ABSTRACT
Structural glaciology yields important details about the evolution of glacier dynamics in response to climate change. The maps provided here document the occurrence and evolution of brittle and ductile structures on the tongue of Forni Glacier, Ortles-Cevedale Group, Central Italian Alps, between 2003 and 2014. Through the remote sensing-based analysis of structures, we found evidence of brittle fractures such as crevasses, faults and ring faults, and ductile structures such as ogives at the base of the icefall in the eastern glacier tongue. Although each of the three glacier tongues have evolved differently, a reduction in flow-related dynamics and an increase in the number of collapse structures occurred over the study period. Analysis of the glacier structural evolution based on the numbers and the locations of different structures, suggest a slowdown of glacier flow on the eastern tongue. The recent evolution of the glacier also suggests that the occurrence of a disintegration scenario is likely to worsen over the next decades.
1. Introduction
Mountain glaciers are rapidly thinning, with the last decade setting an unprecedented record for mass losses. Considering the glaciological balance, the global mean annual value of early twenty-first-century observations (2001–2010) is the most negative since the mid-nineteenth century, with −0.54 m w.e. a–1 and glaciers presently contribute to 1.37 mm a−1 of mean sea-level rise (CitationZemp et al., Citation2015). In the Alps, persistently negative mass balance in the twenty-first century is linked to increased summer melt, while winter balances show no significant trend (CitationWGMS, Citation2015). Glaciers adjust to mass perturbations by changing their dynamics, as the distribution of stresses within the glacier is altered, and improving the understanding of these processes is a crucial step in generating predictive models of glacier evolution (CitationHambrey & Lawson, Citation2000). The analysis of glacier structures can provide insights into both short- and long-term variations in glacier dynamics, with an array of distinctive glacier structures forming due to ductile and brittle ice deformation. Ductile structures such as folds and foliation reflect cumulative strain, and thus long-term strain histories, by developing from primary stratification of snow or crevasse traces under high strain or through rotation by glacier flow (CitationBenn & Evans, Citation2010). Crevasses, on the other hand, open whenever the forces pulling ice apart exceed the strain/stress resistance of the ice. They are generally confined to the shallower glacier layers and reflect patterns of extensional stress (CitationCuffey & Paterson, Citation2010). A number of studies have conducted detailed structural surveys of polar and Alpine glaciers (e.g. CitationGoodsell, Hambrey, & Glasser, Citation2002; CitationGoodsell, Hambrey, Glasser, Nienow, & Mair, Citation2005; CitationHambrey & Lawson, Citation2000; CitationJennings, Hambrey, Glasser, James, & Hubbard, Citation2016), but a few have assessed the temporal evolution of glacier structures in response to evolving stress and strain regimes (CitationGlasser & Scambos, Citation2008; CitationHambrey et al., Citation2005; CitationPhillips et al., Citation2017). This study aims to map brittle and ductile structures originated from the glacier dynamics, by means of the analysis of high-resolution orthophotos, and to describe structural controls of glacier disintegration for a rapidly downwasting Alpine glacier by investigating structure evolution. In this paper, we describe the structures identified on orthophotos acquired in 2003, 2007, 2012, and 2014 (Section 3), then we assess spatial patterns and their evolution (Section 4) and provide explanations for the trends in relation to glacier dynamics (Section 5).
2. Study area
Forni Glacier in the Ortles-Cevedale group (Stelvio National Park, Central Italian Alps) is one of the largest Italian glaciers (see ). The new Italian glacier inventory, based on 2007 data (CitationSmiraglia et al., Citation2015) reported an area of 11.34 km2, an elevation range between 2501 and 3764 m a.s.l. and an NNW aspect. In 2012, the glacier surface decreased to 10.83 km2 (unpublished data). The glacier tongue is up to 120 m thick at the base of the central ice fall, but has an average thickness of 30–40 m (CitationUrbini et al., Citation2017) and the equilibrium line altitude is approximately between 2800 and 3200 m a.s.l. (CitationGaravaglia et al., Citation2014). High ice thinning rates, up to –5 m w.e. a−1 at 2700 m a.s.l, have been measured on the eastern sector of the tongue (CitationSenese, Diolaiuti, Mihalcea, & Smiraglia, Citation2012).
Forni glacier used to be composed of three separate accumulation basins, joining into a single tongue and separated by medial moraines but in 2015, ice flow was interrupted at the base of the Vioz icefall on the eastern tongue (see (B) and ) and the glacier can now be actually viewed as split into two separate ice bodies. Supraglacial debris cover (mainly derived from macrogelivation processes, rockfalls, and landslides affecting the mountain walls and lateral moraine ridges) is now abundant on the glacier surface, particularly at the terminus and on the western tongue, which is almost entirely debris-covered. The differential ablation rates between debris-covered and debris-free areas have produced glaciokarst features such as dirt cones, epiglacial lakes, and cavities (CitationDiolaiuti & Smiraglia, Citation2010).
3. Methods
3.1. Data sources
Three sets of orthophotos acquired through aerial surveys in the summer of 2003, 2007 and 2012 and orthophotos acquired by unmanned aerial vehicle (UAV) survey in 2014 were the primary data source of this study. The orthophotos are purchasable products of BLOM CGR (Compagnia Generale Riprese Aeree) at a nominal scale of 1:10,000 with a square pixel size of 0.5 m × 0.5 m (accuracy stated by BLOM CGR is ± 1 m). In addition, an orthophoto of the glacier tongue was acquired by means of a UAV survey in summer 2014, at a flying height of 250 m, see CitationFugazza et al. (Citation2015) for further details on the survey. The spatial resolution of the UAV orthophoto is 0.15 m × 0.15 m; as no ground control points were collected during the survey and georeferencing relied only on the GPS antenna on board the aircraft, we checked the horizontal accuracy of this orthophoto at stable points (bends of roads, large boulders, buildings) outside the glacier outlines and found a root mean square error of 0.91 m.
We limited our structural survey to the ablation zone of the glacier due to (1) the reduced coverage of the UAV orthophoto compared to the other available images; and (2) the focus on glacial disintegration, highlighted by the evolution of structures on the glacier tongue. The latter is entirely snow-free in all orthophotos except 2007, where some residual snow is present inside some crevasses and depressions and on the higher tongue sectors; thus, most of the structures can be clearly distinguished. The mapping was carried out on the 2007 orthophoto regardless of snow conditions, to provide further insights particularly into the evolution of the eastern section of the glacier. To identify the glacier terminus and lateral margins, we used the glacier outlines from 2003 and 2007, produced by CitationD’Agata, Bocchiola, Maragno, Smiraglia, and Diolaiuti (Citation2014), while outlines from the years 2012 and 2014 were manually digitized from the respective orthophotos as part of this study. The upper limits of the glacier tongue were identified at the base of the western, central and eastern ice falls, entirely covered by the 2014 orthophoto.
3.2. Description of glaciological structures
The structures identified on the orthophotos are mainly of the brittle type, that is, crevasses, crevasse traces, normal faults and ring faults, with the latter two being rarely described on glaciers (). The only ductile deformation structures evident were ogives, and their occurrence was confirmed by means of field surveys on the glacier. Although traces of foliation were also identified, on the orthophotos and during field surveys, few of these structures can be clearly distinguished on the orthophotos, and therefore, they were not considered in this study. Mapping of crevasses and crevasse traces on orthophotos follows the criteria laid out by CitationGoodsell, Hambrey, Glasser, Nienow, and Mair (Citation2005), while ogives were mapped and classified based on the description provided by CitationGoodsell et al. (Citation2002). All identified structures were digitized using GIS software; digitized features were then classified as crevasses, crevasse traces, faults, normal faults and ring faults within a database. Crevasse orientation was calculated from digitized segments and analyzed using circular statistics (CitationFisher, Lewis, & Embleton, Citation1993). In the text, it is reported using cardinal direction or as ‘oblique’ or ‘transverse’ with respect to flow direction. We divided the glacier area according to three glacier tongues, to identify the trends occurring on the main flow units of Forni Glacier.
Figure 3. Examples of glaciological structures identified on Forni Glacier in the field. (A) Crevasses and crevasses traces (marked with black arrows) on the central glacier tongue. (B) Normal faults cutting the glacier moraine (marked with white arrows). (C) Ring faults located near the margin of the eastern tongue (marked with black arrows; the evolution of this feature is reported in ). (D) Circular ring faults (marked with black arrows) located on the Eastern tongue, downvalley from the icefall.
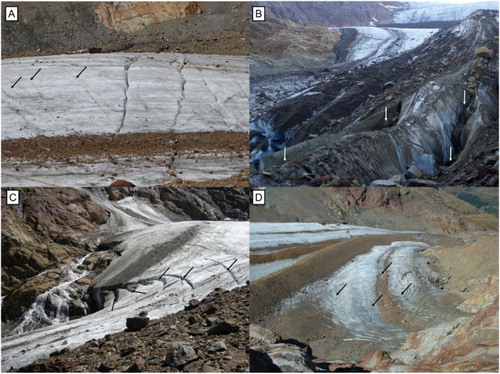
3.2.1. Crevasses
On orthophotos, crevasses mostly appear as dark lines in debris-free areas when their aperture is small, due to shadows projected by crevasse walls, or white lines when they are filled with snow, as was seen in 2007 ((A)). On the debris-covered ice, crevasses also appear as light linear structures as the ice is revealed within crevasse walls. The distribution and preferred orientation of these features are not uniform on the glacier surface, but major spatial groups can be distinguished: at the eastern and western valley margins, chevron crevasses are often found, pointing between a few degrees and almost 90° upvalley, due to lateral gradients in ice velocity (CitationBenn & Evans, Citation2010). In all years, the eastern tongue appears to be the most intensely crevassed, particularly at the valley margins, and where the direction of glacier flow is rotated due to the morphology of the valley, with several intersecting transverse and longitudinal fractures ((A) and ). Splaying crevasses are also found at its terminus, where the ice spreads out laterally, although they are most abundant in the earliest set of imagery from 2003.
Figure 4. Examples of glaciological structures of Forni Glacier as seen on the orthophotos. (A) Chevron crevasses (2014 orthophoto); (B) Crevasse traces (2012); (C) Normal faults (2012); (D) Ring faults (2014); (E) Ogives (2014; the dark ice bands are marked with black arrows, the light ice ones with white arrows).
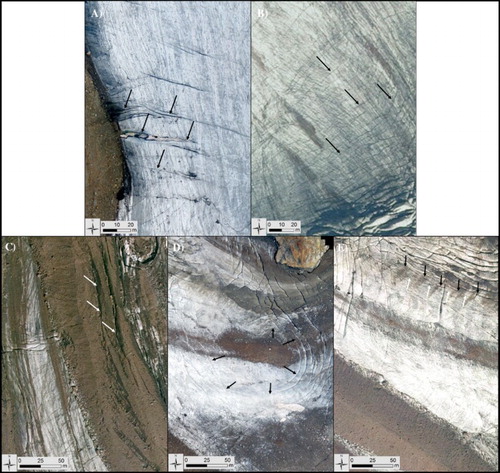
Table 1. Total length and density (km per km2) of structures mapped on the four orthophotos.
3.2.2. Crevasse traces
Crevasse traces also appear as thin dark lines, initially linear and progressively deforming into arcuate structures downvalley and are transverse to main flow direction. They can form when open crevasses move into areas of compressive glacier flow and close, as the lateral continuation of open crevasses or as individual features analogous to tensional veins in rocks (CitationHambrey & Lawson, Citation2000). On Forni Glacier, they are exclusively found on the central tongue in an area of compressive flow at the base of the icefall ((A) and 4(B)), which suggests the closure of open crevasses as the most likely mechanism of formation.
3.2.3. Normal faults
Normal faults also appear as dark or grey linear structures and their classification as faults is thus based on (1) their location on the medial moraines and (2) their orientations, ranging from subparallel to the moraine main axis to transverse ((B) and (C)), thus not coherent with glacier flow and related to the gravitational collapse of steep moraine slopes. Lines appear darker owing to shadows when vertical displacements are higher, while brighter areas suggest the presence of ice-cemented debris within fault scarps. On Forni Glacier, these structures were observed particularly at the terminus of the eastern medial moraine, with an offset ranging between 1 and 5 m.
3.2.4. Ring faults
These structures consist of dark lines forming circular and semicircular concentric rings, and are also unrelated to glacier flow. Ring faults geometry is, in fact, a typical feature of volcanic systems, where magma chambers generate a local stress field triggering caldera collapse (CitationGudmundsson, Marti, & Turon, Citation1997). In glaciated environments, ring faults were observed by CitationBranney and Gilbert (Citation1995) in Rio de los Huemules Valley, Chile, after the eruption of Volcan Hudson. Eruption-induced ice melt of a nearby glacier generated lahars which transported ice blocks to the valley; subsequent melting of debris-mantled ice then generated an array of ring-shaped features. CitationKenneally and Hughes (Citation2002) observed ring faults on Deception Island, also following a volcanic eruption that produced a crater near the terminus of the ice sheet and the consequent formation of ring-shaped structures on the ice wall. The authors also reported the formation of arcuate crevasses intersecting ring faults, oriented at 45° with respect to maximum shear stresses.
On Forni Glacier, the local stress field likely originates from basal or englacial meltwater, producing voids in the ice-bedrock interface and consequent collapse of glacial cavities. Ring faults are found on all orthophotos, particularly at the margins of the eastern tongue, although intersecting crevasses are identifiable only on a few occasions ((C,D) and (D)). Semicircular structures also appear on the western tongue and adjacent medial moraine and at the glacier terminus, owing to the collapse of the ice-cored moraine or melt out of debris-covered ice, exposing clean ice underneath the debris layer.
3.2.5. Ogives
The ogives observed on Forni Glacier can be classified as band ogives, that is, formed by repeated bands of light and dark ice ((E) convex in flow direction (CitationGoodsell et al., Citation2002). Ogives are found exclusively at the base of the eastern and central icefall, the latter in 2003 only, whereas no ogives can be seen below the western icefall. On the eastern tongue, ogives are located about 350 m downvalley from the icefall and their wavelength (i.e. the distance between two dark ice bands) is approximately between 15 and 30 m. Although their mechanism of formation is not entirely clear, the most widely accepted explanation is that they reflect the passage of ice through an icefall in different seasons (CitationGoodsell et al., Citation2002).
4. Results
4.1. Distribution and evolution of structures on the glacier tongue
The structural pattern of the glacier tongue was investigated by analyzing the main groups of structures identified on each single orthophoto, and comparing them year-by-year (see Main Maps and ).
4.1.1. 2003
On the western tongue (see Main Map A), crevasses mainly exhibit a NNE-SSW orientation toward the terminus, while those at the base of the icefall are instead oriented NE-SW. A stable zone without crevasses also exists at the south-eastern margin of the tongue.
On the central tongue, four distinct zones can be recognized. The first area, close the southern margin, is composed of crevasse traces, forming a network of subparallel transverse structures, arcuate and convex in flow direction, with increasing distance between them downvalley. West of this area, crevasses are mainly oblique, while transverse crevasses can be seen further downvalley. At the terminus, normal faults appear on the western medial moraine and highly debris-covered areas.
Four zones can also be distinguished on the eastern tongue. To the south, normal faults cut across the eastern medial moraine, whereas ogives are found close to the northern margin, with a preferential SW-NE orientation and an average wavelength of about 20 m. North of this area is a set of longitudinal structures. At the valley margin, chevron crevasses initially point upvalley at angles lower than 45° and are detached from the rock by a randkluft (i.e. a marginal crevasse located between the glacier and the adjacent rock wall). Following the margin, they change their orientation to near N-S. The last 250 m from the terminus are dominated by ring faults and splaying crevasses close to the eastern margin ((A)), while to the west normal faults appear subparallel to the medial moraine.
4.1.2. 2007
The number of structures visible on the 2007 orthophoto (see Main Map B) is significantly lower than in the previous year (see ), mostly due to snow covering the glacier tongue. On the western tongue, the preferential orientations of crevasses are the same as in 2003, whereas most structures on the central tongue have a WNW-ESE orientation. The eastern tongue has retreated significantly (from 0.38 to 0.31 km2), with the new glacier outline following the location of ring faults identified on the previous orthophoto, and the formation of an ice-contact lake in place of a former ring fault ((B)). Due to snow cover, only the south-eastern side of ogives is visible, but the preferential orientation and average wavelength of these structures are the same as in 2003.
4.1.3. 2012
On this orthophoto, significant changes appear to have occurred on all three sections of the tongue (see Main Map C), with an increase in the density of structures compared to 2003 on the eastern and central tongue (+49% and +146%, respectively) and a decrease on the western tongue (−434%). Here, crevasses do not appear at the valley margin any longer, while the total length of ring faults has increased (+79%). This section of the tongue is either debris covered or included in the western medial moraine, the two surfaces being distinguished only by slight chromatic differences.
On the central tongue, crevasse traces are still visible at the base of the icefall, with the same orientation as in previous years. The same is true for chevron crevasses at the western margin. However, where transverse crevasses were found in previous years, normal faults are now open in a NW-SE direction on the western and eastern medial moraine.
On the eastern tongue, three distinct structure groups are evident: the first, to the east, exhibits ring faults, in an area where no structures were found before. New chevron crevasses oriented ESE-WNW follow the new glacier margin, intersecting a network of structures oriented NNW-SSE. Ring faults appear further downvalley, with evidence of a meltwater infill. The last 600 m from the terminus show again evidence of semicircular structures subparallel to the medial moraine and rotated E-W towards the valley margin. The position and the wavelength of ogives mirror those of previous years.
4.1.4. 2014
A further increase in the number of structures seems to have occurred between 2012 and 2014 (see Main Map D and ). Although the quantitative comparison might be affected by the higher spatial resolution of the latter orthophoto, the observation of the eastern tongue reveals an impressive number of fractures not found in previous years, while on the western tongue, the only structures found are normal faults and few chevron crevasses.
On the central tongue, the same distinct groups can be recognized as in 2012, with an increased density. In the northern part of the tongue, structure orientations are now predominantly ESE-WNW and semicircular ring faults have developed. The medial moraine now covers the last 600 m from the terminus, and new normal faults have formed here.
On the eastern tongue, ring faults increased between 2012 and 2014. Most crevasses are now oriented N-S or subparallel to the tongue, while fewer spread out laterally at the terminus. The orientation of ogives appears to have rotated a few degrees north. Their former location at the base of the icefall now hosts a large ring fault, so they are only visible at the lowest elevations and their total length decreased to a third of the 2003 estimate.
5. Discussion
The three sections of the tongue show different behaviors in relation to the glacier dynamics and their evolution. Specifically, the progressive disintegration of the western tongue exemplifies the processes occurring in glacier marginal zones, with the collapse of unstable moraines and glacial cavities. In 2003, its structures suggested a regime of lateral shear stress, involved in the formation of chevron crevasses towards the terminus and crevasses at the base of the icefall, in combination with extensional stress. The absence of crevasses east of the icefall was instead likely due to the compressive regime at the base of the icefall and the rotation of the valley from a W-E to N-S orientation. Over the observation period, this section of the tongue progressively shrank; 2007 first saw evidence of rock outcrops and cavities, which then collapsed with the formation of ring faults in 2012. In 2014, the western tongue further reduced in extent, following the profile of ring faults, and the only structures still present are faults due to moraine collapse or chevron crevasses, suggesting that residual shear stress still exists in some areas.
The central tongue is the widest and its icefall did not shrink significantly between 2003 and 2014, which might explain its greater stability. Crevasse traces are found in all years in a zone of compressive flow at the base of the icefall, whereas oblique crevasses to the west are likely formed through lateral shear stress and increased through the years. Lateral drag is also involved in the formation of transverse crevasses in combination with extensional tensile stress. Compared to this section of the tongue, the eastern medial moraine, however, underwent major changes, first by widening, and then showing signs of collapse with an increase in normal faults starting in 2012. The widening of the medial moraine is favored by the increased availability of debris, related to the ongoing intensification of rock-degradation and macrogelivation, and differential ablation processes. Fault structures caused by the gravitational collapse of its slopes may thus be even more widespread in the future.
The distribution of structures on the eastern tongue can be explained by the rotation of the valley, likely causing intense lateral drag in an area of extensional stress and producing changes in crevasse orientation along the tongue, which can be seen in all years. The evolution is instead influenced by the thinning of its icefall, which reduced mass transfer toward this area. Local thinning of the tongue was already present in 2003, highlighted by a set of longitudinal structures located north of the ogives, an area which saw the melt out of a rock outcrop in 2007. Glacier disintegration is particularly exemplified by the increase in ring faults in 2012 and the formation of a large one in 2014. While the orientation of ogives clearly indicated the NW direction of flow, it is likely that due to the reduction of the eastern icefall, no new ogives formed in recent years, providing evidence of reduced glacier flow.
6. Conclusions
Through the mapping of crevasses, faults, and ogives, we have assessed the structural evolution of the Forni Glacier tongue during 2003–2014. We can speculate that the dynamics of the glacier changed significantly, shifting from a typical ice flow regime toward a disintegration scenario. The changes can be evident on the western and eastern tongue: the former is now entirely debris covered and the once dominant chevron crevasses have been replaced by ring faults; the latter shows a prominent ring fault where ogives formed in previous years. It can be hypothesized that the increasing occurrence of normal faults on the medial moraines and ring faults at the glacier margins is due to the lack of longitudinal stress, which is typically found in a regime of glacier flow, and the reduction of mass input from the accumulation area, leading to progressive glacier downwasting and disintegration.
The study also shows how UAVs can be useful tools for structural glaciology, providing more detailed on-demand mapping capabilities than previously possible with conventional aerial surveys, in particular in dynamic environments such as glaciers. Further UAV surveys will be carried out in the future to continue monitoring the structural evolution of Forni Glacier. Extensive fieldwork will also be carried out to identify ductile structures such as foliation, which cannot be clearly distinguished on the orthophotos, and aid in the reconstruction of past glacier dynamics.
Software
Data analysis was carried out using ArcMap, version 10.2. Figures were produced with ArcMap and Microsoft Publisher 2013.
main_map.pdf
Download PDF (58.8 MB)Acknowledgements
The authors wish to thank the Central Scientific Committee of the Italian Alpine Club (CSC CAI) and the Stelvio National Park (now Stelvio Park-ERSAF Lombardia), for permitting and supporting our field and UAV surveys and IIT Regione Lombardia for providing the aerial orthophotos.
Disclosure statement
No potential conflict of interest was reported by the authors.
ORCID
Roberto Sergio Azzoni http://orcid.org/0000-0002-5931-486X
Davide Fugazza http://orcid.org/0000-0003-4523-9085
Michele Zucali http://orcid.org/0000-0003-3600-7856
Carlo D'Agata http://orcid.org/0000-0002-3337-7301
Davide Maragno http://orcid.org/0000-0003-4725-7244
Claudio Smiraglia http://orcid.org/0000-0001-6635-2074
Guglielmina Adele Diolaiuti http://orcid.org/0000-0002-3883-9309
Additional information
Funding
References
- Benn, D., & Evans, D. J. A. (2010). Glaciers and glaciations (2nd ed.). Abingdon: Routledge.
- Branney, M. J., & Gilbert, J. S. (1995). Ice-melt collapse pits and associated features in the 1991 lahar deposits of Volcan Hudson, Chile: Criteria to distinguish eruption-induced glacier melt. Bulletin of Volcanology, 57, 293–302. doi: 10.1007/BF00301289
- Cuffey, K., & Paterson, W. S. B. (2010). The physics of glaciers (4th ed.). San Diego, CA: Academic Press.
- D’Agata, C., Bocchiola, D., Maragno, D., Smiraglia, C., & Diolaiuti, G. A. (2014). Glacier shrinkage driven by climate change during half a century (1954–2007) in the Ortles-Cevedale Group (Stelvio National Park, Lombardy, Central Italian Alps). Theoretical and Applied Climatology, 116, 169–190. doi: 10.1007/s00704-013-0938-5
- Diolaiuti, G. A., & Smiraglia, C. (2010). Changing glaciers in a changing climate: How vanishing geomorphosites have been driving deep changes in mountain landscapes and environments. Géomorphologie: relief, processus, environnement, 16, 131–152. doi: 10.4000/geomorphologie.7882
- Fisher, N. I., Lewis, T., & Embleton, B. J. J. (1993). Statistical analysis of spherical data (329 pp.). New York, NY: Cambridge University Press.
- Fugazza, D., Senese, A., Azzoni, R. S., Smiraglia, C., Cernuschi, M., Severi, D., & Diolaiuti, G. A. (2015). High-resolution mapping of glacier surface features: The UAV survey of the Forni Glacier (Stelvio National Park, Italy). Geografia Fisica e Dinamica Quaternaria, 38, 25–33.
- Garavaglia, R., Marzorati, A., Confortola, C., Bocchiola, D., Cola, G., Manzata, E., … Diolaiuti, G. A. (2014). Evoluzione del Ghiacciaio dei Forni: la possibile evoluzione del più grande ghiacciaio vallivo italiano attraverso approcci modellistici monodimensionali. Neve & Valanghe, 81, 60–67.
- Glasser, N. F., & Scambos, T. (2008). A structural glaciological analysis of the 2002 Larsen B ice-shelf collapse. Journal of Glaciology, 54, 3–16. doi: 10.3189/002214308784409017
- Goodsell, B., Hambrey, M. J., & Glasser, N. F. (2002). Formation of band ogives and associated structures at Bas Glacier D’Arolla, Valais, Switzerland. Journal of Glaciology, 48, 287–300. doi: 10.3189/172756502781831494
- Goodsell, B., Hambrey, M. J., Glasser, N. F., Nienow, P., & Mair, D. (2005). The structural glaciology of a temperate valley glacier: Haut Glacier d’Arolla, Valais, Switzerland. Arctic, Antarctic, and Alpine Research, 37, 218–232. doi: 10.1657/1523-0430(2005)037[0218:TSGOAT]2.0.CO;2
- Gudmundsson, A., Marti, J., & Turon, E. (1997). Stress fields generating ring faults in volcanoes. Geophysical Research Letters, 24, 1559–1562. doi: 10.1029/97GL01494
- Hambrey, M. J., & Lawson, W. (2000). Structural styles and deformation fields in glaciers: A review. In A. J. Maltman, B. Hubbard, & M. J. Hambrey (Eds.), Deformation of Glacial Materials (Vol. 176, pp. 59–83). London: Geological Society (Special Publications).
- Hambrey, M. J., Murray, T., Glasser, N. F., Hubbard, A., Hubbard, B., Stuart, G., & Kohler, J. (2005). Structure and changing dynamics of a polythermal valley glacier on a centennial timescale: Midre Lovénbreen, Svalbard. Journal of Geophysical Research, 110, 1–19. Article no. F01006. doi: 10.1029/2004JF000128
- Jennings, S. J. A., Hambrey, M. J., Glasser, N. F., James, T. D., & Hubbard, B. (2016). Structural glaciology of Austre Brøggerbreen, northwestern Svalbard. Journal of Maps, 12, 790–796.
- Kenneally, J. P., & Hughes, T. J. (2002). The calving constraints on inception of Quaternary ice sheets. Quaternary International, 95-96, 43–53. doi: 10.1016/S1040-6182(02)00026-5
- Phillips, E., Everest, J., Evans, D. J. A., Finlayson, A., Ewertowski, M., Guild, A., & Jones, L. (2017). Concentrated, ‘pulsed’ axial glacier flow: Structural glaciological evidence from Kvíárjökull in SE Iceland. Earth Surface Processes and Landform. doi: 10.1002/esp.4145
- Senese, A., Diolaiuti, G., Mihalcea, C., & Smiraglia, C. (2012). Energy and mass balance of Forni Glacier (Stelvio National Park, Italian Alps) from a 4-year meteorological data record. Arctic, Antarctic, and Alpine Research, 44(1), 122–134. doi: 10.1657/1938-4246-44.1.122
- Smiraglia, C., Azzoni, R. S., D’Agata, C., Maragno, D., Fugazza, D., & Diolaiuti, G. A. (2015). The evolution of the Italian Glaciers from the previous database to the New Italian Glacier inventory: Preliminary considerations and results. Geografia Fisica e Dinamica Quaternaria, 38, 79–87.
- Urbini, S., Zirizzotti, A., Baskaradas, J., Tabacco, I. E., Cafarella, L., Senese, A., … Diolaiuti, G. (2017). Airborne radio echo sounding (RES) measures on Alpine Glaciers to evaluate ice thickness and bedrock geometry: Preliminary results from pilot tests performed in the Ortles Cevedale Group (Italian Alps). Annals of Geophysics, 60(2), G0226.
- WGMS. (2015). Global Glacier change bulletin no. 1 (2012–2013). M. Zemp, I. Gärtner-Roer, S. U. Nussbaumer, F. Hüsler, H. Machguth, N. Mölg, … M. Hoelzle (eds.) (p. 230). Zurich: ICSU(WDS)/IUGG(IACS)/UNEP/UNESCO/WMO, World Glacier Monitoring Service.
- Zemp, M., Frey, H., Gaertner-Roer, I., Nussbaumer, S. U., Hoelzle, M., Paul, F., … Vincent, C. (2015). Historically unprecedented global glacier decline in the early 21st century. Journal of Glaciology, 61, 745–762. doi: 10.3189/2015JoG15J017