ABSTRACT
The study describes the hydrogeological setting of the Pearl River Delta, a sub-tropical area of southern China encompassing the metropolises of Guangzhou, Shenzhen, Hong Kong and Macau. In the last 40 years, a booming economy and a population of about 60 million has increased water demand satisfied by a huge system of dams and reservoirs. Aquifers in the studied area are underutilized and only a few recent studies have addressed hydrogeological characterization at a local scale. Understanding groundwater dynamics of the Pearl River Delta is important for developing additional water supplies, understanding and mitigating groundwater pollution, and for implementing ‘Sponge City' concepts. Via a collection of data from literature and field surveys, the hydrogeological setting of the area is synthetized and represented through thematic maps, cross sections and a hydro-stratigraphic column. Hydrogeological conceptual models are developed that describe the groundwater dynamics in urban and rural areas within the Pearl River Delta.
1. Introduction
The Pearl River Delta is a sub-tropical area located alongside the South China Sea where a booming economy has developed in the last 40 years. The area encompasses numerous cities and megacities with Guangzhou located at the center of the fluvial delta and Shenzhen, Hong Kong and Macau along the southern edge of the estuary (). Urbanization is persistent and continuous throughout the coastal areas and the main valleys. As in other tropical megacities, the area suffers from floods and urban waterlogging during the wet seasons and water shortages during the dry season. Numerous reservoirs and dams on the East River, West River and minor streams collect the abundant rainfall in the area (1650–2250 mm/year) (Main Map).
Figure 1. Satellite image (CNES-AIRBUS 2017) of the Pearl River Delta, Guangdong Province (southern China). White circles indicate megacities, cyan lines main rivers, cyan arrows indicate river flow direction.
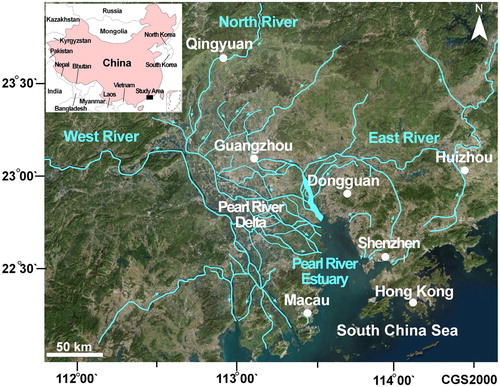
Despite the economic importance, hydrogeological literature about Pearl River Delta is sparse. CitationGGS (1979, Citation1981) provided the first overview with two sets of hydrogeological maps scale 1:200,000. CitationGBGP (1980) investigated the subsoil characteristics with a collection of boreholes stratigraphies and pumping tests. CitationZhong et al. (2009) investigated the Late Quaternary Pearl River Delta stratigraphy and CitationTang et al. (2011) provided information on Quaternary tectonics. Urbanization and industrialization gave rise to several problems about domestic sewage management (CitationLu et al., 2008), slope stability (CitationJiao et al., 2006) and tunneling (CitationZhang et al. 2018). CitationHuang et al. (2011) studied the distribution of arsenic in surface water and shallow aquifers of Pearl River Delta, observing values twenty to one hundred times higher than the background value. CitationWang et al. (2017) focus on submarine groundwater discharge via radon isotopes. CitationHuang et al. (2018) and CitationZhang et al. (2019) analyzed the groundwater chemistry of Pearl River Delta finding groundwater contamination from domestic sewage and industrial wastewater. CitationLancia et al. (2019a) points out the complex geological setting of Shenzhen and compiled an average groundwater budget.
Further understanding of the groundwater dynamics could efficaciously assist in the development of groundwater resources, in understanding and mitigating groundwater pollution and in the application of the Sponge City concept to urban aquifers (CitationLancia et al., 2020). Thus, this study provides a comprehensive hydrogeological understanding of the Pearl River Delta at the regional scale via cartographic representation (Main Map); and develops hydrogeological conceptual models that describe groundwater dynamics in rural and urban areas in the Pearl River Delta.
2. Methods
The map (Main Map) was developed via a collection and organization of hydrogeological and environmental data through a GIS database, scale 1:400,000. Elevation is represented with the Guangdong province DEM (Digital Elevation Model) with a resolution of 90 m (ASTER DEM V003), displayed as a shaded relief and grouped in classes to enhance the basin morphology (Main Map). Rainfall dataset is a combination of synthetic data from remote sensing and pluviometric stations (CitationChen et al., 2011). The dataset was interpolated through an ordinary kriging interpolation method (output cell size 0.01 degree; 12 number of points) and contoured with an interval of 50 mm/year (Main Map). Geological and hydrogeological data are from maps at various scale (CitationGGS, 1979, Citation1981; CitationKirk et al., 2006; CitationYang et al., 2007), integrated with sketches and drawings from research papers (CitationHuang et al., 2018; CitationLancia et al., 2019a, Citation2019b; CitationZhang et al., 2019; CitationZhong et al., 2009). Numerous geological formations were merged into nine hydrogeological units, after detailed analysis and interpretation of the available data, based on local hydrogeological features. The hydraulic conductivity dataset was developed from recovery pumping tests covering all the hydrogeological units (CitationGBGP 1980) and estimations from numerical analysis of the groundwater system in Shenzhen city (CitationLancia et al., 2019a). Average thickness and stratigraphic relationships among the identified units were represented through a stratigraphic column. Three geological sections illustrate the complex tectonic setting. Quantitative hydrogeological data come from a digitalization of CitationGGS (1979, Citation1981) maps. Recharge was converted to millimeter per year (originally expressed in l/s·km2) and catchments were manually drawn and associated to each measurement reported in CitationGGS (1979, Citation1981).
Additional information was extrapolated from satellite image sets from different years, analyzing tone, color and pattern variation among pixels. The photogeological method is fast and efficient for investigating vast areas characterized by dense sub-tropical vegetation, with scarce road accessibility. Method is based on the analysis of color, tone and pattern variation in the satellite image pixels. A Landsat dataset from 1979 was used to describe the area before the massive urbanization of the Pearl River Delta. The geological limit between loose Quaternary deposits and older bedrock was delineated via photogeological detection, at 1:50,000 scale. At the same scale, a detection of tectonic lineaments was also performed. Field surveys were performed to refine the hydrogeological conceptual models and reduce the subsoil uncertainties. With the same technique, urban areas were detected on the CNES-AIRBUS 2017 satellite images at 1:100,000 scale. In addition, a comparison between recent and older satellite datasets was used to map the lands reclaimed after 1979. The Pearl River Delta channel system was delineated from satellite images; raster files were processed to isolate the shallow water bodies, later converted in shapefiles and manually adjusted at 1:50,000 scale.
3. Geological and hydrogeological setting
Pearl River Delta is a wide basin of southern China characterized by the confluence of North, East and West Rivers (total average annual discharge 10,000 m3/s) via hundreds of natural and anthropic channels with an anastomosed geometry (). The study area is located on the southern boundary of the Cathysian block (CitationZhang et al., 2013). Stratigraphy consists of a metamorphosed Pre-Paleozoic basement overlain by a discontinuous Paleozoic to Mesozoic terrigenous sequence with carbonate units. Upper Mesozoic to Tertiary intrusions and volcanism break up the sedimentary bedrock (CitationDarbyshire & Sewell, 1997). Quaternary marine to continental facies fill the depressions in the studied area. Pre-Paleozoic to Mesozoic bedrock experienced numerous transformations via regional and contact metamorphism, increasing petrographic complexities (CitationRen et al., 2016). From a structural point of view, the study area is a Neo-Paleozoic depression, superimposed and reworked by Mesozoic to Paleozoic tectonic events. Because of the geological complexity, many authors consider the investigated area a ‘geological museum' (CitationZhang et al., 2018 and references therein). Only Tertiary strike-slip tectonics and more recent normal faulting are easily recognizable, as older structures have been obliterated. Faults are classed into three sets via their trend: NE-SW, NW-SE and W-E. The first set is evident and well-preserved; major tectonic lines shape the morphology of Pearl River Delta Basin and the coast line. Major lineaments in this trend are the Shenzhen fault, the Guangzhou-Conghua fault and the Wugui Mt. fault. Other fault sets are less developed, with a smaller scale and mostly intersecting the NE-SW main tectonic systems. Throughout the basin, shallow seismic exploration and field evidence in nearby Guangzhou reveal that the Quaternary sequence in many localities of the Pearl River Delta is faulted (CitationTang et al., 2011). Movement of Pearl River Delta faults is likely periodic or paroxysmal, surely not discontinuous or syn-sedimentary. Together with tectonics, climatic variations and subsidence affect the drainage system of the investigated area. Currently, the Pearl River Delta is a wide depression with elevations ranging from 0 to 10 m a.s.l., surrounded by steep reliefs with the highest points reaching 1200 m a.s.l. The Delta has hundreds of channels and streams connected together, increasing their density and connectivity toward the estuary. Groundwater dynamics of the depression is affected by surface water due to the flat morphology and the complicated hydraulic connection among shallow water bodies. The Quaternary sequence is characterized by an anisotropic and heterogeneous groundwater flow (CitationHuang et al., 2011). CitationZhong et al. (2009) describes two continental units and two marine units interbedded, with a total thickness varying from 20 to 90 m (CitationGGS, 1979, Citation1981; CitationYang et al., 2007). Marine silt and clay deposits impede the groundwater dynamics and limit groundwater exchange between Quaternary and bedrock aquifers. At the base of the Quaternary sequence, bedrock aquifers are confined. In fact, Tertiary reworked sequence, karst layers and cataclastic rocks constitute subsoil zones rich in groundwater (CitationCui et al., 2015; CitationLiu et al., 2017).
4. Hydrogeochemistry setting
Hydrogeochemistry of Pearl River Delta is complex due to the sea level variations at the geological time scale, accumulation of organic matter under tropical conditions as well as recent urbanization and industrialization. Water chemistry from CitationLu et al. (2008), CitationWang and Jiao (2012) and CitationHuang et al. (2013, Citation2014, Citation2018) is synthetized in the Piper diagram of . Groundwater sampled from Quaternary aquifers shows a mixing trend. From the estuary to the delta, an increment of Ca2+, Mg2+ and HCO3 - ions is observed to the detriment of the Cl-, SO4 2- and Na+. In the coastal sector, groundwater shows high salinity, elevated concentrations of As, NH4+, dissolved organic carbon and low concentrations of NO3 - and NO2 -. High salinity (total dissolved solids [TDS] up to 25,000 mg/L) derive from an initial marine transgression that flooded the Pearl River Delta and a subsequent migration of the shoreline towards the current condition during the late Holocene (CitationWang & Jiao, 2012). Reductive dissolution of iron oxyhydroxide is one of the important processes that mobilizes solid arsenic in groundwater, with concentrations up to 0.16 mg/L (CitationWang & Jiao, 2012). Abnormally high concentrations of NH4+ (up to 600 mg/L) are linked to the decomposition of organic material in the aquitard layers (CitationWang et al., 2013). Toward the north, groundwater has characteristics similar to the shallow water () with a low salinity (mean TDS 450 mg/L) (CitationHuang et al., 2014). Higher concentrations of K+ and NO3 - are directly link with discharge of domestic sewage and septic tanks (CitationHuang et al., 2018; CitationLu et al., 2008). However, CitationZhang et al. (2019) state that almost 22% of the porous aquifers of Pearl River Delta have a poor-quality, due to natural or anthropogenic conditions.
Figure 2. Piper diagram of Pearl River Delta representing groundwater and surface water, data from CitationLu et al. (2008), CitationWang and Jiao (2012), CitationHuang et al. (2013, Citation2014, Citation2018).
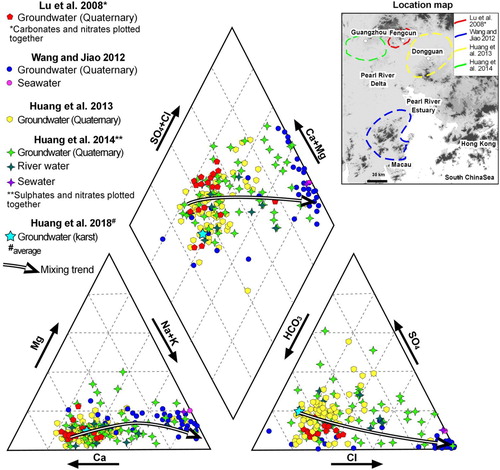
Karst sequences are confined and located beneath the Quaternary sequence. Water has high concentrations of Ca2+ and HCO3 - ions () and excellent quality (CitationHuang et al., 2018). Similarly, fractured aquifers are also confined and located beneath the Quaternary aquifers. Water chemistry is variable depending on the water-rock interaction. The infiltration of agricultural fertilizer, and domestic sewage into groundwater is responsible for the increment of TDS, K+, Na+, Cl-, SO4 2- and NO3 - in fissured aquifers. However, only 9% of the fractured aquifers have a poor water quality (CitationHuang et al., 2018).
5. Results and discussions
The Main Map describes the hydrogeological setting of Pearl River Delta. Collected information synthetizes the complex geological setting which are represented with four conceptual models. Presented models describe groundwater dynamics of rural and urban areas throughout the Pearl River Delta. The distribution of the identified conceptual models is illustrated in .
6. Hydrogeological conceptual models
6.1. Intrusion bedrock model (rural areas)
Intrusive rocks (4, upper Mesozoic intrusion unit, in Main Map) characterize the main relief of the Pearl River Delta with large intrusions of several square kilometers. Bedrock has negligible porosity and open fractures are generally sparse and do not constitute a continuous net. The unit is an extended aquitard but fracturing locally increases in the cataclastic bands. The bands constitute local fissured aquifers with groundwater flow direction parallel to the fault trend. The fissured aquifer can extend below the Quaternary, becoming confined. Mylonithic fault cores and metamorphism limit fissured aquifer extension. Towards the ground surface, subtropical conditions have created a continuous weathered blanket (CitationChen et al., 2017; CitationLancia et al., 2019b) comprising sand and silty sand (saprolite) and fractured to laminated bedrock. The weathered blanket constitutes a shallow aquifer distributed along the slope with an average thickness of 30 m. Groundwater flows parallel to the slope towards streams but anthropogenic elements such as roads or building foundations can interrupt the flow ((a)). Recharge depends on many factors including the slope acclivity and exposure, occurrence of flow landslide as well as vegetation density ((b)). Groundwater is collected along the valley incisions and contributes to the base flow of streams. Along the piedmont, farmers exploit this water for irrigation with rudimentary pipe systems. This conceptual model is also applicable to the fissured lava flows and pyroclastic flows (3, upper Mesozoic volcanic unit, in Main Map), when characterized by low porosity and hydraulic conductivity. The conceptual model is schematized in the Main Map.
Figure 4. Field evidence from Pearl River Delta: (a) contact between saprolite/weathered blanket (aquifer) and granite bedrock (aquitard), ephemeral groundwater discharge into a road ditch; (b) recharge rate frequency distribution for catchments characterized by the intrusion unit; (c) gneiss with sparse fractures; (d) recharge rate frequency distribution for catchments characterized by basement unit and/or Mesozoic to Paleozoic terrigenous unit; (e) contractional tectonic contact between sandstone and siltstone intersected by a cataclastic zone that locally increases rock-mass permeability; (f) karstified and mineralized fracture in marble with flowing groundwater, observed during tunneling.
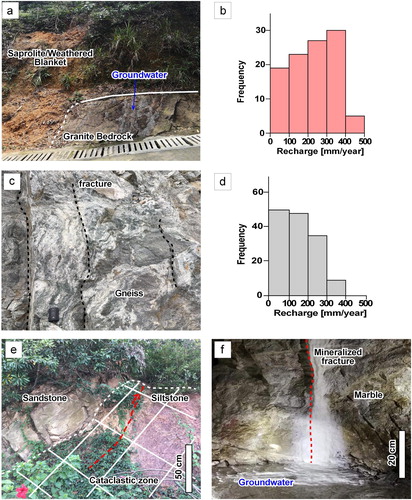
6.2. Sedimentary bedrock model (rural areas)
The terrigenous bedrock (5 Mesozoic terrigenous unit, 6 Paleozoic terrigenous unit, 8 Paleozoic terrigenous clastic unit, 9 Basement unit in Main Map) is characterized by low hydraulic conductivity. Metamorphic processes confer stiffness to the rock masses reducing fracture aperture and length ((c)). Due to the tropical conditions, a continuous weathered blanket covers the bedrock. The blanket is generally thinner when compared to the intrusive rocks. Recharge depends on many factors including the slope acclivity and exposure, occurrence of flow landslide as well as vegetation density ((d)) (CitationLancia et al., 2019b). Along the surface, cataclastic bands ((e)) or fracture zones are less developed than in the granite intrusions and the fissured aquifers have a lower transmissivity. The carbonate sequences have a different hydrogeological behavior. Limestone and dolostone (Paleozoic carbonate unit 7 in Main Map) are fissured aquifers with modest transmissivity. If exposed, aquifers are strongly karstified due to the sub-tropical conditions. Karst basins are mostly preserved at the bottom of the basins and fluvial valleys as carbonate media are resistant to weathering processes if metamorphosed. Current drainage is also mature in marble layers with open fractures, also mineralized ((f)). When located below the Quaternary sequence, these aquifers are confined and can exchange groundwater flow with the coarser Quaternary sequence. The conceptual model of the sedimentary bedrock units is schematized in the Main Map.
6.3. Quaternary plain models (urban and rural areas)
The groundwater table is flat and water exchange between groundwater and shallow water occurs throughout the basin because of the flat morphology. In the urban areas, pressurized water-supply pipes leak from fractures and cracks recharging the shallow aquifers (CitationLerner, 2000). On the other hand, urban sewers in free flow condition drain groundwater when the groundwater table close to the ground level (CitationLancia et al., 2019b). As a result of this favorable condition, leakage represent a minor issue throughout the delta. In urban areas, the main pollution sources are chemical spillages, urban waterlogging and illegal discharge of factories to channels and streams.
In rural areas, the hydrogeological setting is similar. Continental and marine sediments with different grain size create multi-layer aquifers, up to 90 m thick. Clay lenses are aquitards and sand layers the aquifers. Irrigation with surface water mixed with domestic sewage and outdated septic tanks spread contaminants into the shallow aquifers (CitationHuang et al., 2018; CitationLu et al., 2008). Clay lenses preserve confined aquifers from contaminant; currently, urbanization and industrialization do not affect the confined groundwater resources of karst aquifers and is limited on the fractured aquifers. However, groundwater overexploitation of confined aquifers should be avoided in polluted areas as it will accelerate cross-contamination from shallow aquifers. These conceptual models are schematized in the Main Map.
7. Conclusions
The hydrogeological setting of the Pearl River Delta is synthetized and represented via mapping and conceptual models. With a recharge rate up to 500 mm/year, shallow aquifers of the mountain sectors contribute to river and streams as the bedrock is generally impervious. Along the delta, groundwater resources have a poor quality due to saline intrusions and complex geochemical processes. Below the Quaternary deposits, pristine confined aquifers (fissured and karstified) can contribute to satisfy the increasing groundwater water demand via water-wells. In urban areas, leakage from sewers is not a major issue as groundwater likely seeps into the sewers throughout the basin. Main contaminant sources are chemical spillages, urban waterlogging, and illegal discharge of factories into channels and streams. Confined aquifers are sensitive to pollution when groundwater dynamics are altered by human activities. In rural and urban areas, new policies are recommended including irrigation with clean water, a modernization of the septic tanks and construction of sewers and water treatment plants. If shallow aquifers are polluted, exploitation of confined aquifers should be discouraged to avoid contamination.
Software
Collected data from literature and field activity were stored in a geodatabase and represented in a GIS environment (ArcGis 10.6.1 version).
A0map.pdf
Download PDF (14 MB)Acknowledgements
Authors are grateful for the efforts of Associate Editor A. Merschat and Reviewers S. Shen and S. Viaroli.
Disclosure statement
No potential conflict of interest was reported by the author(s).
Additional information
Funding
References
- Chen, M. , Shen, S. L. , Wu, H. N. , Wang, Z. F. , & Horpibulsuk, S. (2017). Geotechnical characteristics of weathered granitic gneiss with geo-hazards investigation of pit excavation in Guangzhou, China. Bulletin of Engineering Geology and the Environment , 76 (2), 681–694. https://doi.org/10.1007/s10064-016-0915-1
- Chen, Y. Y. , Yang, K. , He, J. , Qin, J. , Shi, J. C. , Du, J. Y. , & He, Q. (2011). Improving land surface temperature modeling for dry land of China. Journal of Geophysical Research , 116 (D20104), 1–15. https://doi.org/10.1029/2011JD015921
- Cui, Q. L. , Shen, S. L. , Xu, Y. S. , Wu, H. N. , & Yin, Z. Y. (2015). Mitigation of geohazards during deep excavations in karst regions with caverns: A case study. Engineering Geology , 195 , 16–27. https://doi.org/10.1016/j.enggeo.2015.05.024
- Darbyshire, D. P. F. , & Sewell, R. J. (1997). Nd and Sr isotope geochemistry of plutonic rocks from Hong Kong: implications for granite petrogenesis, regional structure and crustal evolution. Chemical Geology , 143 (1–2), 81–93.
- GBGP-Geological Bureau of Guangdong Province . (1980) Report on regional hydrogeological survey, scale 1:200.000, unpublished pp. 231. (In Chinese).
- Guangzhou Geological Survey-GGS . (1979). Synthetic hydrogeological map Republic of China. F-49 series scale 1:200,000. China geological survey.
- Guangzhou Geological Survey-GGS . (1981). Synthetic hydrogeological map Republic of China F-50 series scale 1:200,000. China geological survey.
- Huang, G. , Chen, Z. , Liu, C. , Sun, J. , & Wang, J. (2014). Impact of human activity and natural processes on groundwater arsenic in an urbanized area (South China) using multivariate statistical techniques. Environmental Science Pollution Research , 21 (22), 13043–13054. https://doi.org/10.1007/s11356-014-3269-x
- Huang, G. , Liu, C. , Sun, J. , Zhang, M. , Jing, J. , & Li, L. (2018). A regional investigation on factors controlling the groundwater chemistry of various aquifers in a rapidly urbanized area: A case study of the Pearl River Delta. Science of the Total Environment , 625 , 510–518. https://doi.org/10.1016/j.scitotenv.2017.12.322
- Huang, G. , Sun, J. , Ying, Z. , Jing, J. , Zhang, Y. , & Liu, J. (2011). Distribution of arsenic in sewage irrigation area of Pearl River Delta, China. Journal of Earth Science , 22 (3), 396–410. https://doi.org/10.1007/s12583-011-0192-7
- Huang, G. , Sun, J. , Zhang, Y. , Chen, Z. , & Liu, F. (2013). Impact of the anthropogenic and natural processes on the evolution of groundwater chemistry in a rapidly urbanized coastal area, South China. Science of the Total Environment , 463 , 209–221. https://doi.org/10.1016/j.scitotenv.2013.05.078
- Jiao, J. J. , Ding, G. , & Leung, C. M. (2006). Confined groundwater near the rockhead in igneous rocks in the Mid-Levels area, Hong Kong, China. Engineering Geology , 84 (3-4), 207–219. https://doi.org/10.1016/j.enggeo.2006.02.006
- Kirk, P. A. , Sewell, R. J. , Campbell, S. D. G. , Fletcher, C. N. J. , Lai, K. W. , & Li, X. C. (2006). Geological map of Hong Kong, scale 1:100,000. Hong Kong Geological Survey.
- Lancia, M. , Zheng, C. , He, X. , Lerner, D. N. , & Andrews, C. (2019b). Groundwater complexity in urban catchments: Shenzhen, southern China. Groundwater . https://doi.org/10.1111/gwat.12935
- Lancia, M. , Zheng, C. , He, X. , Lerner, D. N. , Andrews, C. , & Tian, Y. (2020). Hydrogeological constraints and opportunities for “Sponge City” developments: Shenzhen, southern China. Journal of Hydrology Regional Studies . https://doi.org/10.1016/j.ejrh.2020.100679
- Lancia, M. , Zheng, C. , Yi, S. , Lerner, D. N. , & Andrews, C. (2019a). Analysis of groundwater resources in densely populated urban watersheds with a complex tectonic setting: Shenzhen, southern China. Hydrogeology Journal , 27 (1), 183–194. https://doi.org/10.1007/s10040-018-1867-2
- Lerner, D. N. (2000). Identifying and quantifying urban groundwater recharge: A review. Hydrogeology Journal , 10 (1), 143–152. https://doi.org/10.1007/s10040-001-0177-1
- Liu, X. X. , Xu, Y. S. , Cheng, W. C. , Shen, S. L. , & Horpibulsuk, S. (2017). Investigation of hydraulic parameters of a weathered mylonite fault from field pumping tests: A case study. Bulletin of Engineering Geology and the Environment , 76 (4), 1431–1448. https://doi.org/10.1007/s10064-016-0910-6
- Lu, Y. , Tang, C. , Chen, J. , & Sakura, Y. (2008). Impact of septic tank systems on local groundwater quality and water supply in the Pearl River Delta, China: Case study. Hydrological Processes , 22 (3), 443–450. https://doi.org/10.1002/hyp.6617
- Ren, D. J. , Shen, S. L. , Cheng, W. C. , Zhang, N. , & Wang, Z. F. (2016). Geological formation and geo-hazards during subway construction in Guangzhou. Environmental Earth Sciences , 75 (11), 934. https://doi.org/10.1007/s12665-016-5710-6
- Tang, Y. , Chen, G. , Peng, Z. , & Zhang, K. (2011). Late Quaternary tectonics of the Pearl River Delta, SE China. Evidence from Xilingang. Geodinamica Acta , 24 (3–4), 133–139. https://doi.org/10.3166/ga.24.133-139
- Wang, X. , Li, H. , Yang, J. , Zheng, C. , Zhang, Y. , An, A. , Zhang, M. , & Xiao, K. (2017). Nutrient inputs through submarine groundwater discharge in an embayment: A radon investigation in Daya Bay, China. Hydrogeology Journal , 551 , 784–792. https://doi.org/10.1016/j.jhydrol.2017.02.036
- Wang, X. S. , Jiao, J. J. , Wang, Y. , Cherry, J. A. , Kuang, X. , Liu, K. , Lee, C. , & Gong, Z. (2013). Accumulation and transport of ammonium in the Pearl River Delta (China) in the last 10,000 years: Conceptual and numerical models. Hydrogeology Journal , 21 (5), 961–976. https://doi.org/10.1007/s10040-013-0976-1
- Wang, Y. , & Jiao, J. J. (2012). Origin of groundwater salinity and hydrogeochemical processes in the confined Quaternary aquifer of the Pearl River Delta. Journal of Hydrology , 438–439 , 112–124. https://doi.org/10.1016/j.jhydrol.2012.03.008
- Yang, Z. , Yi, S. , & Wang, X. (2007). Hydrogeological map of Shenzhen, scale 1:50,000. Shenzhen Geological Construction, Shenzhen.
- Zhang, F. , Huang, G. , Hou, Q. , Liu, C. , Zhang, Y. , & Zhang, Q. (2019). Groundwater quality in Pearl River Delta after the rapid expansion of industrialization and urbanization: Distributions, main impact factors, and driving forces. (Journal of Hydrology , 577 ). Available Online. https://doi.org/10.1016/j.jhydrol.2019.124004
- Zhang, G. W. , Wang, Y. J. , Li, S. Z. , Dong, Y. P. , Liu, S. F. , He, D. F. , Cheng, S. Y. , Lu, R. K. , & Yao, A. P. (2013). Tectonics of South China continents and its implications. Science China Earth Science , 56 (11), 1804–1828. https://doi.org/10.1007/s11430-013-4679-1
- Zhang, N. , Shen, J. S. , Zhou, A. N. , & Arulrajah, A. (2018). Tunneling induced geohazards in mylonite rock faults with rich groundwater: A case study in Guangzhou. Tunneling and Underground Space Technology , 74 (2018), 262–272. https://doi.org/.1016/J.TUST.2017.12.021 doi: 10.1016/j.tust.2017.12.021
- Zhong, Y. , Yim, W. W. S. , Tu, F. , & Huang, G. (2009). Late Quaternary environmental changes in the Pearl River Delta mouth region, China. Quaternary International , 206 (1-2), 35–45. https://doi.org/10.1016/j.quaint.2008.10.012