATA were designed following a standardized format to allow interconnectivity between the IATAs i.e. a ‘no’ outcome at one decision node will lead a user to leave one IATA but may present the option to link directly to an alternative (Stone et al. Citation2020). For example, there are 4 interconnected IATA following inhalation exposure which differentiate NMs based on dissolution rates and how biopersistence impacts the fate and hazard outcomes of exposure to different NMs (Braakhuis et al. Citation2021). As further IATA are developed, tested and refined for the BIORIMA purposes explicit linkages between IATA which can account for variations in properties e.g. dissolution rate, can be taken into account and the user will be guided to follow the most relevant testing strategy for each different NM.
In this article, we have demonstrated the methodology needed to generate an IATA and have applied this approach to generate an example IATA intended to be used by developers of NMs and NM-MDs for intravenous injection. This example IATA takes into account the regulatory guidelines that govern the safety assessment of NMs, as well as considerations of the NM administration route (injection), and potential adverse effects (e.g. in blood). This IATA guides the user through considerations they must make, via a tiered testing approach, to determine the suitability of the NM for injection into blood. Of benefit is that IATAs have the flexibility for users to select the types and level of testing required, that meet their specific needs. In order to support the development of NMs for patient use, testing strategies need to be specific and focused. These testing strategies should not only contain the classical animal based testing approach, but also include integrated in vitro and in silico testing approaches at earlier stages of testing, allowing identification of promising NMs for further investment and development. The incorporation of animal alternative approaches is in line with new guidelines for pre-clinical screening of NM-MDs, ISO 10993:2 and 10993:11 (International Organization for Standardization Citation2006, Citation2009). By promoting early stage in vitro safety assessment via streamlined tiered testing strategies, IATAs could enable the timely safety assessment of NMs through pre-clinical testing to patient use, and potentially limit the attrition of NMs at the later stages of development. Thus, a tiered testing strategy has the potential to reduce time and financial investment, as well as improve ethical acceptance of testing during the development of NMs and NM-MDs.
The OECD has generated guidelines for IATA development, highlighting the use of AOPs to inform the risk assessment testing (OECD Citation2016). The AOP framework allows the integration of in vitro and in silico testing, and is based upon chemically-induced mechanisms of action, linking molecular initiating events (MIEs) (such as receptor activation), with biological responses (termed key events (KE)) (e.g. protein production), that directly result in adverse outcomes (AO) (e.g. organ dysfunction). The AOP framework itself is appropriate for particle induced effects, while specific particle related key events need to be incorporated (Gerloff et al. Citation2017). This is exemplified by ongoing work to develop AOPs related to the inhalation of fibrous NMs, such as carbon nanotubes (CNTs) (Labib et al. Citation2016; Vietti, Lison, and van den Brule Citation2016; Halappanavar et al. Citation2020). Due to the lack of extensive standardized data for many NMs, the use of AOPs to inform IATA design is limited at this time, however in future selection of IATA decision nodes for toxicity testing may be based in validated KEs and thereby identify the activation of a specific AOP.
The IATA development method suggested here can be useful throughout the lifecycle of the NM, allowing specific focused testing using the most current data available. The collection of data in a structured manner for IATA generation, makes the scientific foundations of these IATAs easier to understand, build on, and refine in the future as new data are generated. However, before this can be achieved, such a body of data is essential to improve the efficiency and relevance of a hazard assessment to support risk assessment and risk decision making. This would allow testing to move away from the more check list testing to focused testing, that will reduce the resources and animal numbers required. For efficient data analysis, generation of a data matrix in which to collect such data, is recommended. When new data are generated, the relevant elements of these gathered data should be considered and recorded in a consistent manner (e.g. via a predefined template), in order to allow systematic assessment of suitability, quality, reliability and limitations. The use of open access databases (e.g. based on eNanoMapper currently used for non-medical nanomaterials) will be key to the efficient acquisition and sharing of data (Jeliazkova et al. Citation2015).
The example IATA presented here can be used when assessing the intentional administration of an NM to a patient via the IV route to determine the effects of the NM on the blood. Comparable IATAs could also be generated for the safety assessment of patients when other administration routes are considered. However, IATAs can be developed considering not only the safety of patients but also effects on workers and environment potentially exposed to NMs. For example, if generating IATAs for occupational and environmental exposure scenarios, it will be important to consider, depending on the level of testing desired, that these different regulations may alter the specific data requirements, affecting how the testing strategies are applied and how the data are interpreted. To assess the safety of NMs that are intentionally administered to patients, benefit risk analysis should be performed, to determine if the benefits of the treatment outweigh the potential risks of the NM, in accordance with ISO 14971 (British Standards Institution Citation2007; International Organization for Standardization Citation2019). It is likely that across these types of IATA similar physiochemical and toxicological methods and testing strategies can be adopted. In addition, consideration of the types of information sources (e.g. regulatory dossiers, journal articles, etc.) is needed, in order to assess the information relevancy, applicability and reliability, including an assessment of their strengths and limitations (Ankley et al. Citation2010; OECD Citation2017a, Citation2017b).
Finally, these IATAs can become part of a larger testing strategy covering different administration routes and different adverse outcomes, with each IATA considered to be a building block that interconnects with other accompanying IATAs. For example, important considerations related to a specific IATAs (e.g. physicochemical parameters in blood-contact IATA) can be connected with other IATAs (e.g. systemic IATA). The generation of this IATA ‘network’ will be useful in reducing duplication of work in the safety assessment of NMs, as well as allowing for testing in parallel using connected IATAs, which could reduce the costs and animal use associated with testing. These IATA aim to provide guidance and will be invaluable as part of online databases, as well as decision support systems for the safety assessment of NMs.
Conclusion
The method of IATA development put forward here is useful in helping NM-MD developers to focus and reduce the burden of testing with tiered testing allowing for the choice to consider key outcomes of interest or regulatory requirements. This will improve the safety and speed of NM entry to the market, as well as reducing the attrition of NMs at a later stage of safety testing. This is important because clinical trials require a much greater investment of resources than testing in earlier development stages.
IATAs generated using this methodology can be used to create a focused testing strategy for both current and future NMs. Additionally, this approach can also be used during pre-clinical screening to generate IATAs for administration routes relevant to clinical applications, such as dermal, oral, ingestion, and other medicinal products containing NMs. IATAs can be used as powerful tools to streamline safety testing and promote translation of NMs to the clinic.
IATAs promote the integrated use of in vitro and in silico approaches to generate a testing strategy that only answers the questions at the level needed to address safety concerns, providing flexibility for the user while saving resources and reducing animal numbers. IATAs can be part of a larger network of interconnecting IATAs, e.g. the intravenous injection IATA could lead on to a systemic exposure IATA, giving a more holistic approach to safety testing. Additionally, a range of different IATAs could be ‘plugged’ into a risk management framework.
IATAs can be applied across different regulatory landscapes, such as chemical and medical, allowing for a potential reduction in duplicate testing. This could also have the potential to drive the development of standardized methods across disciplines, to improve modeling with increased feedback of data into the IATA, and increase the use and reliability of in vitro testing in all regulatory fields for NM. Going forward, IATAs could be incorporated into a centralized database, similar to the AOP wiki (https://aopwiki.org/), that allows for the exchange and connection of IATAs, and open exchange of raw data, to further reduce the burden of testing in the future. Additionally, an extensive database of in vitro results would increase the confidence of regulatory bodies in these methodologies for the screening of pre-clinical NMs, potentially reducing the reliance on animal testing.
Disclosure statement
No potential conflict of interest was reported by the author(s).
Additional information
Funding
References
- Accomasso, L., C. Cristallini, and C. Giachino. 2018. “Risk Assessment and Risk Minimization in Nanomedicine: A Need for Predictive, Alternative, and 3Rs Strategies.” Frontiers in Pharmacology 9: 228. doi:10.3389/fphar.2018.00228.
- Ankley, G. T., R. S. Bennett, R. J. Erickson, D. J. Hoff, M. W. Hornung, R. D. Johnson, D. R. Mount, et al. 2010. “Adverse Outcome Pathways: A Conceptual Framework to Support Ecotoxicology Research and Risk Assessment.” Environmental Toxicology and Chemistry 29 (3): 730–741. doi:10.1002/etc.34.
- Braakhuis, H. M., F. Murphy, L. Ma-Hock, S. Dekkers, J. Keller, A. G. Oomen, and V. Stone. 2021. “An Integrated Approach to Testing and Assessment to Support Grouping and Read-Across of Nanomaterials after Inhalation Exposure.” Applied in Vitro Toxicology 7 (3): 112–128. doi:10.1089/aivt.2021.0009.
- British Standards Institution. 2007. Medical Devices: Application of Risk Management to Medical Devices. London: BSI.
- Dai, Q., S. Wilhelm, D. Ding, A. M. Syed, S. Sindhwani, Y. Zhang, Y. Y. Chen, P. MacMillan, and W. C. W. Chan. 2018. “Quantifying the Ligand-Coated Nanoparticle Delivery to Cancer Cells in Solid Tumors.” ACS Nano 12 (8): 8423–8435. doi:10.1021/acsnano.8b03900.
- Di Cristo, L., A. G. Oomen, S. Dekkers, C. Moore, W. Rocchia, F. Murphy, H. J. Johnston, et al. 2021. “Grouping Hypotheses and an Integrated Approach to Testing and Assessment Nanomaterials following Oral Ingestion.” Nanomaterials 11 (10): 2623. doi:10.3390/nano11102623.
- Edwards‐Jones, V. 2009. “The Benefits of Silver in Hygiene, Personal Care and Healthcare.” Letters in Applied Microbiology 49 (2): 147–152.
- European Chemicals Agency. 2017. Guidance on Information Requirements and Chemical Safety Assessment. Appendix R7-1 for Nanomaterials Applicable to Chapter R7a Endpoint Specific Guidance. Helsinki (Finland): European Chemicals Agency.
- European Commission. 2017. “Regulation (EU) 2017/745 of the European Parliament and of the Council of 5 April 2017 on Medical Devices.” Official Journal of the European Union : 175.
- Fernández-Cruz, M. L., D. Hernández-Moreno, J. Catalán, R. K. Cross, H. Stockmann-Juvala, J. Cabellos, V. R. Lopes, et al. 2018. “Quality Evaluation of Human and Environmental Toxicity Studies Performed with Nanomaterials – the GUIDEnano Approach.” Environmental Science: Nano 5 (2): 381–397. doi:10.1039/C7EN00716G.
- Gerloff, K., B. Landesmann, A. Worth, S. Munn, T. Palosaari, and M. Whelan. 2017. “The Adverse Outcome Pathway Approach in Nanotoxicology.” Computational Toxicology 1: 3–11. doi:10.1016/j.comtox.2016.07.001.
- Ghasemi-Mobarakeh, L., D. Kolahreez, S. Ramakrishna, and D. Williams. 2019. “Key Terminology in Biomaterials and Biocompatibility.” Current Opinion in Biomedical Engineering 10: 45–50. doi:10.1016/j.cobme.2019.02.004.
- Giubilato, E., V. Cazzagon, M. J. B. Amorim, M. Blosi, J. Bouillard, H. Bouwmeester, A. L. Costa, et al. 2020. “Risk Management Framework for Nano-Biomaterials Used in Medical Devices and Advanced Therapy Medicinal Products.” Materials (Basel, Switzerland) 13 (20): 4532. doi:10.3390/ma13204532.
- Halappanavar, S., S. van den Brule, P. Nymark, L. Gaté, C. Seidel, S. Valentino, V. Zhernovkov, et al. 2020. “Adverse Outcome Pathways as a Tool for the Design of Testing Strategies to Support the Safety Assessment of Emerging Advanced Materials at the Nanoscale.” Particle and Fibre Toxicology 17 (1): 16. doi:10.1186/s12989-020-00344-4.
- Hamad, T. I., A. A. Fatalla, A. S. Waheed, Z. G. M. Azzawi, Y. Cao, and K. Song. 2018. “Biomechanical Evaluation of Nano-Zirconia Coatings on Ti-6Al-7Nb Implant Screws in Rabbit Tibias.” Current Medical Science 38 (3): 530–537. doi:10.1007/s11596-018-1911-4.
- Horev-Azaria, L., G. Baldi, D. Beno, D. Bonacchi, U. Golla-Schindler, J. C. Kirkpatrick, S. Kolle, et al. 2013. “Predictive Toxicology of Cobalt Ferrite Nanoparticles: Comparative In-Vitro Study of Different Cellular Models Using Methods of Knowledge Discovery from Data.” Particle and Fibre Toxicology 10 (1): 32. doi:10.1186/1743-8977-10-32.
- Houacine, C., S. S. Yousaf, I. Khan, R. K. Khurana, and K. K. Singh. 2018. “Potential of Natural Biomaterials in Nano-Scale Drug Delivery.” Current Pharmaceutical Design 24 (43): 5188–5206. doi:10.2174/1381612825666190118153057.
- Hristozov, D. R., A. Zabeo, C. Foran, P. Isigonis, A. Critto, A. Marcomini, and I. Linkov. 2014. “A Weight of Evidence Approach for Hazard Screening of Engineered Nanomaterials.” Nanotoxicology 8 (1): 72–87. doi:10.3109/17435390.2012.750695.
- International Organization for Standardization. 2006. BS EN ISO 10993-2:2006. Biological Evaluation of Medical Devices. Part 2: Animal Welfare Requirements. Geneva, Switzerland.
- International Organization for Standardization. 2009. BS EN ISO 10993-11:2009. Biological Evaluation of Medical Devices. Part 11: Tests for Systemic Toxicity. Geneva, Switzerland.
- International Organization for Standardization. 2017a. BS EN ISO 10993-4:2017. Biological Evaluation of Medical Devices. Part 4: Selection of Tests for Interactions With Blood. Geneva, Switzerland.
- International Organization for Standardization. 2017b. PD ISO/TR 10993-22:2017. Biological Evaluation of Medical Devices. Part 22: Guidance on Nanomaterials. Geneva, Switzerland.
- International Organization for Standardization. 2019. BS EN ISO 14971:2019. Medical Devices. Application of Risk Management to Medical Devices. Geneva, Switzerland.
- Jeliazkova, N., C. Chomenidis, P. Doganis, B. Fadeel, R. Grafström, B. Hardy, J. Hastings, et al. 2015. “The eNanoMapper Database for Nanomaterial Safety Information.” Beilstein Journal of Nanotechnology 6: 1609–1634. doi:10.3762/bjnano.6.165.
- Johnston, H. J., R. Verdon, S. Gillies, D. M. Brown, T. F. Fernandes, T. B. Henry, A. G. Rossi, et al. 2018. “Adoption of In Vitro Systems and Zebrafish Embryos as Alternative Models for Reducing Rodent Use in Assessments of Immunological and Oxidative Stress Responses to Nanomaterials.” Critical Reviews in Toxicology 48 (3): 252–271. doi:10.1080/10408444.2017.1404965.
- Klimisch, H. J., M. Andreae, and U. Tillmann. 1997. “A Systematic Approach for Evaluating the Quality of Experimental Toxicological and Ecotoxicological Data.” Regulatory Toxicology and Pharmacology : RTP 25 (1): 1–5. doi:10.1006/rtph.1996.1076.
- Kroll, A., M. H. Pillukat, D. Hahn, and J. Schnekenburger. 2009. “Current in Vitro Methods in Nanoparticle Risk Assessment: Limitations and Challenges.” European Journal of Pharmaceutics and Biopharmaceutics : Official Journal of Arbeitsgemeinschaft Fur Pharmazeutische Verfahrenstechnik e.V 72 (2): 370–377. doi:10.1016/j.ejpb.2008.08.009.
- Labib, S., A. Williams, C. L. Yauk, J. K. Nikota, H. Wallin, U. Vogel, and S. Halappanavar. 2016. “Nano-Risk Science: Application of Toxicogenomics in an Adverse Outcome Pathway Framework for Risk Assessment of Multi-Walled Carbon Nanotubes.” Particle and Fibre Toxicology 13 (1): 15. doi:10.1186/s12989-016-0125-9.
- Labouta, H. I., N. Asgarian, K. Rinker, and D. T. Cramb. 2019. “Meta-Analysis of Nanoparticle Cytotoxicity via Data-Mining the Literature.” ACS Nano 13 (2): 1583–1594. doi:10.1021/acsnano.8b07562.
- Lim, E.-K., T. Kim, S. Paik, S. Haam, Y.-M. Huh, and K. Lee. 2015. “Nanomaterials for Theranostics: Recent Advances and Future Challenges.” Chemical Reviews 115 (1): 327–394. doi:10.1021/cr300213b.
- Murphy, F., S. Dekkers, H. Braakhuis, L. Ma-Hock, H. Johnston, G. Janer, L. di Cristo, et al. 2021. “An Integrated Approach to Testing and Assessment of High Aspect Ratio Nanomaterials and Its Application for Grouping Based on a Common Mesothelioma Hazard.” NanoImpact 22: 100314. doi:10.1016/j.impact.2021.100314.
- Nieskens, T. T. G, and A.-K. Sjögren. 2019. “Emerging In Vitro Systems to Screen and Predict Drug-Induced Kidney Toxicity.” Seminars in Nephrology 39 (2): 215–226. doi:10.1016/j.semnephrol.2018.12.009.
- OECD. 2016. Guidance Document for the Use of Adverse Outcome Pathways in Developing Integrated Approaches to Testing and Assessment (IATA). Paris: OECD Publishing.
- OECD. 2017a. Guidance Document on the Reporting of Defined Approaches to Be Used within Integrated Approaches to Testing and Assessment. Paris: OECD Publishing.
- OECD. 2017b. Alternative Testing Strategies in Risk Assessment of Manufactured Nanomaterials: current State of Knowledge and Research Needs to Advance Their Use. Paris: OECD Publishing.
- Osorio, M., A. Cañas, J. Puerta, L. Díaz, T. Naranjo, I. Ortiz, and C. Castro. 2019. “Ex Vivo and In Vivo Biocompatibility Assessment (Blood and Tissue) of Three-Dimensional Bacterial Nanocellulose Biomaterials for Soft Tissue Implants.” Scientific Reports 9 (1): 10553. doi:10.1038/s41598-019-46918-x.
- Park, M. V. D. Z., E. A. J. Bleeker, W. Brand, F. R. Cassee, M. Van Elk, I. Gosens, W. H. De Jong, et al. 2017. “Considerations for Safe Innovation: The Case of Graphene.” ACS Nano 11 (10): 9574–9593. doi:10.1021/acsnano.7b04120.
- Rasmussen, K., M. González, P. Kearns, J. R. Sintes, F. Rossi, and P. Sayre. 2016. “Review of Achievements of the OECD Working Party on Manufactured Nanomaterials’ Testing and Assessment Programme. From Exploratory Testing to Test Guidelines.” Regulatory Toxicology and Pharmacology : RTP 74: 147–160. doi:10.1016/j.yrtph.2015.11.004.
- Ratner, B. D, and S. J. Bryant. 2004. “Biomaterials: Where We Have Been and Where We Are Going.” Annual Review of Biomedical Engineering 6 (1): 41–75. doi:10.1146/annurev.bioeng.6.040803.140027.
- Rawal, S, and M. M. Patel. 2019. “Threatening Cancer with Nanoparticle Aided Combination Oncotherapy.” Journal of Controlled Release : Official Journal of the Controlled Release Society 301: 76–109. doi:10.1016/j.jconrel.2019.03.015.
- Rocca, M., L. L. Morford, D. L. Blanset, W. G. Halpern, J. Cavagnaro, and C. J. Bowman. 2018. “Applying a Weight of Evidence Approach to the Evaluation of Developmental Toxicity of Biopharmaceuticals.” Regulatory Toxicology and Pharmacology : RTP 98: 69–79. doi:10.1016/j.yrtph.2018.07.006.
- Stone, V., S. Gottardo, E. A. J. Bleeker, H. Braakhuis, S. Dekkers, T. Fernandes, A. Haase, et al. 2020. “A Framework for Grouping and Read-across of Nanomaterials- Supporting Innovation and Risk Assessment.” Nano Today. 35: 100941. doi:10.1016/j.nantod.2020.100941.
- Suter, G., S. Cormier, and M. Barron. 2017. “A Weight of Evidence Framework for Environmental Assessments: Inferring Qualities.” Integrated Environmental Assessment and Management 13 (6): 1038–1044. doi:10.1002/ieam.1954.
- Thiagarajan, V., S. Madhurantakam, S. Sethuraman, J. B. Balaguru Rayappan, and U. Maheswari Krishnan. 2016. “Nano Interfaced Biosensor for Detection of Choline in Triple Negative Breast Cancer Cells.” Journal of Colloid and Interface Science 462: 334–340. doi:10.1016/j.jcis.2015.10.014.
- Tombácz, E., R. Turcu, V. Socoliuc, and L. Vékás. 2015. “Magnetic Iron Oxide Nanoparticles: Recent Trends in Design and Synthesis of Magnetoresponsive Nanosystems.” Biochemical and Biophysical Research Communications 468 (3): 442–453. doi:10.1016/j.bbrc.2015.08.030.
- U.S. Environmental Protection Agency (U.S. EPA). 1992. Guidelines for Exposure Assessment. Washington, DC: National Academy Press.
- Vakili-Ghartavol, R., A. A. Momtazi-Borojeni, Z. Vakili-Ghartavol, H. T. Aiyelabegan, M. R. Jaafari, S. M. Rezayat, and S. Arbabi Bidgoli. 2020. “Toxicity Assessment of Superparamagnetic Iron Oxide Nanoparticles in Different Tissues.” Artificial Cells, Nanomedicine, and Biotechnology 48 (1): 443–451. doi:10.1080/21691401.2019.1709855.
- Vietti, G., D. Lison, and S. van den Brule. 2016. “Mechanisms of Lung Fibrosis Induced by Carbon Nanotubes: Towards an Adverse Outcome Pathway (AOP).” Particle and Fibre Toxicology 13 (1): 11. doi:10.1186/s12989-016-0123-y.
- Wang, D., Z. Lin, T. Wang, Z. Yao, M. Qin, S. Zheng, and W. Lu. 2016. “Where Does the Toxicity of Metal Oxide Nanoparticles Come from: The Nanoparticles, the Ions, or a Combination of Both?” Journal of Hazardous Materials 308: 328–334. doi:10.1016/j.jhazmat.2016.01.066.
- Willett, C. 2019. “The Use of Adverse Outcome Pathways (AOPs) to Support Chemical Safety Decisions within the Context of Integrated Approaches to Testing and Assessment (IATA).” In Alternatives to Animal Testing, edited by H. Kojima, T. Seidle, and H. Spielmann, 83–90. Singapore: Springer.
- Williams, D. F. 2019. “Specifications for Innovative, Enabling Biomaterials Based on the Principles of Biocompatibility Mechanisms.” Frontiers in Bioengineering and Biotechnology 7: 255. doi:10.3389/fbioe.2019.00255.
- Yang, L., L. Zhang, and T. J. Webster. 2011. “Nanobiomaterials: State of the Art and Future Trends.” Advanced Engineering Materials 13 (6): B197–B217. doi:10.1002/adem.201080140.