Abstract
Data from selected Lower Pontian sandstone reservoir in the Kloštar Field, situated in the Sava Depression (Northern Croatia), were used for mapping with Sequential Indicator Simulations rather than using a classical approach. Such approaches offer better insight in distribution of geological variables or zonal uncertainties in the cases with larger datasets (15 points or more). Obtained maps of porosity and reservoir thickness are presented here along with probability maps of certain selected cut of values of petrophysical parameters. Maps showed distinct sedimentological features that can clearly be observed on the both sets of maps.
1. Introduction
Oil and gas systems are highly heterogeneous rock bodies, yet relatively recently these geological systems, i.e. oil and gas reservoirs, were regarded as homogeneous. This assumption was made to simplify highly complex subsurface relations in order to facilitate reservoir description. Today, contemporary methods in geological research enable these systems to be described more accurately. Geostatistical methods, used as advanced geological tools, are often applied in reservoir model development. With this approach, a highly detailed and reliable reservoir description can be achieved. Geostatistical methods (deterministic and stochastic) can only be used with a sufficient number of input data points; this is hard to define, but generally should not be less than 10 or 15 for highly anisotropic data sets (CitationMalvić, 2008). In a set with a low number of data points, it is recommended to perform cross-validation using an appropriate interpolation method for every geological variable (CitationMalvić & Đureković, 2003). Stochastic models provide various solutions for a single input data set which may be similar (but never identical) with all solutions equally probable. Sequential Indicator Simulations (SIS) have been used as stochastic models to map three geological variables in the Lower Pontian reservoir in the Kloštar Field. The large number of wells drilled (approximately 170) in this field makes it extremely well suited for such analyses, a judgment which has been proven in previous research studies that included testing of different mapping approaches (CitationBalić et al., 2008), structural and palinspastic mapping (CitationMalvić & Jović, 2012; CitationVelić, et al., 2011), and neural-network analyses (CitationCvetković & Velić, 2012; CitationCvetković, et al., 2009). The Kloštar Field is located SE of Croatia's capital, Zagreb (). Geologically, this area belongs to the Sava Depression, which is a part of the Pannonian Basin system (PBS).
Figure 1. Kloštar Field location (CitationVelić et al., 2011)
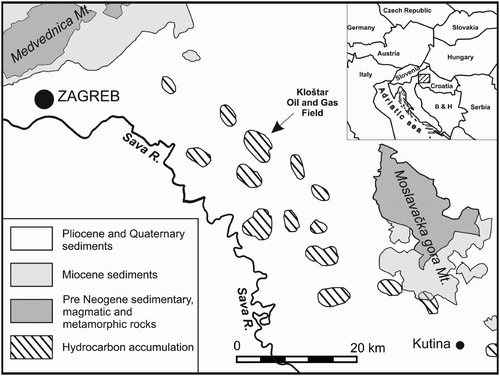
2. Depositional history, stratigraphy, and tectonics of the Sava Depression and Kloštar Field
Evolution of the PBS began in the Early Miocene. Its development continued during the Miocene, Pliocene, and Quaternary (). According to CitationRoyden (1988), the beginning of the extension of the Pannonian Basin is assumed to have occurred in the Ottnangian and Karpatian. Sedimentation areas at the time of extension in Croatia were the marginal parts of the Medvednica, Papuk, and Psunj Mountains and the lowest parts of the Sava, Drava, Slavonia-Srijem, and Mura Depressions (e.g. CitationMalvić, 2003; CitationVrbanac, 1996). According to CitationĆorić et al. (2009), the oldest marine Miocene sediments in the Croatian part of the Pannonian Basin System (CPBS) were Badenian. There is an unconformity between these and the Paleozoic and Mesozoic basement. During the Badenian (16.4–13.0 Ma; CitationHaq & Eysinga, 1998), the transtension became stronger. Pull-apart basins were opened, as well as some smaller depressions, which formed structures in, e.g. the Sava Depression, such as Kloštar, Okoli, and Križ. According to CitationVrbanac (1996) and CitationRögl (1996, Citation1998), most of northern Croatia experienced marine sedimentation during the Badenian, while today's high mountains (Kalnik, Papuk, Psunj) were islands. Siliciclastic material was deposited in shallow seas through alluvial deltas. The material source was local, i.e. it originated from Paleozoic and Mesozoic basement rocks in the area. Other plentiful material sources were shallow sea reefs. Under calm shallow conditions, the Sarmatian (13.0–11.5 Ma; CitationHaq & Eysinga, 1998) began. Its start is actually very hard to separate from the top of the Badenian. Terrestrial influence became stronger, and clayey limestones and sandstones were sedimented (CitationRögl, 1996, Citation1998; CitationVrbanac, 1996). The Lower Pannonian () (11.5–9.3 Ma; CitationHaq & Eysinga, 1998) was characterized by brackish shoal filling (CitationRögl, 1996, Citation1998; CitationVrbanac, 1996). There were small deltas on the marginal parts of depressions. Upper Pannonian sedimentation (9.3–7.1 Ma; CitationHaq & Eysinga, 1998) represented considerable environmental change. Sedimentation occurred through cyclic turbidity flows in brackish to freshwater environments (CitationRögl, 1996, Citation1998; CitationVrbanac, 1996) with sandstone morphology following the depositional direction of currents (CitationSaftić, et al., 2003). In general, their direction was north-northwest/south-southeast with the Eastern Alps as the main source of material. Detritus was transported by turbidity currents and redeposited several times before it was finally sedimented (CitationMalvić et al., 2005; CitationŠimon, 1980). Lower Pontian () (7.1–6.3 Ma; CitationHaq & Eysinga, 1998) sediments were deposited by turbidity currents in the deepest part of the sedimentation area. In calm periods, when turbidity currents were inactive, marls, as typical sediments found in calm deep water, were deposited. In the Late Pontian () (6.3–5.6 Ma; CitationHaq & Eysinga, 1998), depositional mechanisms were again local (deltas) and depositional areas were slowly filled. During the Pliocene and part of the Pleistocene () (5.6–0.0 Ma; CitationHaq & Eysinga, 1998), sedimentation continued in the remnants of the Pannonian Lake, filling it with marly clays, marls, and rarely sandy marls, and locally with sands in deltas. Climate changes and basin inversion were active in the Quaternary (CitationFrisch et al., 1998), and the sediments which were deposited are typical of glacial and interglacial periods. The youngest beds are unconsolidated loess, clay, humus, gravel, and sand.
Figure 2. Chrono- and lithostratigraphy overview for SE margin of the Pannonian Basin System (modified after CitationMalvić & Cvetković, 2013).
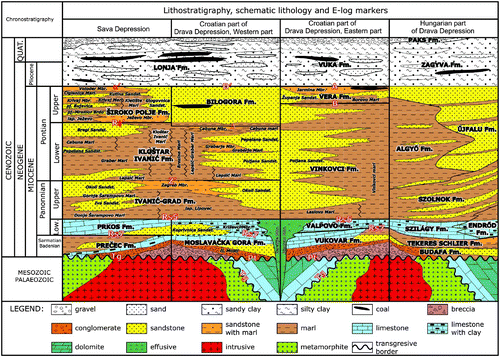
The Kloštar Field is anticline with direction northwest-southeast. A normal fault divides the structure into two parts, northwestern and southeastern. The normal fault represents the termination of reservoir facies and the border of hydrocarbon saturation. It played a key role in Upper Pannonian and Lower Pontian reservoir formation. In the Upper Pannonian, as well as in the Lower Pontian, extension prevailed in the whole area of the CPBS (CitationMalvić & Velić, 2011). During the transition from the Upper Pannonian to the Lower Pontian, the normal fault was active, which caused uplifting of the NE part. NE of the fault and SW of the Moslavačka Gora Mountain, a new, deeper area of sedimentation was created. It is very possible that two sources of material were active: (1) the eastern Alps and (2) Moslavačka Gora Mountain, with local eroded material transported through alluvial fans. The Kloštar Field is located on the edge of the Sava Depression, and it is logical to conclude that part of its material came from Moslavačka Gora Mountain. During the Late Pontian, transpression began and is still active today.
3. Applied mapping methods
SIS are very often used for lithofacies mapping. It was already known that there are more than two lithofacies in the Kloštar Field, and therefore this method was used to demonstrate reservoir heterogeneity. Besides mapping basic reservoir variables like porosity, permeability, reservoir depth, and thickness, lithofacies maps are very important, especially in hydrocarbon reservoir modeling. A precise description of lithofacies type is required because it has a direct influence on the distribution of petrophysical parameters.
With stochastic applications, more simulations can be obtained, which are very much alike, but not identical. The basic characteristic of these models is that they are all equally probable. Because they are all equally probable, it is not possible to choose one model as the best. As stochastic systems are characterized by randomness and contain uncertainties, it is possible to map the highest-uncertainty areas using a series of simulations; stochastic models are therefore highly applicable to reservoir characterization (CitationMalvić, 2008). Many published books and papers describe sequential geostatistical simulations and their applications, e.g. CitationHand et al. (1994), CitationDamayanti & Hicks (1996), CitationDeutsch & Journel (1997), CitationSahin & Al-Salem (2001), CitationMalvić (2008), and CitationGeiger (2012).
The first step is to define a grid system above the wells. The first grid node without an estimated value is randomly chosen (the first stochastic element), and a kriging estimation is performed for this grid point. The second stochastic element is introduced by choosing a figure randomly from the standard normal distribution at this grid point. This figure is the new estimated value at the grid point. This randomly chosen figure is now part of the input data set. In the same way, values at all other grid nodes can be estimated to create the first simulation. By choosing a new way of visiting grid nodes, a new stochastic simulation can be created. Usually, 100 simulations are performed. SIS assumes only third-order stationarity, which means that variograms are the only representative statistical feature. Because indicator transformations are used, the source data are changed into binary variables (0 or 1) and so the variograms for every cutoff value had to be standardized. The source data set is always the same, which means that the input variance is also always the same despite the differences in the output data sets. The differences in the output data sets are attributable to indicator transformation. The results of SIS are models that show values of the mapped variables and probability maps for different cutoffs. In this case, they show the probability that the mapped variable is higher than a certain cutoff value.
4. Mapping the input dataset
Indicator-based methods imply an indicator transformation of the variables based on a certain number of cutoff values into binary values of 0 or 1 and a variogram analysis for every cutoff. The mapped indicator variables were the porosity and thickness of the single reservoir, which will for the purpose of this paper be called reservoir ‘A’. Porosity values represent average well log porosities measured in 20 wells (). The well log used for porosity calculation was spontaneous potential (SP) because the wells were old and only conventional logging was performed in them. Furthermore, the porosity value in well K-12 was extremely small compared to values from other wells (). Because of this, well K-12 was excluded from the calculations, i.e. mapped as an outlier as described by CitationNovak Zelenika et al. (2010). The remaining 19 wells represent a relatively small statistical sample for which the mean and variance are not representative, i.e. they are outside the statistically significant limit of 95%.
Table 1. Analyzed variables values of the reservoir ‘A’ of the Kloštar Field.
5. Sequential indicator simulation results
The porosity and thickness of the ‘A’ reservoir were chosen as variables to describe the facies (depth was not used because of the strong influence of younger tectonics). One hundred realizations were created with realizations 1, 50, and 100 presented, including variable values and probabilities for every cutoff value. Increased porosity values in all realizations (Map 1) can be observed in the central part of the map. In the first realization of the porosity distribution (Map 1), high porosities occur in the central part and in northern and southern areas. The lowest estimated porosity value is approximately 5% in the 100th realization (Map 1) in the northern and central parts of the reservoir. This area in all other realizations had an estimated porosity between 18% and 19%. The eastern part of the reservoir also showed differences in all realizations. Those differences point to the highest uncertainties caused by lateral facies changes.
Realizations of thickness over the whole mapped area show uniform values close to the average. Although they are not huge, small differences exist. Neither porosity nor thickness (Map 1) maps show structural elements or depositional channels.
In addition to a value scale, SIS can also show the probability that a variable value is higher than a certain cutoff value. Such maps are shown as Map 2 for porosity and as Map 3 for thickness of the ‘A’ reservoir. A main channel direction and the existence of different lithofacies on the porosity maps can be observed. The same direction can also be seen on the thickness map for a cutoff value of 13 m (Map 3).
6. Discussion and conclusions
In a Lower Pontian reservoir, 20 well data points were used for mapping. A solution to the problem of how to demonstrate two or more lithofacies was obtained by porosity and thickness mapping. The assumption that higher porosity represents a sandy lithofacies and lower porosity a marly lithofacies was used. In this way, it was possible to distinguish sandstones, marly sandstones, sandy marls, and pure marls. It was also assumed that higher thicknesses should point to the central part of the depositional channel, where the coarsest material was deposited.
There are some uncertainties in SIS mapping, which are related mostly to the number and definition of cutoffs as well as to the presentation of the representative realizations. According to CitationNovak Zelenika et al. (2010), the recommended number of cutoffs for this type and size of reservoir is between 5 and 11. Although normality is not a condition for indicator-based mapping, CitationNovak Zelenika et al. (2011) proved that defining cutoffs so that input data histograms follow a normal distribution can be very useful. Following these two recommendations, the cutoffs used for SIS of the Lower Pontian reservoir were defined. Representative realizations (numbers 1, 50, and 100) were chosen. Because all realizations are equally probable, presentation of realizations 1, 50, and 100 is statistically justified and equally valuable as a presentation of realizations P5, P50, and P95. Another approach is to perform E-type realization, which is a point-by-point average of all realizations. It is the closest to the P50 realization.
Simulations gave good results for porosity distribution in the ‘A’ reservoir. Probability maps for certain cutoff values provided the material transport direction and the distribution channel location. Additional depositional channels and a N-S transport direction for the Lower Pontian reservoirs could also be observed. In this way, the direction of regional turbid transport, which was described by CitationVrbanac et al. (2010), was demonstrated and supplemented with local changes in the Kloštar structure. In the Pliocene and Quaternary periods, regional transpression caused structural inversion in the Kloštar area, when the Kloštar anticline and traps assumed their present form.
Software
Sequential Indicator Simulation maps of porosity, depth and thickness were made using Statios WinGslib™ software. The final layout of the maps were made using CorelDRAW X6™
Main Map: Sequential Indicator Simulation Maps of Porosity and Thickness Distribution in Reservoir ‘A’ in Kloštar Field, Croatia
Download PDF (4.7 MB)Acknowledgements
This work is part of a project funded by University of Zagreb in 2012 titled ‘Geomathematical methods for maintaining or increasing of hydrocarbon production in clastic reservoirs in the Pannonian Basin’ and multidisciplinary research project, ‘Stratigraphical and geomathematical researches of petroleum geological systems in Croatia’ (project no. 195-1951293-0237) and ‘Evidence of Biotic and Abiotic Changes in Palaeoenvironments’ (project no. 119-1951293-1162) financed by the Ministry of Science, Education and Sports of the Republic of Croatia. The authors would also like to thank Dr. Lidija Galović, Stuart Gill, Dr. Mike Smith and an anonymous reviewer for the improvements to the manuscript and maps.
References
- Balić , D. , Velić , J. and Malvić , T. 2008 . Selection of the most appropriate interpolation method for sandstone reservoirs in the Kloštar oil and gas field . Geologia Croatica , 61 ( 1 ) : 27 – 35 .
- Ćorić , S. , Pavelić , D. , Rögl , F. , Mandic , O. , Vrabac , S. , Avanić , R. , Jerković , L. and Vranjković , A. 2009 . Revised middle Miocene datum for initial marine flooding of North Croatian basins (Pannonian basin system, central paratethys) . Geologia Croatica , 62 ( 1 ) : 31 – 43 .
- Cvetković, M., & Velić, J. (2012). Successfulness of inter well lithology prediction on Upper Miocene sediments with artificial neural networks, Geomathematics as geoscience, Malvić, T., Geiger, J. & Cvetković, M. (eds.), Zagreb, Hrvatsko geološko društvo, 13–20.
- Cvetković , M. , Velić , J. and Malvić , T. 2009 . Application of neural networks in petroleum reservoir lithology and saturation prediction . Geologia Croatica , 62 ( 2 ) : 115 – 121 .
- Damayanti , M. C. and Hicks , P. J. 1996 October 23–25 . A geostatistical study of a Pilot Area in the Griffithsvile oil field. Technical advances going into the 21st century, Society of Petroleum Engineers, 37332, Columbus OH 93 – 106 .
- Deutsch , C. V. and Journel , A. G. 1997 . GSLIB: Geostatistical software library and user's guide , 2 , 369 New York : Oxford University Press .
- Frisch , W. , Kuhlemann , J. , Dunkl , I. and Brügel , A. 1998 . A palinspastic reconstruction and topographic evolution of the Eastern Alps during the Tertiary tectonical evolution . Tectonophysics , 297 : 1 – 15 . (doi:10.1016/S0040-1951(98)00160-7)
- Geiger , J. 2012 . “ Some thoughts on the pre- and post-processing in sequential gaussian simulation and their effects on reservoir characterisation ” . In New horizons in Central European geomathematics, geostatistics and geoinformatics , Edited by: J. , E. , Pál-Molnár , T. and Malvić . 17 – 34 . Szeged : Geolitera . 171 p
- Hand , J. L. , Yang , C.-T. and Moritz , A. L. 1994 September 25–28 . Ability of geostatistical simulations to reproduce geology: A critical evaluation. SPE Annual Technical Conference and Exhibition, Society of Petroleum Engineers, 28414 New Orleans, Louisiana, pp. 533–545
- Haq , B. U. and Eysinga , F. W. B. 1998 . Geological time table , 5 , Amsterdam : Elsevier Science . (Wall Chart)
- Malvić, T. (2003). Naftnogeološki odnosi i vjerojatnost pronalaska novih zaliha ugljikovodika u bjelovarskoj uleknini [Oil-Geological Relations and Probability of Discovering New Hydrocarbon Reserves in the Bjelovar Sag] (Unpublished PhD Thesis). Faculty of Mining, Geology and Petroleum Engineering, University of Zagreb.
- Malvić, T. (2008). Primjena geostatistike u analizi geoloških podataka, INA-Industry of Oil Plc., 1st ed., 103 pp.
- Malvić , T. and Cvetković , M. 2013 . Lithostratigraphic units in the Drava Depression (Croatian and Hungarian parts) – a correlation . Nafta , 64 ( 1 ) : 27 – 33 .
- Malvić , T. and Đureković , M. 2003 . Application of methods: Inverse distance weighting, ordinary kriging and collocated cokriging in porosity evaluation, and comparison of results on the Beničanci and Stari Gradac fields in Croatia . Nafta , 54 ( 9 ) : 331 – 340 .
- Malvić , T. and Jović , G. 2012 . Thickness maps of Neogene and Quaternary sediments in the Kloštar Field (Sava Depression, Croatia) . Journal of Maps , 8 ( 3 ) : 1 – 32 . (doi:10.1080/17445647.2012.710463)
- Malvić, T., & Velić, J. (2011). Neogene Tectonics in Croatian Part of the Pannonian Basin and Reflectance in Hydrocarbon Accumulations. U: New Frontiers in Tectonic Research: At the Midst of Plate Convergence, Schattner, U. (eds.), InTech, Rijeka, 215–238.
- Malvić , T. , Velić , J. and Peh , Z. 2005 . Qualitative-Quantitative analyses of the influence of depth and lithological vomposition on lower pontian dandstone porosity in the central part of Bjelovar Sag (Croatia) . Geologia Croatica , 58 ( 1 ) : 73 – 85 .
- Novak Zelenika , K. , Malvić , T. and Geiger , J. 2010 . Mapping of the Late Miocene sandstone facies using Indicator Kriging . Nafta , 61 ( 5 ) : 225 – 233 .
- Novak Zelenika, K., Velić, J., Malvić, T., & Cvetković, M. (2011). Geological variables fitting in normal distribution and application in indicator geostatistical methods. Proceedings of IAMG 2011 Conference, held in September 5–9 2011, Salzburg, 245–251.
- Royden, L. H. (1988). The Pannonian Basin. Tulsa, OK: American Association of Petroleum Geologists, 390 pp.
- Rögl , F. 1996 . Stratigraphic correlation of the paratethys oligocene and Miocene . Mitteilungen Ges. Geol. Bergbaustudenten Österreich , 41 : 65 – 73 .
- Rögl , F. 1998 . Palaeographic consideration for mediterranean and paratethys seaways (Oligocene to Miocene) . Ann. Naturhist. Mus. Wien , 99A : 279 – 310 .
- Saftić , B. , Velić , J. , Sztano , O. , Juhas , G. and Ivković , Ž. 2003 . Tertiary subsurface facies, source rocks and hydrocarbon reservoirs in the SW part of the Pannonian Basin (northern Croatia and south-western Hungary) . Geologia Croatica , 56 ( 1 ) : 101 – 122 .
- Sahin , A. and Al-Salem , A. A. 2001 . Stochastic modeling of porosity distribution in a multi-zonal carbonate reservoir , 1 – 11 . Society of Petroleum Engineers . 68113. SPE Middle East Oil Show, 17–20 March 2001, Bahrain
- Šimon, J. (1980). Prilog stratigrafiji u taložnom sustavu pješčanih rezervoara Sava-grupe naslaga mlađeg tercijara u Panonskom bazenu sjeverne Hrvatske. Doktorska disertacija, Rudarsko-geološko-naftni fakultet Sveučilišta u Zagrebu, 66 str., Zagreb.
- Velić , J. , Malvić , T. and Cvetković , M. 2011 . Palinspastic reconstruction of synsedimentary tectonics of Neogene and Quaternary sediments in the Kloštar Field (Sava Depression, Pannonian Basin, Croatia) . Zeitschrift der Deutschen Gesellschaft für Geowissenschaften , 162 ( 2 ) : 193 – 203 . (doi:10.1127/1860-1804/2011/0162-0193)
- Vrbanac, B. (1996). Paleostrukturne i sedimentološke analize gornjopanonskih naslaga formacije Ivanić-Grad u Savskoj depresiji (Unpublished PhD Thesis). Faculty of Scineces, University of Zagreb, 121 p.
- Vrbanac, B., Velić, J., & Malvić, T. (2010). Sedimentation of deep-water turbidites in main and marginal basins in the SW part of the Pannonian Basin. Geologica Carpathica, 61(1), 55–69.