ABSTRACT
There is an increasing demand for advanced materials with enhanced temperature capability in highly corrosive environments, for instance enable space vehicles to resist several launches and re-entries. The EU-funded project C3HARME aims at combining the best features of CMCs and UHTCs to design, develop, manufacture and qualify a new class of Ultra-High Temperature Ceramic Matrix Composite (UHTCMCs) with self-healing capabilities. Applications selected to implement the new materials are near-zero erosion nozzles and near-zero ablation thermal protection systems. This paper aims at giving an introduction to the challenges addressed by C3HARME project including (i) the integration between well-established and novel techniques for CMCs and UHTCs production, (ii) the need for very high temperature characterisation, (iii) the meaning of self-healing capability for UHTCMCs, (iv) the contribution of modelling to materials development and (V) the investigation of the microstructure/thermo-mechanical property correlations.
Introduction
Silicon-based composites, such as C/SiC or SiC/SiC, are largely used for aeronautical and space application thanks to the beneficial protection of a SiO2 surface film [Citation1]. However, although SiO2 is an excellent oxidation barrier at temperatures up to 1600°C and in oxygen-rich atmospheres, above this temperature it begins to soften dramatically and in low-oxygen atmospheres it develops substantial vapour pressures [Citation2]. In particular, for propulsion with solid propellants, extensive fractions of Si-based phases are not acceptable. Another limit with such SiC-containing materials in thermal protection systems (TPSs) is the occurrence of (unpredictable) spontaneous surface temperature jumps during operation at elevated temperatures which may lead to extensive damage [Citation3,Citation4]. On the other hand, C/C or graphite based materials currently employed for rocket nozzles and hot parts are plagued by severe erosion. If not controlled, erosion may jeopardise flight safety due to uncontrolled thrust imbalance when, for instance, two boosters are fired at the same time.
In recent years, UHTCs have been extensively investigated as innovative thermal protection systems [Citation5–10], as well as other applications where oxidation and/or erosion resistance at temperature up to 2000°C are required. These materials include ZrB2, HfB2, ZrC, HfC and TaC, which have melting temperatures ranging from 3200 to 4000°C. For heat fluxes >10 MWm-2 and projected service temperature exceeding 2000°C, the thermal and chemical stability of UHTC compounds is of great importance and for this reason they are being considered as candidates for use in extreme environments, e.g. hypersonic flight and propulsion. More recently, the development of suitable techniques to densify and machine UHTCs at reasonable cost triggered a considerable amount of testing. UHTC nozzle inserts were tested in small scale rocket engine motors [Citation11] confirming that they lacked the capability to withstand rapid thermal gradients without suffering premature failure due to poor thermal shock resistance. However, these tests also confirmed the outstanding erosion resistance displayed by this class of ceramics. TPS systems such as nose cones and leading edges entirely based on bulk, unreinforced UHTC ceramics were successfully tested in arc jet experiments [Citation4].
ZrB2-SiC is the compositional system which attracted the largest attention of the scientific community [Citation4–7]. The post-test analyses confirmed the potential of ZrB2-SiC composites to endure re-entry conditions with temperatures approaching 2000°C, thanks to the formation of a solid, external multiphase oxide scale. However, prototypes with more realistic sizes failed during tests in ground simulated re-entry conditions due to lack of thermal shock resistance and insufficient damage tolerance [Citation5,Citation12]. In view of these recognised limits, the scientific community has redirected its effort to design and produce new reinforced UHTC systems by incorporating high aspect ratio secondary phases, mainly SiC whiskers, C or SiC fibres [Citation13,Citation14]. Innovative hot pressed/spark plasma sintered UHTCMCs using randomised C or SiC fibres embedded into ZrB2, HfB2 or TaC matrices were developed [Citation14–20]. The advantage of this process exploits conventional powder metallurgical methods, such as mechanical mixing, to introduce fibres or other reinforcing elements. The addition of 10–20 vol.-% SiC fibres increased the fracture toughness of ZrB2 up to 40% with respect to the unreinforced bulk, though the as-fabricated materials still exhibited brittle failure, due to the formation of strong fibre/matrix interfaces [Citation18].
A further step towards the creation of damage tolerant materials implies the use of continuous SiC or C fibres (Cf) infiltrated with UHTCs through the CMC technology [Citation21]. Various researchers produced carbon fibre-reinforced ZrC–SiC and ZrC–ZrB2–SiC composites through slurry infiltration, polymer infiltration and pyrolysis in a 3D-carbon fibre preform which showed non-brittle fracture behaviour, or 2D C/ZrB2–SiC and 2D C/SiC–ZrB2–TaC composites via slurry infiltration and chemical vapour infiltration (CVI) [Citation22,Citation23]. UHTCMC composites were fabricated [Citation2,Citation24] impregnating 2.5D Cf preforms with various UHTC powders (HfB2, ZrB2–SiC, HfC, etc.) by vacuum impregnation and CVI. Their high temperature performance was tested using an oxyacetylene torch test facility with the peak front face temperature being up to 2950°C. The HfB2-based composites performed significantly better than other compositions. Fibre-reinforced composites with a ZrB2 matrix were fabricated by reactive melt infiltration with a Zr-based intermetallic compound [Citation25]. Different precursors were evaluated in order to manufacture fibre reinforced preforms which allow for a melt infiltration process. Sample preforms contained boron powder, forming ZrB2 with Zr2Cu during melt infiltration. Depending on the precursors used, the composition of the infiltrated samples varied in the amount of ZrC and ZrB2 [Citation25]. Finally, both SiCf and Cf /ZrB2 UHTCMCs, coupling conventional impregnation techniques with sintering by hot pressing were recently fabricated [Citation26–31].
The rationale of C3HARME
The concept underpinning C3HARME is that, to overcome present technological limits, novel materials must be conceived, combining the best features of CMCs with those of UHTCs, as depicted in the sketch of . The integration of these two classes of materials should mitigate the limits from each, leading to multiple benefits. In this respect we aim at achieving improved erosion/ablation resistance compared to pure CMCs, improved damage tolerance and thermal shock compared to UHTCs and intermediate specific weight.
Figure 1. Sketch of UHTCMCs concept: wisely combining qualities of CMCs and UHTCs we would like to get improved erosion/ablation resistance compared to pure CMCs, improved damage tolerance and thermal shock compared to UHTCs and intermediate specific weight.
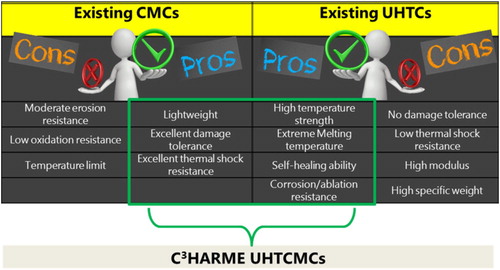
The newly developed materials can be fabricated both through the incorporation of fibres into UHTC matrices (ceramic approach) and through impregnation of fibre preforms (CMC approach) with UHTCs-based slurries and subsequent hot consolidation which can be accomplished through sintering or non-sintering techniques.
Applications selected to implement the new materials are near-zero erosion nozzles and near-zero ablation TPS tiles. Near-zero erosion materials for nozzles will allow better stability of the propulsion motor, avoiding problems arising from erosion in the throat. Near-zero ablation TPS materials will impart higher reliability and safety to spacecrafts (and their crew) due to improved manoeuvrability; there will be little or no change in aerodynamic performance due to surface recession while sharper profiles for leading edges will be maintained. Both applications, although different, share the need of ultra-refractoriness (imparted by the ceramic phase) and damage tolerance (imparted by fibres), but work in different aggressive chemical environments with reactive particle-loaded flows and in a broad range of surface pressures and heat flux rates. The corrosive environment of a propulsion chamber is characterised by intense enthalpy gas flows of CO/CO2/H2O vapour and the presence of condensed phases such as particles; while re-entry is characterised by high enthalpy reacting flows of fully dissociated gases (oxygen, nitrogen, etc.). This implies a need for different constituent materials, fibres and ceramic matrices, with suitable chemical/physical properties, compositions and architecture (fibre volume fraction, orientation).
A strongly recommended new functionality for materials to be used in extreme environments is self-healing capability. For bulk ceramics, self-healing is intended to be the partial or complete sealing of cracks through a thermal treatment, also called crack-healing. Recently, self-healing capability has been invoked for UHTCs ceramics. This happens when 10-20 vol.-% SiC particles, fibres, whiskers or MeSi2-based phases are added to UHTCs matrices (e.g. ZrB2 and HfB2). Self-protection is induced by surface-oxidation which results in formation of a protective borosilicate layer [Citation32]. In the bulk, oxidation products can easily penetrate into the defects and flaws, thus leading to cracks being blunted and healed. Doping with WC was reported to impart a self-healing capability thanks to sintering of the oxidation products (ZrO2, B–O glasses) developed during high temperature exposure [Citation33]. Similarly, it was reported that the addition of W into B2O3 increases the stability of the protective liquid/glassy layer resulting in higher oxidation resistance for (Zr,W)B2 compared to nominally pure ZrB2 [Citation34]. The self-healing capability of UHTCMCs to resist against extensive ablation/erosion in harsh environments met in propulsion and re-entry addressed by C3HARME project is meant to arise from the in situ formation of adherent, ultra-refractory solid oxide scales, essentially the oxidation products of the UHTC matrices. In this sense, incorporation of nanosised ceramic dopants such as WC can trigger chemicals reactions capable of better protecting the oxide scale during to exposure in severe conditions. The self-healing capability will result in great benefits, such as the increased reliability of the structural ceramic components and the cut of the maintenance costs.
Consortium and technical approach
C3HARME Consortium is led by CNR – ISTEC and is composed of three academic institutions (University of Birmingham, University of Naples ‘Federico II’, Trinity College Dublin), three research centres (National Research Council of Italy – Institute of Science and Technology for Ceramics, Fundación Tecnalia Research and Innovation, Deutsches Zentrum für Luft und Raumfahrt), three large companies (Avio S.p.A., Airbus Safran Launchers, Airbus Group) and three small/medium enterprises (IN Srl, Nanoker Research SL, High Performance Structures Gestão e Engenharia Lda); .
The Consortium merges a critical mass of scientific expertise and excellence in key areas of materials science, engineering, process technology, material modelling and processing, and industrial scale-up.
In order to achieve the ambitious goal of C3HARME, an iterative approach, including design, materials fabrication and environment centred testing is adopted. The research methodology involves a logical sequence for design, preparation and high temperature/thermo-mechanical property characterisation, as depicted in .
The C3HARME project activities start from the definition of technical requirements for the two applications and design of prototypes for characterisation at the lab scale and in relevant environment. On this basis, a collection of materials specifications and the preliminary selection of the most promising compositions were achieved. In C3HARME, different key technologies are considered: (a) hot pressing/spark plasma sintering (HP/SPS), (b) microwave chemical vapour infiltration (MCVI), (c) reactive metal infiltration (RMI), (d) polymer-infiltration-pyrolysis processing (PIP). Selection of the resulting best processing routes in the project is dictated by the need for fast processing/cost-effectiveness combined with quality of performance.
Throughout the project, multiscale modelling to orient the material development from the early stages, including characterisation and upscaling, is extensively used. Parameter-free density functional theory is used for understanding interfaces between materials, their performances under extreme conditions and their aging. Micro-models for chopped and continuous-fibre material with idealised microstructures where relevant properties are estimated from analytical or numerical (finite element) methods are under development. Further, numerical and analytical macro-models are used to predict the structural integrity of components during operation and fluid-dynamic calculations to understand the aerothermal behaviour and predict the gas/surface reactivity. All the research steps are accompanied by key testing/validation in order to provide solid support to our assumptions.
Scale up of components, both nozzles and tiles, are also pursued thanks to the collaboration with industrial partners. Finally, nozzles throats are the technological demonstrator to be produced and then tested under real operating conditions in small scale solid/hybrid propulsion motors, with temperatures up to 3000°C, pressure up to 100 bar, thrust up to 2500 N, burning time over 35 s. On the other hand, TPS tiles are the prototypes to be produced and thus tested in the arc heated facilities, at different enthalpy and pressure levels representing different flight trajectory points (temperatures up to 2500°C and testing time of several minutes).
Results
Promising results were obtained with the hot press (HP)/spark plasma sintering (SPS) technique for the fast hot consolidation of UHTCMCs. The preferred matrix is essentially based on ZrB2 enriched with secondary phases with different functionalities such as sintering aids, self-healing agents, reinforcing and anti-oxidation agents. Materials sintered and characterised include short fibre Cf reinforced composites produced using conventional powder processing [Citation35] followed by HP/SPS: near fully dense, crack-free materials were obtained. These composites are quasi isotropic, with homogenous distribution of fibres inside ultra-refractory ceramic matrices ((a)). By carefully mastering temperatures and dwell times during HP/SPS, the fibre/matrix interface bonding can be made stronger or weaker.
Figure 4. SEM images of (a) polished surface of a short fibre-reinforced composite, (b) polished section of an unidirectional ‘0/0°’ continuous fibre-reinforced composite. Inset: magnified image of fibres and matrix in (b).
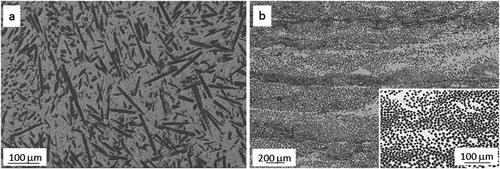
Continuous fibre-reinforced composites made using the slurry infiltration method followed by sintering were also produced [Citation29]. As for the textures, unidirectional ‘0/0°’ were first investigated ((b)) and fully characterised [Citation36]. Advanced textures characterised by architectures ‘0/90°’ and 2D are under study. Independently of the texture, due to excessive thermal expansion mismatch between fibre and matrix, some cracks initiated inside the densified matrix upon cooling. By carefully tailoring the cooling stage, the occurrence of cracks can be mitigated though not completely avoided. Delamination between overlapped layers may also occur: further optimisation of the matrix composition is continuing to limit this issue as much as possible.
The effect of SiC content in the ceramic matrix has been also investigated [Citation37], showing that SiC amount has effects mainly on densification and oxidation resistance, beside affecting the matrix/fibre interface adhesion. Attempts to sinter slurry-impregnated 2.5D and 3D fabrics via hot pressing are under development. Examples of small simple shaped prototypes are reported in .
Conclusions
Purpose of C3HARME project is to significantly improve performance of the existing materials for aerospace applications in terms of increased capability to withstand severe environments, achieving also efficiency, reliability, cost-effectiveness and scalability.
The C3HARME project pursues this goal by introducing innovative material solutions while adapting existing and well-established processing techniques. In this sense, C3HARME Consortium manages a well-balanced mixture of innovative and consolidated technology for new and very demanding applications, mitigating the level of risk intrinsic in top-quality research and innovative development.
Acknowledgement
This paper was originally presented at the Ultra-High Temperature Ceramics: Materials for Extreme Environments Applications IV Conference (Windsor, UK) and has subsequently been revised and extended before consideration by Advances in Applied Ceramics.
Disclosure statement
No potential conflict of interest was reported by the authors.
ORCID
Diletta Sciti http://orcid.org/0000-0002-3836-6118
Laura Silvestroni http://orcid.org/0000-0003-4595-0299
Frédéric Monteverde http://orcid.org/0000-0002-9766-2275
Antonio Vinci http://orcid.org/0000-0002-0144-0839
Luca Zoli http://orcid.org/0000-0002-6208-2035
Additional information
Funding
References
- Krenkel W. Ceramic matrix composites: fiber reinforced ceramics and their applications. 2008. doi:10.1002/9783527622412.
- Paul A, Binner J, Vaidhyanathan B. UHTC composites for hypersonic applications. Ultra-High Temperature Ceramics: Materials for Extreme Environment Applications. 2014:144–166. doi:10.1002/9781118700853.ch7.
- Panerai F, Helber B, Chazot O, et al. Surface temperature jump beyond active oxidation of carbon/silicon carbide composites in extreme aerothermal conditions. Carbon. 2014;71:102–119. doi:10.1016/j.carbon.2014.01.018.
- Monteverde F, Cecere A, Savino R. Thermo-chemical surface instabilities of SiC-ZrB2 ceramics in high enthalpy dissociated supersonic airflows. J Eur Ceram Soc. 2017;37(6):2325–2341. doi:1016/j.jeurceramsoc.2017.01.018 doi: 10.1016/j.jeurceramsoc.2017.01.018
- Mallik M, Roy S, Ray KK, et al. Effect of SiC content, additives and process parameters on densification and structure-property relations of pressureless sintered ZrB2-SiC composites. Ceram Int. 2013;39:2915–2932. doi:10.1016/j.ceramint.2012.09.066.
- Zou J, Zhang G-J, Vleugels J, et al. Strong ZrB2-SiC-WC Ceramics at 1600°C. J Am Ceram Soc. 2012;76:n/a), doi:10.1111/j.1551-2916.2011.05062.x.
- Hu C, Sakka Y, Grasso S, et al. Microstructure and properties of ZrB2-SiC composites prepared by spark plasma sintering using TaSi2 as sintering additive. J Eur Ceram Soc. 2010;30:2625–2631. doi:10.1016/j.jeurceramsoc.2010.05.013.
- Nino A, Hirabara T, Sugiyama S, et al. Preparation and characterization of tantalum carbide (TaC) ceramics. Int J Refract Metals Hard Mater. 2015;52:203–208. doi:10.1016/j.ijrmhm.2015.06.015.
- Silvestroni L, Guicciardi S, Sciti D, et al. Strength and toughness: the challenging case of TaC-based composites. Compos B: Eng. 2015;72:10–20. doi:10.1016/j.compositesb.2014.11.043.
- Silvestroni L, Guicciardi S, Sciti D, et al. TaB2-based ceramics: microstructure, mechanical properties and oxidation resistance. J Eur Ceram Soc. 2012;32:97–105. doi:10.1016/j.jeurceramsoc.2011.07.032.
- Savino R, Festa G, Cecere A, et al. Experimental set up for characterization of carbide-based materials in propulsion environment. J Eur Ceram Soc. 2015;35:1715–1723. doi:10.1016/j.jeurceramsoc.2014.12.032.
- Alfano D, Gardi R, Scatteia L, et al. UHTC-based hot structures: characterization, design, and on-ground/in-flight testing. Ultra-High Temperature Ceramics: Materials for Extreme Environment Applications. 2014: 416–436. doi:10.1002/9781118700853.ch16.
- Yang F, Zhang X, Han J, et al. Processing and mechanical properties of short carbon fibers toughened zirconium diboride-based ceramics. Mater Des. 2008;29:1817–1820. doi:10.1016/j.matdes.2008.03.011.
- Guicciardi S, Nygren M, Sciti D, et al. Microstructure and toughening mechanisms in spark plasma-sintered ZrB2 ceramics reinforced by SiC whiskers or SiC-chopped fibers. J Am Ceram Soc. 2010;93:2384–2391. doi:10.1111/j.1551-2916.2010.03730.x.
- Silvestroni L, Fabbriche DD, Sciti D. Tyranno SA3 fiber–ZrB2 composites. Part I: microstructure and densification. Mater Des (1980-2015). 2015;65:1253–1263. doi.org/10.1016/j.matdes.2014.08.068.
- Sciti D, Savino R, Silvestroni L. Aerothermal behaviour of a SiC fibre-reinforced ZrB2 sharp component in supersonic regime. J Eur Ceram Soc. 2012;32:1837–1845. doi:10.1016/j.jeurceramsoc.2012.01.019.
- Pienti L, Sciti D, Savino R, et al. Ablation tests on HfC- and TaC-based ceramics for aeropropulsive applications. Journal of the European Ceramic Society. 2015;35:1401–1411. doi:10.1016/j.jeurceramsoc.2014.11.018.
- Sciti D, Guicciardi S, Silvestroni L. Are short Hi-Nicalon SiC fibers a secondary or a toughening phase for ultra-high temperature ceramics? Materials and Design. 2014;55:821–829. doi:10.1016/j.matdes.2013.10.019.
- Sha JJ, Li J, Wang SH, et al. Improved microstructure and fracture properties of short carbon fiber-toughened ZrB2-based UHTC composites via colloidal process. Int J Refract Metals Hard Mater. 2016;60:68–74. doi:10.1016/j.ijrmhm.2016.07.010.
- Zoli L, Sciti D, Grasso S, et al. Rapid spark plasma sintering to produce dense UHTCs reinforced with undamaged carbon fibres. Mater Des. 2017;130:1–7. doi:10.1016/j.matdes.2017.05.029.
- Leslie CJ, Boakye EE, Keller KA, et al. Development and characterization of continuous SiC fiber-reinforced HfB2-based UHTC matrix composites using polymer impregnation and slurry infiltration techniques. Int J Appl Ceram Technol. 2015;12:235–244. doi:10.1111/ijac.12279.
- Li Q, Dong S, Wang Z, et al. Fabrication and properties of 3-D Cf/SiC-ZrC composites, using ZrC precursor and polycarbosilane. J Am Ceram Soc. 2012;95:1216–1219. doi:10.1111/j.1551-2916.2012.05116.x.
- Li Q, Dong S, Wang Z, et al. Fabrication and properties of 3-D Cf/ZrB2-ZrC-SiC composites via polymer infiltration and pyrolysis. Ceram Int. 2013;39:5937–5941. doi:10.1016/j.ceramint.2012.11.074.
- Paul A, Venugopal S, Binner JGP, et al. UHTC-carbon fibre composites: preparation, oxyacetylene torch testing and characterisation. J Eur Ceram Soc. 2013;33:423–432. doi:10.1016/j.jeurceramsoc.2012.08.018.
- Kütemeyer M, Schomer L, Helmreich T, et al. Fabrication of ultra high temperature ceramic matrix composites using a reactive melt infiltration process. J Eur Ceram Soc. 2016;36:3647–3655. doi:10.1016/j.jeurceramsoc.2016.04.039.
- Sciti D, Natali Murri A, Zoli L, et al. From random chopped to oriented continuous SiC fibers-ZrB2 composites. Mater Des. 2014;63; doi:10.1016/j.matdes.2014.06.037.
- Zoli L, Medri V, Sciti D, et al. Continuous SiC fibers-ZrB2 composites. J Eur Ceram Soc. 2015;35:4371–4376. doi: 10.1016/j.jeurceramsoc.2015.08.008
- Sciti D, Natali Murri A, Medri V, et al. Continuous C fibre composites with a porous ZrB2 Matrix. Mater Des. 2015;85; doi:10.1016/j.matdes.2015.06.136.
- Zoli L, Sciti D. Efficacy of a ZrB2–SiC matrix in protecting C fibres from oxidation in novel UHTCMC materials. Mater Des. 2017;113:207–213. doi:10.1016/j.matdes.2016.09.104.
- Vinci A, Zoli L, Sciti D, et al. Oxidation behaviour of a continuous carbon fibre reinforced ZrB2–SiC composite. Corros Sci. 2017;123; doi:10.1016/j.corsci.2017.04.012.
- Galizia P, Zoli L, Sciti D, et al. Tough salami-inspired Cf/ZrB2 UHTCMCs produced by electrophoretic deposition. J Eur Ceram Soc. 2018;38; doi:10.1016/j.jeurceramsoc.2017.09.047.
- Zhang X, Xu L, Du S, et al. Crack-healing behavior of zirconium diboride composite reinforced with silicon carbide whiskers. Scr Mater. 2008;59:1222–1225. doi:10.1016/j.scriptamat.2008.08.013.
- Zhang SC, Hilmas GE, Fahrenholtz WG. Oxidation of zirconium diboride with tungsten carbide additions. JAm Ceram Soc. 2011;94:1198–1205. doi:10.1111/j.1551-2916.2010.04216.x.
- Dehdashti MK, Fahrenholtz WG, Hilmas GE. Effects of temperature and the incorporation of W on the oxidation of ZrB2ceramics. Corros Sci. 2014;80:221–228. doi:10.1016/j.corsci.2013.11.030.
- Silvestroni L, Melandri C, Sciti D, et al. Relationships between carbon fiber type and interfacial domain in ZrB2-based ceramics. J Eur Ceram Soc. 2016;36:17–24. doi:10.1016/j.jeurceramsoc.2015.09.026.
- Zoli L, Galizia P, Sciti D, et al. On the thermal shock resistance and mechanical properties of novel unidirectional UHTCMCs for extreme environments. Scientific Reports 2018; 8: 9148. doi:10.1038/s41598-018-27328-x.
- Vinci A, Zoli L, Sciti D, et al. Understanding the mechanical properties of novel UHTCMCs through random forest and regression tree analysis. Mater Des. 2018;145:97–107. doi:10.1016/j.matdes.2018.02.061.