ABSTRACT
A 1:4470 map of the Morsárjökull foreland, southern Iceland shows the spatial distribution of post Little Ice Age glacial sediment–landform associations pertaining to the operation of a typical active temperate outlet glacier of the south Vatnajökull ice cap. The map depicts an exemplar for the debris-charged glaciated valley landsystem, with characteristics further indicative of ‘uncovered alpine glaciers’, such as the uneven distribution of moraine volume, the localized appearance of a fluted subglacial bed and within-valley lateral moraine asymmetry. Such glaciers are characterized by strong glacier-climate coupling, because temporally and spatially sporadic rock avalanche-type medial moraines can only locally retard ablation. This morainic debris also has a short residence time in the glacier system due to its strong coupling with the proglacial fluvial system and resulting in efficient sediment transfer. Areas of more substantial latero-frontal moraine document phases of rock slope failure onto the snout and the passage of the debris to the ice margin.
1. Introduction
The aim of the mapping presented here was to assess, at the scale of 1:4470 (Main Map), the spatial distribution of post Little Ice Age (LIA) glacial sediment–landform associations on the Morsárjökull foreland (), southern Iceland, focussing specifically on the influence of supraglacial debris transport on the active temperate glacial landsystem signature typical of the south Vatnajökull outlet lobes. Recent rock slope failure (RSF) events, such as that observed at Morsárjökull in 2007 (CitationSæmundsson et al., Citation2011) and captured on the aerial photography used in this study, have served to demonstrate the nature of supraglacial debris emplacement, transport and deposition in real time. Therefore, observations on the evolution of landforms associated with the recession of Morsárjökull allow us to erect models of glacial process-form regimes with increasing confidence. Unlike Kvíárjökull, a south coast glacier previously highlighted as an exemplar of the glaciated valley landsystem (CitationBoulton & Eyles, Citation1979; CitationEyles, Citation1983a), Morsárjökull, although short (5 km from icefall to snout), is confined by a deep valley in which both the glacier snout and its proglacial outwash are constrained, and copious extraglacial debris is derived from surrounding rock slopes. These characteristics have resulted in the development of a foreland that serves as an exemplar for the debris-charged glaciated valley landsystem in the area (CitationEyles, Citation1979, Citation1983a, Citation1983b) and one that is also typical of the ‘uncovered alpine glacier’ identified by CitationBenn, Kirkbride, Owen, and Brazier (Citation2003) in their continuum of glaciated valley landsystem types.
2. Methods of map production
The Morsárjökull snout and its foreland were mapped using colour aerial photographs taken by the Airborne Remote Sensing Facility (ARSF) of the Natural Environment Research Council UK in 2007. A total of 66 photographs covering the Öræfajökull outlet glaciers were orthorectified and mosaicked using Agisoft Photoscan Professional Edition. Coordinates for 21 ground control points (GCPs) were collected in September 2013 using digital Global Positioning System over the glacier foreland. Fifteen points were used as ground control points (GCP) to establish absolute orientation for the generation of an orthophotograph and digital elevation model (DEM). A further 6 points were utilized as independent checkpoints to assess the errors. The error in 3D space based on these 23 checkpoints was 0.85 m (x error = 0.32; y error = 0.46 m; z = 0.65 m). Mapping was then undertaken on a coloured ink film overlain on an extract from the orthophotograph pertaining to the area covered by the Morsárjökull snout and its foreland and involved the simultaneous interpretation of surface materials and landforms based on ground truth fieldwork in 2012 and 2013 and the desktop viewing of stereoscopic images. The orthophotograph processing and contour generation were both performed in ESRI ArcGIS. Seven surficial geology map units with their characteristic landforms, as well as areas of bedrock, are identified on and around the glacier foreland and are colour-coded following the protocol established for previous Icelandic glacier foreland maps (CitationBennett, Evans, Carbonneau, & Twigg, Citation2010; CitationEvans & Orton, Citation2015; CitationEvans & Twigg, Citation2002; CitationEvans, Ewertowski, & Orton, 2017; CitationEvans, Shand, & Petrie, Citation2009; CitationEvans, Twigg, & Shand, Citation2006, Citation2007; CitationHowarth & Welch, Citation1969a, Citation1969b). These include till and moraines (ridges of boulder and diamicton accumulations), glacifluvial deposits and landforms (braided spreads and inter-moraine corridors of locally pitted gravels and sands), older glacigenic deposits (lying outside the LIA maximum glacier limits), paraglacial deposits (contained in valley-side fans), moraines constructed from glacifluvial deposits (ice-pushed ridges of gravel and sand), small areas of glacilacustrine sediment at lake margins, recent alluvial fans and weathered bedrock (residuum). Contours were overlain on the surficial geology and geomorphology and are spaced differently according to the general topography so that the more subdued terrain of the glacier foreland was contour spaced at 5 m intervals below 200 m a.s.l. and the higher relief mountain sides at the lateral margins of the glacier at 20 m intervals above 200 m a.s.l. The mountain sides are depicted by the orthophotograph image and have not been mapped in the western and southeastern map margins, as they contain no glacial deposits but comprise instead bedrock outcrops and minor areas of scree and other colluvium. The same approach is taken with the glacier surface, which is represented by the orthophotograph in order to depict ice structures such as crevasses, ogives and debris cover patterns. The map overlay containing the base data was manually digitized on a large format Cal-Comp tablet digitizer using MapData vector digitizing software. The digitized vector files for the base data were converted from MapData format into ArcInfo ‘generate’ format for importing into Adobe Illustrator. The Main Map is at a scale of 1:4470 when printed on an A1 sheet.
3. Morsárjökull and its historical activity
The valley glacier of Morsárjökull is fed by two spectacular icefalls that descend over the 350 m high bedrock cliff that forms the trough head of Morsárdalur. Ice flow over these icefalls is nourished by the Öræfajökull mountain icefield on the summit of the Öræfajökull stratovolcano. Glacier thinning had resulted in the eastern icefall becoming detached from the glacier snout in the mid-1930s to expose the bedrock cliff, but has continued to feed the snout via ice avalanches ever since (). Although the western icefall is still connected to the snout it too has thinned to reveal most of the underlying bedrock cliff. The descent of ice through the two icefalls, either by direct flow or avalanching, creates well-developed ogives or Forbes bands, features that were studied intensively during early glaciological research in the area (CitationIves & King, Citation1954, Citation1955; CitationKing & Ives, Citation1955, Citation1956). The two icefalls flow around a nunatak that is connected to the long exposed part of the bedrock cliff, leading to the formation of two flow units in the valley glacier separated by a medial moraine. Although this medial moraine constitutes an ice-stream interaction type in the scheme of CitationEyles and Rogerson (Citation1978), it is clear from the maps produced by CitationIves and King (Citation1954, Citation1955) and from early ground photographs () that the moraine widened in the middle reaches of the snout before narrowing again immediately down flow. This indicates an intermittent RSF input from the bedrock cliff backwall over time and hence the characteristics of CitationEyles and Rogerson’s (1978) avalanche-type medial moraine. Repeat aerial photographs and observations on the supraglacial changes to rock slope failures indicate that glacier flow transfers supraglacial debris from the base of the icefalls to the snout quickly. Indeed calculations by CitationÞórarinsson (1952) indicated an average surface velocity for the glacier of 130 m per year, which would transfer medial moraine debris from the valley head backwall to the snout in approximately 30 years. This indicates that debris transfer through the valley glacier is efficient. In combination with a coupled glacier-outwash system (CitationBenn et al., Citation2003), this efficient debris throughput is unlikely to lead to the development of large areas of debris-covered, stagnant ice or substantial RSF-related latero-frontal moraine arcs (cf. CitationReznichenko, Davies, & Alexander, Citation2011, Citation2012a, Citation2012b) around the margins of a receding active temperate valley glacier.
Figure 2. Ground photographs of Morsárjökull taken by Jack Ives in 1953: (a) the nunatak and backwall cliffs in the distance and the ogives and medial moraine and their relationship to the twin flow units of the glacier; (b) the ice falls and their relationship to the backwall cliffs and nunatak, showing the narrow connection of the west icefall to the valley glacier and the ice avalanches feeding the eastern ice flow unit.
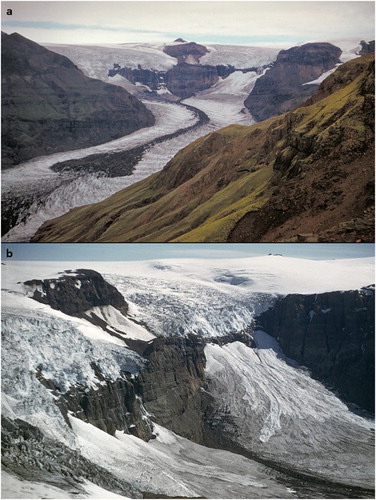
Ongoing observations on an historical rock avalanche deposit confirm the efficacy of this debris transfer process. On 20 March and then the 17 April 2007, the steep bedrock cliffs that overlook the eastern margin of Morsárjökull collapsed due to a huge RSF, sending approximately 4,000,000 m3 of rock debris on to the glacier surface (CitationSæmundsson et al., Citation2011; ). The runout of the debris extended over the eastern flow unit and the medial moraine. Between 2007 and 2011, the outer, down-glacier margin of the rock debris had been transported by glacier flow over a distance of 0.331 km, indicating a glacier surface flow rate of approximately 83 m per year (CitationHermle, Citation2015).
The early history of the marginal fluctuations of Morsárjökull, like other glacier snouts in southern Iceland, indicates a strong coupling with regional climate and a prominent moraine signature reflective of LIA conditions. It likely reached its maximum LIA extent shortly after 1750 (CitationÞórarinsson, 1943) and the outermost moraine arc (Öldur) may date to the late nineteenth century, according to lichenometric dating by CitationChenet, Roussel, Jomelli, and Grancher (Citation2010). Related to that time period, CitationÞoroddsen (Citation1911) identified a dual moraine belt (Öldur) at the glacier margin in 1894 AD, but the ice had by that time receded from the outermost of these moraines. A further prominent moraine belt is thought to mark the glacier terminus in 1904, as demarcated by the first accurate map produced by the Danish Geodetic Survey. Hence, CitationRabot (Citation1906) calculated a 400 m glacier recession from the outermost moraine arc (Öldur) to its position demarcated by the Danish Geodetic survey map in 1904. Snout oscillations since 1931 have been documented by the Icelandic Glaciological Society () and these have been mapped by CitationHermle (Citation2015) and CitationStötter, Hermle, and Sailer (Citation2016; ) who identify relatively uniform and continuous recession rates of between 30 and 40 m per year but punctuated by a phase of ice advance in the early 1970s to late 1980s. More specifically within this overall recession pattern CitationHermle (Citation2015) and CitationStötter et al. (Citation2016) identify five phases of glacier activity: (1) 1932–1956, a period of pronounced retreat of >1 km, with a mean annual value of approximately −43.5 m; (2) 1957–1973, a period of reduced rates of retreat with a mean annual value of approximately −17.2 m; (3) 1974–1989, a period of advance with a mean annual value of around +10.2 m; (4) 1990–1999, a period of predominant stillstand but with mean annual recession of around −7.4 m; and (5) 2000–2013, a period of accelerating glacier melt with a mean annual value of around −37.8 m and a maximum value during the season 2012–2013 of −126 m. For the period prior to those of historical observations, CitationHannesdóttir, Björnsson, Pálsson, Aðalgeirsdóttir, and Guðmundsson (Citation2015) used a modelling approach to propose that the greatest loss in the area of the glacier occurred in the period 1904–1945 during which it lost around 3 km2. The similarity in advance patterns between Morsárjökull and the nearby Öræfajökull outlets of Falljökull and Skaftafellsjökull, as well as the quasi-stationary positions of Öræfajökull-nourished Svínafellsjökull and Kvíárjökull, from the early 1970s to the late 1980s () indicates that these glaciers are responding to the same climatic drivers and have not been decoupled from those climatic drivers by supraglacial debris covers.
Figure 3. Variations since 1931 of the non-surge-type margins of the Vatnajökull South Region glaciers, using data from Veðurstofa Íslands (Icelandic Meteorological Office) and the Icelandic Glaciological Society. Data compilation by Ben Chandler. Note the period of advance for Morsárjökull and the nearby Öræfajökull outlets of Falljökull and Skaftafellsjökull from the early 1970s to the late 1980s.
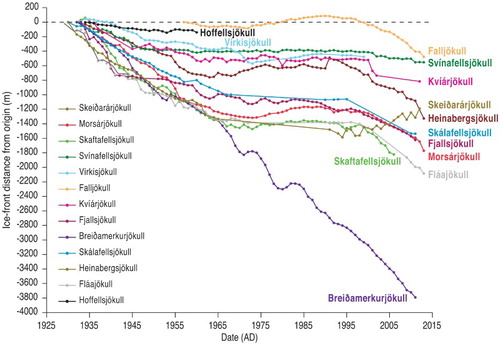
Figure 4. Historical changes of the Morsárjökull snout: (a) DEM showing ice margin position from 1903 to 2011 and the position of the rock avalanche debris in 2007, 2011 and 2017 (modified from CitationStötter et al., Citation2016); (b) longitudinal profile of the glacier and its underlying topography, showing ice thickness and snout margin through time. Also demarcated in light blue is the average ELA derived from MODIS satellite imagery (modified from CitationHannesdóttir et al., Citation2015).
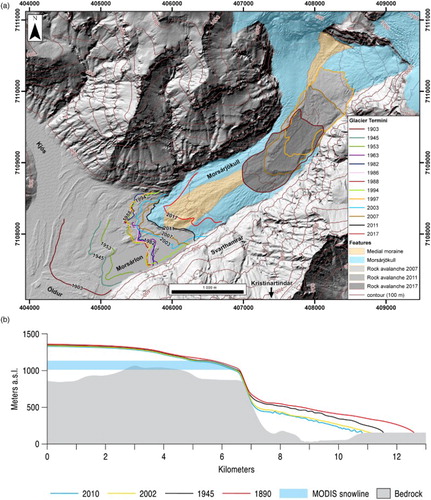
Like most of the south-coast outlet glaciers, Morsárjökull's recent accelerated recession has begun to uncover an overdeepening in which a proglacial lake, Morsárlon, has developed and initiated snout calving (cf. CitationBennett et al., Citation2010; CitationEvans & Orton, Citation2015; CitationEvans & Twigg, Citation2002; CitationEvans, Ewertowski, & Orton, Citation2016; CitationEvans et al., Citation2009; CitationHannesdóttir et al., Citation2015; CitationHowarth & Price, Citation1969; CitationPhillips, Finlayson, & Jones, Citation2013, Citation2014; CitationÞórarinsson, 1939). The name of Morsárlon has been proposed by CitationStötter et al. (Citation2016), who suggest that it likely developed in 1940–1941 based on their assessment of historical archives; it is visible on the first aerial images taken in 1945. The bedrock topography (CitationHannesdóttir et al., Citation2015; CitationMagnússon, Björnsson, & Pálsson, Citation2007; CitationMagnússon, Pálsson, Björnsson, & Guðmundsson, Citation2012) indicates that snout recession will likely accelerate in response to increased calving activity as the glacier uncovers its ca. 60 m deep overdeepening in the next decade ((b)).
4. Glacial geomorphology and surficial geology of Morsárjökull
In addition to bedrock, seven surficial geology map units are identified on and around the glacier foreland on the 1:4470 scale map (Main Map), all of which are colour-coded following the protocol established for previous Icelandic glacier foreland maps (CitationBennett et al., Citation2010; CitationEvans & Orton, Citation2015; CitationEvans & Twigg, Citation2002; CitationEvans et al., Citation2006, Citation2007, Citation2009, Citation2017; CitationHowarth & Welch, Citation1969a, Citation1969b). The mountain sides to the west and southeast of the foreland contain no glacial deposits and so the bedrock outcrops and minor areas of scree and other colluvium in these areas are depicted by the orthophotograph image; small areas of moraine and some lateral meltwater channels have been mapped above the more extensive glacigenic and paraglacial and slope deposits on the southeastern mountain slopes. More comprehensive mapping was undertaken on the mountain slopes to the north of the foreland where lateral moraines and tills are interspersed with glacifluvial channel fills, paraglacially modified deposits and alluvial fans in dissected bedrock terrain. Areas of older, residual glacigenic deposits (pre-LIA till) and weathered bedrock too small to differentiate at this scale are mapped as residuum. Glacigenic deposits on the glacier foreland are associated with specific landforms and geomorphic process-form regimes, the landforms being depicted using the symbology also established in previous Icelandic glacier maps. These are predominantly: (a) till and moraines dating to and since the LIA; and (b) glacifluvial outwash. Minor areas of glacilacustrine and push moraine ridges developed in ice-contact glacifluvial deposits are also identified. Unlike previous maps of the Icelandic glacier forelands, the map of the Morsárjökull foreland includes individual boulders large enough to be visible on the aerial photography, because they are present in large numbers and are therefore significant in terms of debris transport in a glaciated valley setting.
4.1. Till and moraine and associated features dating to the LIA and younger
The till and moraines surficial geology map unit on the Morsárjökull foreland is not unlike that of other recently deglaciated forelands in southern Iceland in that it includes locally densely spaced push moraines and associated flutings. Also common are the crenulated to sawtooth planforms of the push moraines, characteristic of construction by snouts indented by radial crevasses or pecten (CitationChandler, Evans, & Roberts, Citation2016; CitationChandler, Evans, Roberts, Ewertowski, & Clayton, Citation2016; CitationEvans et al. Citation2016, Citation2017; CitationPrice, Citation1970; CitationSharp, Citation1984). However, a further important characteristic of the moraines of the Morsárjökull foreland is the large volume of individual moraine ridges, especially on the northern and southern or valley-side margins of the foreland. Although composite moraines comprising partially overprinted push ridges on other south coast active temperate forelands may attain heights of 15 m (e.g. CitationEvans & Hiemstra, Citation2005; CitationEvans et al., 2017), individual moraine ridges rarely exceed 2 m in height (e.g. CitationBoulton, 1986; CitationChandler, Evans, & Roberts, Citation2016; CitationChandler, Evans, Roberts, Ewertowski, et al., 2016; CitationEvans et al., Citation2016, Citation2017; CitationSharp, Citation1984); in contrast individual moraine ridges on the north and south sides of the Morsárjökull foreland are commonly ≥5 m high, with the largest complex, Öldur, attaining a relief of 12 m and thereby indicating possible within-valley lateral moraine asymmetry (CitationBenn, Citation1989). Their spatial variability is also well illustrated by the densely spaced moraine ridges pertaining to the 1970s–1990s period of quasi-stationary snout oscillations, wherein the moraines on the north foreland are more voluminous than those of the central foreland. On the central foreland significant areas of strongly fluted glacier bed are visible between the narrow and low amplitude moraines. When viewed over the whole foreland (Main Map) this contrast in moraine ridge size indicates greater volumes of debris delivery to the snout along its lateral, especially south, margins and, in contrast, a relatively impoverished debris supply to moraine construction along the central valley axis. Moraine spacing can also be seen to vary with age, as illustrated by the better-preserved moraine record along the north foreland, and this reflects the rates of ice recession recognized by CitationHannesdóttir et al. (Citation2015) and CitationStötter et al. (Citation2016). Two zones reflect glacier margin stability in their dense moraine spacing (cf. Main Map and (a)): (a) an outer zone dating from around 1890 to 1903; and (b) an inner zone dating from the late 1970s to the late 1990s. Between these two zones lies an intermediate zone of more widely spaced and lower amplitude moraines that documents a relatively faster rate of ice-marginal recession from 1903 to the 1970s.
As highlighted above, flutings (or flutes) are typically tens of metres long and are located on those areas of the foreland where moraine spacing is wide enough to expose the former subglacial bed, specifically in the central axis of the foreland and in the intermediate age zone dating to 1903–1970s. Typical of flutings on southern Iceland glacier forelands, the planform of the Morsárjökull flutings reflects arrangements in arcuate sets between push moraines, each set slightly offset from one another and thereby recording the slight sub-marginal ice flow directional changes from year to year. Some individual flutings initiate at stoss clasts (cf. CitationBenn, 1994; CitationBoulton, Citation1976) but many appear to be only draped by large isolated boulders and are hence unrelated to them. At a few locations the fluting pattern appears to comprise large, drumlin-like bedforms ((b); cf. CitationKrüger & Thomsen, Citation1984; CitationBoulton, 1987; CitationJohnson et al., Citation2010; CitationJónsson, Schomacker, Benediktsson, Ingólfsson, & Johnson, Citation2014).
Figure 5. Glacial landforms and sediments of the Morsárjökull foreland: (a) large sawtooth moraine ridges (SM) and intervening glacifluvial outwash corridors (OC) produced where proglacial outwash was confined between moraine arcs on the northern foreland. This area of moraines is typical of the outer zone of slow snout recession dating to the period 1890–1903; (b) heavily fluted terrain (F) with relatively widely spaced push moraines (P) located in the central axis of the foreland and representative of relatively low debris provision and slow snout recession and readvances in the period 1970s–1990s. The large moraine complex in the centre of the image dates to around 1988 and was constructed around the time between the 1974 and 1989 advance period and 1990–1999 stillstand period; (c) heavily dissected and reworked moraines on the outer foreland, showing residual ‘islands’ of moraines isolated by relict and active braided outwash tracts. Note also the isolated large boulders let down onto the outwash after moraines have been completely reworked; (d) kame terraces (KT) and incised outwash developed on the proximal slopes of the immediately post-1903 sawtooth moraine complex (1903 SMC) on the south side of the foreland. Also clearly visible are isolated large boulders let down onto the outwash after moraine reworking.
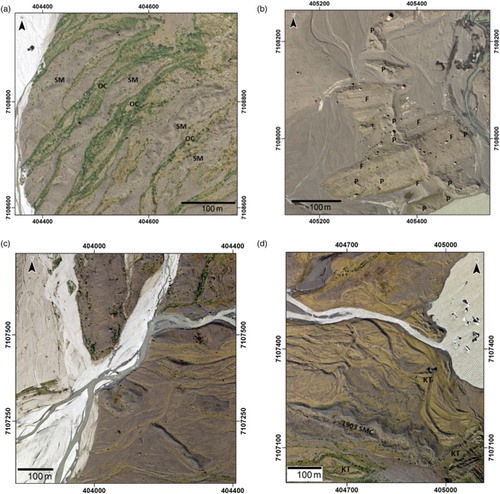
Prominent across the foreland are numerous isolated boulders, which lie on moraine ridges and have been let down onto outwash surfaces by meltwater reworking of till and moraine assemblages. They are predominantly angular and lack the wear features typical of subglacial traction zone transport, but some are partially embedded in till and moraine surfaces with their upper faces abraded and striated to form subglacially modified facets. These characteristics attest to predominantly passive or supraglacial to an englacial transport of the abundant supplied of extraglacial debris but in some cases also record contact with the glacier bed, the latter appearing to take place in sub-marginal settings based upon boulder emplacement within moraine crests ((c), ). Together with sawtooth moraine planforms, this reflects the likely construction of the Morsárjökull moraines through the combined processes of pushing and dumping. Between moraines the passive dumping of supraglacial debris, such as that visible in the medial moraine of the glacier, amounts to only the draping of boulders onto the fluted subglacial surface.
Figure 6. Stratigraphic exposure in the outermost arc of the Morsárjökull frontal moraines showing a vertical sequence comprising poorly sorted, ice-proximal outwash fan gravels at the base, overlain by compact and fissile structured, matrix-supported diamicton (subglacial till) and capped by crudely stratified to massive, clast-supported diamictons with distorted pockets of openwork gravels and gravelly sands (gravity mass flow deposits) containing large boulders. One metre high backpack is lying at the base of the section.
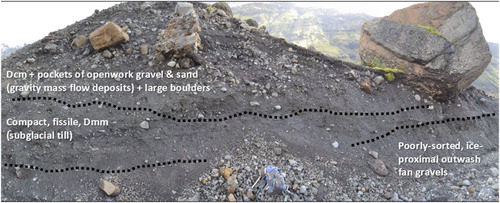
A small number of stratigraphic exposures in the Morsárjökull moraines occur where meltwater stream erosion has excavated their margins (). Sediments are typically arranged in a vertical sequence comprising, from bottom to top: (a) poorly sorted, ice-proximal outwash fan gravels; (b) compact and fissile structured, matrix-supported diamictons typical of Icelandic subglacial tills (CitationBenn, 1995; CitationEvans, Citation2000; CitationEvans, Roberts, & Evans, Citation2016; CitationKrüger, Citation1979); and (c) crudely stratified to massive, clast-supported diamictons with distorted pockets of openwork gravels and gravelly sands typical of gravity mass flows and localized meltwater reworking and often containing large boulders. The occurrence of these sediment sequences within sawtooth moraine ridges documents both supraglacial and sub-marginal processes typical of dump and push moraines (cf. CitationBoulton & Eyles, Citation1979; CitationChandler, Evans, & Roberts, Citation2016; CitationChandler, Evans, Roberts, Ewertowski, et al., 2016; CitationEyles, Citation1979; CitationSharp, Citation1984).
4.2. Glacifluvial deposits including eskers
Extensive spreads and thicknesses of coarse-grained glacifluvial deposits are typical of the Icelandic south coast glacier lobes, and the foreland of Morsárjökull is no exception, although the valley setting gives rise to a laterally restricted depositional environment or valley sandur (valley trains) in contrast to the more common outwash/sandur fans (e.g. CitationChurch, Citation1972; CitationChurch & Gilbert, Citation1975; CitationWilliams & Rust, Citation1969; CitationZielinksi & van Loon, Citation2003). Hence the proglacial outwash that has accumulated beyond the LIA maximum moraines comprises a narrow valley sandur with highly active and frequently migrating channels with minimal terrace preservation. It is fed not only by meltwater from Morsárjökull but also by nival melt from the non-glacierized mountain catchments in the Kjós Valley, where the river Kjósarlækur has formed a braided stream network which has reworked parts of the Morsárjökull outer moraine arcs. Within the LIA moraine sequences glacifluvial deposits are arranged in a series of linear sandar developed between the larger moraine ridges, documenting the localized topographic confinement of proglacial meltwater. Only in the central axis of the foreland, where moraines are more subdued, do these corridors widen to form spatially restricted fans. The greatest degree of moraine reworking by meltwater is evident also in this central axis of the foreland where terraced outwash contains only isolated ‘islands’ of remnant moraine ridges ((c)). Towards the left lateral side of the foreland these outwash features grade into kame terraces ((d)) along the south shore of Morsárlon where proglacial drainage was deflected to the centre of the valley by the more substantial latero-frontal moraine arcs in this area.
Kettled outwash, indicative of glacifluvial aggradation over shallow snout ice, is apparent only along the narrow strip of sandur deposited in the last 20 years. In places snout oscillations ( and ) have displaced this material to form a spatially restricted map unit classified as ‘push moraine ridges developed in ice-contact glacifluvial deposits’. The largely restricted occurrence of kettled outwash in this area is likely a reflection of the recent early stages of recession from the adverse slope of the overdeepening and hence, like other south coast outlet glaciers, will probably form an increasingly important component of the landsystem in future (c.f. CitationBennett et al., Citation2010; CitationBennett & Evans, Citation2012; CitationBradwell, Sigurðsson, & Everest, Citation2013).
5. The debris-charged glaciated valley landsystem at Morsárjökull
Morsárjökull is confined by a deep valley in which both the glacier snout and its proglacial outwash are constrained, and copious extraglacial debris is derived from surrounding rock slopes. Two dominant glacial geomorphic process-form regimes can be recognized in the main landform-sediment assemblages. Firstly, till and moraines dating to the LIA and the subsequent historical period of snout recession record the deposition of subglacially deformed/fluted till and the pulsed delivery of supraglacial debris, which combine to feed glacier sub-marginal and marginal processes and form dump and push moraines, often with sawtooth planforms due to radial crevasses and ice-marginal pecten. Secondly, glacifluvial outwash is related to the building of valley sandur or linear sandur tracts between the larger moraine ridges, with local reworking into push moraine ridges, typical of active temperate snout recession. These characteristics have resulted in the development of a foreland that serves as an exemplar for the debris-charged glaciated valley landsystem in the area. In detail, the uneven distribution of moraine volume over the foreland appears to reflect greater debris provision to the lateral margins of the snout over time, which contrasts with the central axis of the foreland and its larger areas of fluted subglacial bed and more modest moraine sizes. This is typical of valley glacier settings where a constant supply of extraglacial debris is derived from precipitous bounding rockwalls and contributes to lateral moraine construction. Additionally, within-valley lateral moraine asymmetry (CitationBenn, Citation1989) arises in situations where more extensive free faces exist on one side of the glacier catchment. This is well demonstrated by the large moraine complex of Öldur, which has been developed on the southern foreland as a product of relatively greater debris supply from the heavily dissected bedrock slopes of Kristinartindur on the south margin of the glacier; a modern analogue for this process was demonstrated by the 2007 rock avalanche onto the glacier surface (CitationSæmundsson et al., Citation2011; ).
Although Morsárjökull has a debris-charged snout, it has not produced large areas of supraglacially derived hummocky (stagnation) topography, constructing instead latero-frontal moraines that are in places separated by fluted subglacial surfaces. This landsystem signature is typical of the ‘uncovered alpine glacier’ identified by CitationBenn et al. (Citation2003) in their continuum of glaciated valley landsystem types. Such landsystems reveal combined signatures of strong glacier-climate coupling, or active temperate activity, coupled with the localized and sporadic retardation of ablation and the concomitant development of boulder rubble drapes that can be related to rock avalanche-type medial moraines (CitationEyles & Rogerson, Citation1978). Additionally, this landsystem signature is classified as one that is ‘coupled’ in that there is efficient sediment transfer from the glacial to the fluvial zones and hence moraine development is relatively limited and large amounts of sediment pass into and through the proglacial zone. The supply of extraglacial debris via rockfall and RSF is therefore transferred through the glacier efficiently and most sediment leaves the glacier as glacifluvial load; the dynamic activity of migrating proglacial meltwater streams effectively removes large parts of any moraines that are formed at the snout, so that over longer timescales the landsystem signature is dominated by outwash heads or aggrading sandar (CitationKirkbride, Citation2000; CitationBenn et al., Citation2003; e.g. Heinabergsjökull/Skalafellsjökull, CitationEvans & Orton, Citation2015). Although the latero-frontal moraines on the Morsárjökull foreland are relatively large compared to those of other Icelandic south coast glaciers, their localized substantial reworking by proglacial meltwater and conversion into terraced and incised outwash with remnant boulders is indicative of the operation of an uncovered and coupled glaciated valley landsystem.
6. Conclusion
A 1:4470 map of the Morsárjökull foreland, southern Iceland reveals the details of the spatial distribution of post LIA glacial sediment–landform associations, which relate specifically to the influence of supraglacial debris transport on the typical active temperate glacial landsystem signature for the south Vatnajökull outlet lobes. Two geomorphic process-form regimes are identified based upon the landform-sediment assemblages: (1) till and moraines dating to and since the LIA; and (2) glacifluvial outwash, locally developed into push moraine ridges during recent snout recession. More specifically, the foreland is an exemplar for the debris-charged glaciated valley landsystem, with the uneven distribution of moraine volume and localized appearance of a fluted subglacial bed over the foreland reflecting greater debris provision to the lateral margins of the snout over time as well as within-valley lateral moraine asymmetry. This patchy coverage of supraglacially derived hummocky (stagnation) topography and its association with well-developed latero-frontal moraines locally separated by fluted subglacial surfaces indicates a landsystem signature typical of ‘uncovered alpine glaciers’. Such glaciers are characterized by strong glacier-climate coupling and only localized retardation of ablation by the development of boulder rubble drapes related to sporadic rock avalanche-type medial moraines. Strong coupling of the glacial system results in efficient sediment transfer from the glacial to the fluvial zones and therefore relatively limited moraine construction, with areas of more substantial latero-frontal moraine documenting phases of RSF onto the snout and the passage of the debris to the ice margin.
Software
Aerial photographs were orthorectified and mosaicked using Agisoft Photoscan Professional Edition. Orthophotograph processing and contour generation were both performed in ESRI ArcGIS. The map overlay containing the base data was manually digitized on a large format Cal-Comp tablet digitizer using MapData vector digitizing software. The digitized vector files for the base data were converted from MapData format into ArcInfo ‘generate’ format for importing into Adobe Illustrator.
Morsajokull_map.compressed.pdf
Download PDF (6.8 MB)Acknowledgements
Aerial photographs taken in 2007 were provided by the NERC Airborne Remote Sensing Facility, UK. A research permit to undertake fieldwork at Morsárjökull was provided by Regina Hreinsdóttir on behalf of Skaftafell National Park. Ben Chandler compiled Figure 2 based upon data from Veðurstofa Íslands (Icelandic Meteorological Office) and the Icelandic Glaciological Society.
Disclosure statement
No potential conflict of interest was reported by the authors.
ORCID
Marek Ewertowski http://orcid.org/0000-0002-0422-2327
Additional information
Funding
References
- Benn, D. I. (1989). Debris transport by Loch Lomond readvance glaciers in northern Scotland – basin form and the within-valley asymmetry of lateral moraines. Journal of Quaternary Science, 4, 243–254. doi: 10.1002/jqs.3390040305
- Benn, D. I. (1994). Fluted moraine formation and till genesis below a temperate glacier: Slettmarkbreen, Jotunheimen, Norway. Sedimentology, 41, 279–292. doi: 10.1111/j.1365-3091.1994.tb01406.x
- Benn, D. I. (1995). Fabric signature of subglacial till deformation, Breiðamerkurjokull, Iceland. Sedimentology, 42, 735–747. doi: 10.1111/j.1365-3091.1995.tb00406.x
- Benn, D. I., Kirkbride, M. P., Owen, L. A., & Brazier, V. (2003). Glaciated valley landsystems. In D. J. A. Evans (Ed.), Glacial landsystems (pp. 372–406). London: Arnold.
- Bennett, G. L., & Evans, D. J. A. (2012). Glacier retreat and landform production on an overdeepened glacier foreland: The debris-charged glacial landsystem at Kvíárjökull, Iceland. Earth Surface Processes and Landforms, 37, 1584–1602. doi: 10.1002/esp.3259
- Bennett, G. L., Evans, D. J. A., Carbonneau, P., & Twigg, D. R. (2010). Evolution of a debris-charged glacier landsystem, Kvíárjökull, Iceland. Journal of Maps, 6, 40–67. doi: 10.4113/jom.2010.1114
- Boulton, G. S. (1976). The origin of glacially fluted surfaces – observations and theory. Journal of Glaciology, 17, 287–309. doi: 10.1017/S0022143000013605
- Boulton, G. S. (1986). Push moraines and glacier contact fans in marine and terrestrial environments. Sedimentology, 33, 677–698. doi: 10.1111/j.1365-3091.1986.tb01969.x
- Boulton, G. S. (1987). A theory of drumlin formation by subglacial sediment deformation. In J. Menzies & J. Rose (Eds.), Drumlin symposium (pp. 25–80). Rotterdam: Balkema.
- Boulton, G. S., & Eyles, N. (1979). Sedimentation by valley glaciers: A model and genetic classification. In C. Schluchter (Ed.), Moraines and varves (pp. 11–23). Rotterdam: Balkema.
- Bradwell, T., Sigurðsson, O., & Everest, J. (2013). Recent, very rapid retreat of a temperate glacier in SE Iceland. Boreas, 42, 959–973.
- Chandler, B. M. P., Evans, D. J. A., & Roberts, D. H. (2016). Characteristics of recessional moraines at a temperate glacier in SE Iceland: Insights into patterns, rates and drivers of glacier retreat. Quaternary Science Reviews, 135, 171–205. doi: 10.1016/j.quascirev.2016.01.025
- Chandler, B. M. P., Evans, D. J. A., Roberts, D. H., Ewertowski, M., & Clayton, A. I. (2016). Glacial geomorphology of the Skálafellsjökull foreland, Iceland: A case study of ‘annual’ moraines. Journal of Maps, 12, 905–916.
- Chenet, M., Roussel, E., Jomelli, V., & Grancher, D. (2010). Asynchronous little ice Age glacial maximum extent in southeast Iceland. Geomorphology, 114, 253–260. doi: 10.1016/j.geomorph.2009.07.012
- Church, M. (1972). Baffin island sandurs – a study of Arctic fluvial processes. Ottawa: Geological Survey of Canada. Bulletin 216.
- Church, M., & Gilbert, R. (1975). Proglacial fluvial and lacustrine sediments. In A. V. Jopling & B. C. McDonald (Eds.), Glaciofluvial and glaciolacustrine sedimentation (pp. 22–100). Tulsa: SEPM Special Publication 23.
- Evans, D. J. A. (2000). A gravel outwash/deformation till continuum, skalafellsjokull, Iceland. Geografiska Annaler, Series A: Physical Geography, 82, 499–512. doi: 10.1111/j.0435-3676.2000.00137.x
- Evans, D. J. A., Ewertowski, M., & Orton, C. (2016). Fláajökull (north lobe), Iceland: Active temperate piedmont lobe glacial landsystem. Journal of Maps, 12, 777–789.
- Evans, D. J. A., Ewertowski, M., & Orton, C. (2017). Skaftafellsjokull, Iceland: Glacial geomorphology recording glacier recession since the little ice age. Journal of Maps, 13, 358–368. doi: 10.1080/17445647.2017.1310676
- Evans, D. J. A., & Hiemstra, J. F. (2005). Till deposition by glacier submarginal, incremental thickening. Earth Surface Processes and Landforms, 30, 1633–1662. doi: 10.1002/esp.1224
- Evans, D. J. A., & Orton, C. (2015). Heinabergsjökull and Skálafellsjökull, Iceland: Active temperate piedmont lobe and outwash head glacial landsystem. Journal of Maps, 11, 415–431. doi: 10.1080/17445647.2014.919617
- Evans, D. J. A., Roberts, D. H., & Evans, S. C. (2016). Multiple subglacial till deposition: A modern exemplar for Quaternary palaeoglaciology. Quaternary Science Reviews, 145, 1–21. doi: 10.1016/j.quascirev.2016.05.029
- Evans, D. J. A., Shand, M., & Petrie, G. (2009). Maps of the snout and proglacial landforms of Fjallsjökull, Iceland (1945, 1965, 1998). Scottish Geographical Journal, 125, 304–320. doi: 10.1080/14702540903364310
- Evans, D. J. A., & Twigg, D. R. (2002). The active temperate glacial landsystem: A model based on Breiðamerkurjökull and Fjallsjökull, Iceland. Quaternary Science Reviews, 21, 2143–2177. doi: 10.1016/S0277-3791(02)00019-7
- Evans, D. J. A., Twigg, D. R., Rea, B. R., & Shand, M. (2007). Surficial geology and geomorphology of the Brúarjökull surging glacier landsystem. Journal of Maps, 3, 349–367. doi: 10.1080/jom.2007.9710850
- Evans, D. J. A., Twigg, D. R., & Shand, M. (2006). Surficial geology and geomorphology of the Þorisjokull plateau icefield, west-central Iceland. Journal of Maps, 2, 17–29. doi: 10.4113/jom.2006.52
- Eyles, N. (1979). Facies of supraglacial sedimentation on Icelandic and alpine temperate glaciers. Canadian Journal of Earth Sciences, 16, 1341–1361. doi: 10.1139/e79-121
- Eyles, N. (1983a). The glaciated valley landsystem. In N. Eyles (Ed.), Glacial geology: An Introduction for engineers and earth scientists (pp. 91–110). Oxford: Pergamon.
- Eyles, N. (1983b). Modern Icelandic glaciers as depositional models for ‘hummocky moraine’ in the Scottish highlands. In E. B. Evenson, C. Schluchter, & J. Rabassa (Eds.), Tills and related deposits: Genesis, petrology, stratigraphy (pp. 47–60). Rotterdam: Balkema.
- Eyles, N., & Rogerson, R. J. (1978). A framework for the investigation of medial moraine formation: Austerdalsbreen, Norway, and Berendon glacier, British Columbia, Canada. Journal of Glaciology, 20, 99–113. doi: 10.1017/S0022143000021249
- Hannesdóttir, H., Björnsson, H., Pálsson, F., Aðalgeirsdóttir, G., & Guðmundsson, S. (2015). Area, volume and mass changes of southeast Vatnajökull ice cap, Iceland, from the little ice age maximum in the late 19th century to 2010. Cryosphere, 9, 565–585. doi: 10.5194/tc-9-565-2015
- Hermle, D. (2015). Impact of climate change and the 2007 rock avalanche on the glacial history of Morsárjökull, southeast Iceland. Analysis of multi-temporal remote sensing data (1982–2014) (Unpublished MSc thesis). University of Innsbruck, 184 pp.
- Howarth, P. J., & Price, R. J. (1969). The proglacial lakes of Breiðamerkurjökull and Fjallsjökull, Iceland. Geographical Journal, 135, 573–581. doi: 10.2307/1795105
- Howarth, P. J., & Welch, R. (1969a). Breiðamerkurjökull, South-east Iceland, August 1945. 1:30,000 scale map. University of Glasgow.
- Howarth, P. J., & Welch, R. (1969b). Breiðamerkurjökull, South-east Iceland, August 1965. 1:30,000 scale map. University of Glasgow.
- Ives, J. D., & King, C. A. M. (1954). Glaciological observations on Morsárjökull, SW Vatnajökull. Pt. I: The ogive banding. Journal of Glaciology, 2, 423–428. doi: 10.3189/002214354793702443
- Ives, J. D., & King, C. A. M. (1955). Glaciological observations on Morsárjökull, SW Vatnajökull. Pt. II: Regime of the glacier, present and past. Journal of Glaciology, 2, 477–482. doi: 10.3189/002214355793702325
- Johnson, M. D., Schomacker, A., Benediktsson, Í. Ó., Geiger, A. J., Ferguson, A., & Ingólfsson, Ó. (2010). Active drumlin field revealed at the margin of Múlajökull, Iceland: A surge-type glacier. Geology, 38, 943–946. doi: 10.1130/G31371.1
- Jónsson, S. A., Schomacker, A., Benediktsson, Í. Ó., Ingólfsson, Ó., & Johnson, M. D. (2014). The drumlin field and the geomorphology of the Múlajökull surge-type glacier, central Iceland. Geomorphology, 207, 213–220. doi: 10.1016/j.geomorph.2013.11.007
- King, C. A. M., & Ives, J. D. (1955). Glaciological observations on some of the outlet glaciers of south-west Vatnajökull. Pt. I: Glacier regime. Journal of Glaciology, 2, 563–569. doi: 10.3189/002214355793702109
- King, C. A. M., & Ives, J. D. (1956). Glaciological observations on some of the outlet glaciers of south-west Vatnajökull, Iceland, 1954. Pt. II: Ogives. Journal of Glaciology, 2, 646–651. doi: 10.3189/S0022143000033098
- Kirkbride, M. P. (2000). Ice marginal geomorphology and Holocene expansion of debris-covered Tasman glacier, New Zealand. In M. Nakawo, C. Raymond, & A. Fountain (Eds.), Debris-covered glaciers (pp. 211–217). Wallingford: IAHS Publication 264.
- Krüger, J. (1979). Structures and textures in till indicating subglacial deposition. Boreas, 8, 323–340. doi: 10.1111/j.1502-3885.1979.tb00816.x
- Krüger, J., & Thomsen, H. H. (1984). Morphology, stratigraphy and genesis of small drumlins in front of the glacier Myrdalsjokull, south Iceland. Journal of Glaciology, 30, 94–105. doi: 10.1017/S0022143000008534
- Magnússon, E., Björnsson, H., & Pálsson, F. (2007). Landslag í grennd Kvískerja í fortíð og framtíð. Niðurstöður íssjármælinga á Kvíár-, Hrútár- og Fjallsjökli. Jökull, 57, 83–89.
- Magnússon, E., Pálsson, F., Björnsson, H., & Guðmundsson, S. (2012). Removing the ice cap of Öræfajökull central volcano, SE-Iceland. Mapping and interpretation of bedrock topography, ice volumes, subglacial troughs and implications for hazards assessments. Jökull, 62, 132–150.
- Phillips, E., Finlayson, A., Bradwell, T., Everest, J., & Jones, L. (2014). Structural evolution triggers a dynamic reduction in active glacier length during rapid retreat: Evidence from Falljökull, SE Iceland. Journal of Geophysical Research – Earth Surface, 119, 2194–2000. doi: 10.1002/2014JF003165
- Phillips, E., Finlayson, A., & Jones, L. (2013). Fracturing, block faulting, and moulin development associated with progressive collapse and retreat of a maritime glacier: Falljökull, SE Iceland. Journal of Geophysical Research – Earth Surface, 118, 1–17.
- Price, R. J. (1970). Moraines at Fjallsjökull, Iceland. Arctic and Alpine Research, 2, 27–42. doi: 10.2307/1550139
- Rabot, C. (1906). Les variations des glaciers de l‘Islande meridionale des 1893–1894 à 1903–1904 d'après la nouvelle carte d'Islande. Zeitschrift für Gletscherkunde, 1906, 132–138.
- Reznichenko, N. V., Davies, T. R., & Alexander, D. J. (2011). Effects of rock avalanches on glacier behavior and moraine formation. Geomorphology, 132, 327–338. doi: 10.1016/j.geomorph.2011.05.019
- Reznichenko, N. V., Davies, T. R., Shulmeister, J. P., & Larsen, S. H. (2012a). A new technique for identifying rock avalanche-sourced sediment in moraines and some palaeoclimatic implications. Geology, 40, 319–322. doi: 10.1130/G32684.1
- Reznichenko, N. V., Davies, T. R., Shulmeister, J. P., & Winkler, S. (2012b). Influence of rock avalanches upon the formation of moraines and their subsequent palaeoclimatic interpretation: A critical appraisal. Zeitschrift für Geomorphologie, 56, 37–54. doi: 10.1127/0372-8854/2012/S-00079
- Sæmundsson, Þ., Sigurðsson, I. A., Pétursson, G. H., Jónsson, H. P., Decaulne, A., Roberts, M. J., & Jensen, E. H. (2011). Bergfóðið sem féll á Morsárjökull 2007 Mar 22. Náttúrufræðingurinn, 81, 131–141.
- Sharp, M. J. (1984). Annual moraine ridges at Skalafellsjökull, southeast Iceland. Journal of Glaciology, 30, 82–93. doi: 10.1017/S0022143000008522
- Stötter, J., Hermle, D., & Sailer, R. (2016). Morsárlon – a lake as young as jack ives? Past, present and future development of a proglacial lake in southeast Iceland. In K. Mainali & S. Sicroff (Eds.), Jack D. Ives, Montologist – Festschrift for a Mountain Advocate (pp. 245–266). Lalitpur: Himalayan Association for the Advancement of Science, Font Press.
- Þoroddsen, Þ. (1911). Lýsing Íslands II. Hið íslenska bókmenntafélag, Kaupmannahöfn.
- Þ órarinsson, S. (1939). The ice-dammed lakes of Iceland with particular reference to their values as indicators of glacier oscillations. Geografiska Annaler, 21, 216–242. doi: 10.1080/20014422.1939.11880679
- Þ órarinsson, S. (1943). Vatnajökull: Scientific results of the Swedish-Icelandic investigations 1936-37-38. Chapter 11. Oscillations of the Icelandic glaciers in the last 250 years. Geografiska Annaler, 25, 1–54.
- Þ órarinsson, S. (1952). Svigdur á morsárjökli. Jökull, 2, 22–25.
- Williams, P. F., & Rust, B. R. (1969). The sedimentology of a braided river. Journal of Sedimentary Petrology, 39, 649–679. doi: 10.1306/74D71EE2-2B21-11D7-8648000102C1865D
- Zielinksi, T., & van Loon, A. J. (2003). Pleistocene sandur deposits represent braidplains not alluvial fans. Boreas, 32, 590–611. doi: 10.1080/03009480310004170