ABSTRACT
The Punta Bianca Unit (NW Italy) is a continent-derived metamorphic unit that experienced underthrusting and later exhumation in the hinterland sector of the Northern Apennines fold-and-thrust belt. We present a novel 1:5000 scale geological map that aims to illustrate the polyphase tectonic evolution of the Punta Bianca Unit and its relationships with non-metamorphic units. The geologic data presented in the map are coupled with the structural analysis of the main tectonic elements, lithostratigraphic, finite strain and microstructural data that allow to highlight the tectonic history of the study area. In particular, we recognized that the Punta Bianca Unit underwent an early phase of underplating (D1), followed by syn-orogenic exhumation to shallow crustal levels and coupling with the overlying Tuscan Nappe (D2), and nappe stacking/refolding (D3). Low-angle semibrittle (D4) and high-angle brittle (D5) faulting affected both the Punta Bianca Unit and the Tuscan Nappe during the latest stages of deformation.
1. Introduction
Orogenic wedges are the result of active convergence between two colliding continental plates. They consist of stacked tectonic nappes, accreted from the underthrusted plate at shallow to deep crustal levels. While thrusting controls the growth of orogenic wedges, extensional and gravitational instabilities may shape their long-term evolution, and are responsible for the exhumation from deep structural levels (CitationKearey et al., 2009; CitationKonstantinovskaya & Malavieille, 2011; CitationPlatt, 1986; CitationSaffer & Bekins, 2002; CitationStern, 2002). Extension within orogenic wedges is not necessarily the result of a change in tectonic regime from contractional to extensional (e.g. CitationRing & Glodny, 2010), but may be determined by local and transient instabilities related to the internal dynamics of the wedge. For example, CitationDahlen (1990) and CitationDavis et al. (1983) showed that continuous underthrusting may trigger gravitational collapse of the orogenic wedge, if the critical taper slope stability angle is exceeded (see also CitationPlatt, 1986). Many authors have also demonstrated that the coeval activity of oppositely verging thrusts and low-angle normal faults may drive the exhumation of deep-seated metamorphic rocks (e.g. CitationGrujic et al., 1996; CitationLaw et al., 2004; CitationRing & Glodny, 2010). Studying exhumed metamorphic units represents, hence, the key to understand the tectonic and metamorphic processes that control the internal dynamics of orogenic wedges.
In this work, we investigate the tectonic structures exposed in the Punta Bianca Unit (PBU). The PBU represents the innermost exposure among the exhumed continental units that occupy the lower structural levels in the Northern Apennines. We present a new, 1:5000 scale geological map of the PBU (Main Map), supported by novel structural data that show, at greater resolution than previous studies, the tectonic structures of this key sector of the belt. We provide the first detailed microstructural description of the lithologies of the PBU and finite-strain analysis data. This contribution highlights an example of a polyphase tectonic history that occurred during underthrusting, syn-orogenic exhumation and stacking of an underplated metamorphic unit within an orogenic wedge.
2. Geologic setting
The Punta Bianca Unit (PBU) belongs to the Tuscan Metamorphic Units (TMUs), a group of exhumed nappes exposed in the Northern Apennines hinterland (a, b). The TMUs consist of a Variscan basement covered by Triassic to Oligocene–Miocene metasedimentary sequences (CitationBoccaletti et al., 1971; CitationCarmignani et al., 2001; CitationElter, 1960). Alpine deformation transposed the structures related to the Variscan event that are only locally preserved at a few sites (e.g. CitationFranceschelli et al., 2004 and references therein; CitationMolli, 2008). Alpine metamorphism of the TMUs peaked at conditions ranging from blueschist to sub-greenschist-facies conditions (CitationDi Pisa et al., 1985; CitationFranceschelli et al., 1986; CitationGiorgetti et al., 1998; CitationLo Pò & Braga, 2014; CitationMolli et al., 2000; CitationPapeschi et al., 2020; CitationTheye et al., 1997). The TMUs are regionally overlain by (1) the Tuscan Nappe (b), consisting of Triassic–Oligocene–Miocene passive margin sequences with anchizone-facies metamorphism (CitationBaldacci et al., 1967; CitationCarmignani et al., 1978; CitationCarosi et al., 2003) and (2) the Ligurian and Subligurian Units, comprising oceanic and ocean-continent transition sequences that experienced anchizone to lower-blueschist facies metamorphism during the Alpine Cycle (CitationElter, 1975; CitationMarroni et al., 2001).
Figure 1. Geological setting: (a) Tectonic sketch map of Italy and neighboring regions. Black lines are thrust fronts of Alpine belts; (b) simplified geological map of the Northern Apennines and Corsica (modified after CitationPapeschi et al., 2017). AA: Alpi Apuane; MP: Monti Pisani; MR: Monticiano-Roccastrada; MTR: Mid-Tuscan Ridge; (c) Tectonic sketch map of the La Spezia area highlighting the location of the study area (modified after CitationClemenzi et al., 2015). PBU: Punta Bianca Unit; TD: Tellaro Detachment.
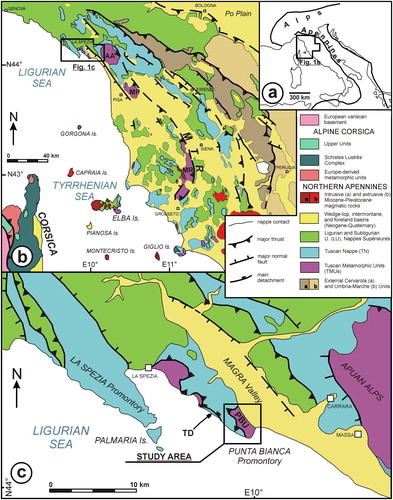
The PBU crops out at the southern tip of the Punta Bianca Promontory in the Gulf of La Spezia (), underneath the Tuscan Nappe (TN) and the Ligurian Units (LU) exposed to the NW (c; CitationCarosi et al., 2003; CitationFederici & Raggi, 1975; CitationMontomoli et al., 2001; CitationStorti, 1995). The TN experienced a polyphase tectonic history that involved the early development of a regional W-verging fold (La Spezia fold; CitationCarosi et al., 2003; CitationCarter, 1992; CitationGianmarino & Giglia, 1990; CitationMontomoli, 2002) and late faulting, mainly along the east-verging Tellaro Detachment (c; CitationClemenzi et al., 2015; CitationMolli et al., 2018a; CitationStorti, 1995). The TN was affected by very low-grade metamorphism, with peak temperatures around ∼200°C (CitationMolli et al., 2011; CitationMontomoli et al., 2001).
The PBU consists of a Paleozoic–Triassic metasedimentary sequence (; CitationCiarapica & Passeri, 2005; CitationMartini et al., 1986; CitationRau et al., 1985) that experienced lower greenschist-facies metamorphism constrained at temperatures of ∼300–400°C and pressures between ∼0.4 GPa (CitationFranceschelli et al., 1986) and 0.7 GPa (CitationLo Pò et al., 2016). The Alpine tectonic evolution of the PBU was previously described by CitationCarosi et al. (1991) and CitationStorti (1995), who recognized an earlier E-verging nappe stacking event (D1), followed by D2 and D3 symmetric W- and E-verging syn-orogenic extension of the overthickened thrust wedge.
Figure 2. Schematic stratigraphic column of the PBU and the overlying TN in the study area. Letters from A to R mark the lithofacies by CitationRau et al. (1985). Corresponding formations after CitationAbbate et al. (2005) are shown on the right.
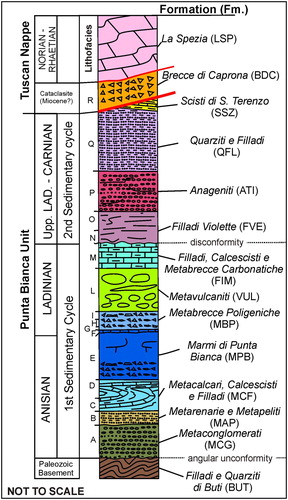
3. Methods
The map at 1:5000 scale covers an area of about 10 km2, comprised between 44°2′10″N–44°4′10″N of latitude and 9°56′20″E–9°59′20″E of longitude and corresponding to the southeastern edge of the Punta Bianca Promontory (Liguria, Italy). The mapped area is outlined by the Costa Celle ridge to the north, the Pian della Chiesa and Costa di Murlo ridges to the west, the Magra River to the east and the Ligurian Sea to the south.
The topographic base map of the geological map (converted in vector-format from a raster, Carta Tecnica Regionale – Regione Liguria sc. 1:5000 1990/2006) using the Gauss-Boaga projection and the Hayford-Roma40/West datum. CitationAbbate et al. (2005) realized the previous geological map of the area at 1:50,000 scale as part of the CARG project of the Istituto Superiore per la Protezione e la Ricerca Ambientale (ISPRA). The map we present expands the above-mentioned work and includes new lithological, structural (measures of foliations, lineations and fold axes) and petrographic data, aiming to characterize the deformation style of the PBU. Lineations and foliations were classified according to CitationPasschier and Trouw (2005) and fold following CitationRamsay and Huber (1987). We realized standard, oriented thin sections for microstructural analysis by cutting samples parallel to the object lineation and perpendicular to the main foliation. Microstructural observations were carried out using standard polarized optical microscopy at the microtectonics lab and the Philips XL30 Scanning Electron Microscope (SEM) at the University of Pisa equipped with an energy-dispersive X-ray spectroscopic system (EDAX). Chemical analysis of mineral grains were performed using an accelerating voltage of 20 keV and a beam current of 5 nA. We selected five samples, two of polymictic metabreccia, two of monomictic metabreccia and one of metavolcanite for finite strain analyses, following the Rf/ϕ method described by Dunnet (Citation1969). Considered strain markers were deformed clasts in the metabreccia and vesicles in the metavolcanite (Main Map).
4. Lithostratigraphy
4.1 The Punta Bianca Unit
The PBU shows a ∼150-m-thick Triassic succession overlying a Paleozoic, polymetamorphic basement (). The basement is mapped as a single formation (Filladi e Quarziti di Buti Fm.; BUT), consisting of phyllite and metasandstone with thin quartzite layers. The protolith age was referred to the Upper Ordovician by CitationAbbate et al. (2005) based on a correlation with the nearby Apuan Alps (). CitationRau et al. (1985) recognized within the Triassic metasedimentary sequence two distinct sedimentary cycles: (1) the first cycle of Anisian–Ladinian age and (2) the second cycle of Upper Ladinian–Carnian age, separated by a disconformity. CitationRau et al. (1985) distinguished 16 lithofacies (coded in letters from A to R; ). CitationAbbate et al. (2005) grouped the lithofacies into 10 formations, because most lithofacies describe subtle lithological variations identifiable only in a well-exposed section along the coast from Punta Bianca to Cala Marola (Main Map) that are not discernible at map scale (e.g. lithofacies G–H–I in ).
The Anisian–Ladinian cycle () has been interpreted as the sedimentary fill of a failed rift system (CitationMartini et al., 1986; CitationRau et al., 1985). The cycle starts with massive, coarse-grained green metaconglomerate that contains lenses of phyllite and metasandstone (Metaconglomerati Fm.; MCG), referred to the Anisian and interpreted as a continental deposit. MCG is transitional and fining upward to layers of metasandstone, phyllite, and fine-grained metaconglomerate (Metarenarie e Metapeliti Fm.; MAP) of Anisian age and continental environment. Metaconglomerate layers in both MCG and MAP range from clast- to matrix-supported and contain phyllite and quartzite clasts, eroded from the Variscan basement. MAP is transitional to diplopora-bearing metalimestone and black phyllite of Anisian age and open shelf environment (Metacalcari, Calcescisti e Filladi Fm.; MCF). MCF passes upward to massive marble with thin layers of calcschist and lenses of monomictic carbonate metabreccia containing clasts with fragments of diplopora, crinoids and ammonites (Marmi di Punta Bianca Fm.; MPB). MPB marks the transition from the Anisian to the Ladinian and is interpreted as a scarp breccia supplied by carbonate platforms. MPB is overlain by matrix-supported polymictic metabreccia lenses, containing clasts of marble, phyllite and quartzite, interlayered with metasandstone and phyllite (Metabrecce Poligeniche Fm.; MPB), interpreted as Ladinian scarp deposits. Upward, the sequence is characterized by metasandstone and phyllite that envelop lenses of metabasalt showing an alkaline affinity (Metavulcaniti Fm.; VUL) of Ladinian age (CitationRicci & Serri, 1975; CitationStoppa, 1985), emplaced in a relatively deep marine environment as suggested by the presence of pillow structures. Anisian – Ladinian cycle () ends with a sequence of marble, calcschist, phyllite, and polymictic metabreccia deposited in a deep marine environment (Filladi, Calcescisti e Metabrecce Carbonatiche Fm.; FIM). According to CitationRau et al. (1985), FIM contains paleokarstic features that record Middle Triassic emersion above the sea level.
The Upper Ladinian–Carnian cycle (; CitationMartini et al., 1986; CitationRau et al., 1985) indicate, at regional scale, the base of the Alpine cycle and is marked by the deposits of the Verrucano Group (e.g. CitationCassinis et al., 2018), representative of continental and coastal deposits of arid environment. From bottom to top, the Verrucano Group comprises (1) violet phyllite and metasiltstone with lenses of metaconglomerate and quartzite of Upper Ladinian–Carnian age (Filladi Violette Fm.; FVE), (2) quartz-metaconglomerate, with thin layers of quartzite and phyllite of Upper Ladinian–Carnian age (Anageniti Fm.; ATI) and (3) interlayered quartzite and violet phyllite (Quarziti e Filladi Fm.; QFL), referred to the Carnian. Along the tectonic contact with the Tuscan Nappe, lenses of phyllite and quartzite with layers of metalimestone (Scisti di S. Terenzo Fm.; SSZ) cap the Verrucano Group. This transitional siliciclastic–carbonatic formation is interpreted to mark the transition to the Upper Triassic carbonate platform deposits that characterize the Tuscan Sequence (e.g. CitationCassinis et al., 2018; CitationCiarapica & Passeri, 2005).
4.2 The Tuscan Nappe
The Tuscan Nappe (TN) in the study area is characterized only by two formations: a Miocene tectonic breccia (Brecce di Caprona Fm.; BDC; CitationCarosi et al., 2018) overlain by the Norian–Rhaetian La Spezia Fm. (LSP) (). The tectonic breccia consists of a carbonatic matrix containing clasts of limestone, dolomitic limestone and, minor quartzite and phyllite. They derive from original alternations of evaporite, dolomite and limestone, known as Calcare Cavernoso Fm. (age: Norian; CitationGandin et al., 2000; CitationVighi, 1958), that underwent strong tectonization and later karstic reworking during the Oligocene–Miocene (e.g. CitationBurckhardt, 1946). The La Spezia Fm. consists of interlayered limestone, dolomitic limestone, marl and grey-blackish shale of carbonatic ramp environment. Oolitic and lumachella limestone are locally present.
5. Structural analysis
5.1 Map-scale structures
In the study area (c), the TN and the PBU are arranged in an open, km-scale antiform (interpreted as a F3 fold, see Section 5.2) characterized by a (30–60°) W–SW-dipping western flank (a, b), a gentler (0–15°) E–NE dipping eastern flank, and a periclinal plunge of about ∼5° to the N–NW (). The western flank of the antiform culminates in a recumbent SW-verging hectometer-scale F2 fold (see par. 5.2) that affects the formations of the PBU (a–d). An F2 structure with similar geometry but opposite vergence occurs in the northern part of the study area (Ameglia area) (Main Map).
Figure 3. Structures exposed along the D–D’ cross section (see Main Map). From West to East: (a) the TN overlies the W-dipping formations of the PBU. (b) W-dipping attitude of the formations of the PBU. (c) Overview of the contact between the PBU and the TN close to the main W-verging F2 fold (hinge zone in the Verrucano group), crosscut by a D4 shear zone (SZD4). (d) Contact between the Paleozoic basement and the Anisian–Ladinian formations. (e) D5 normal faults affecting the contact between BUT and MCG on the eastern side of the D3 antiform.
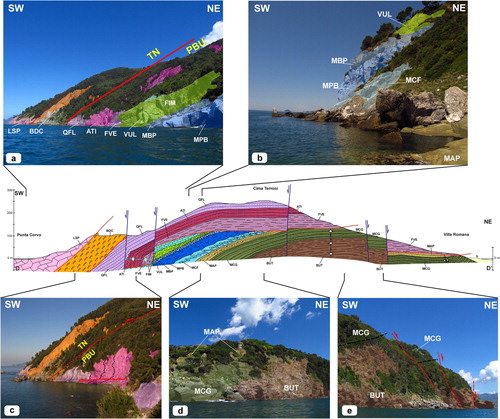
The tectonic contact between the PBU and the TN, refolded by the antiformal stack, strikes about NW–SE and dips to the W between 40–50° (a, c; Montemarcello area) and 10–20° (Ameglia area) (Main Map). At map scale, the contact is marked by lenses of cataclasites of the Brecce di Caprona Fm. in the TN and by tectonic slices of the upper formations of the Verrucano Group in the PBU. Due to the incompetent nature of the Brecce di Caprona Fm., no clear kinematic indicators can be observed. A second-order top-to-E–NE shear zone with ramp-and-flat geometry related to the D4 event (see Section 5.2) slices the Verrucano group over the lower formation of the PBU and truncates the hinge zone of the F3 antiformal stack (). In the Ameglia area, this thrust elides the Anisian–Ladinian formations and the Verrucano Group overlies directly the Paleozoic basement (Main Map). NW–SE striking high-angle normal faults, dipping to both the SW and the NE, segment the first-order folds and tectonic contacts (e). High-angle normal and transcurrent faults, oriented nearly E–W and N–S, segment the first-order folds and tectonic contacts (e, Main Map).
5.2 The Punta Bianca Unit: mesoscale structures
The PBU shows a polyphase deformation history, characterized by three ductile tectonic phases (D1–D2–D3), overprinted by a semibrittle (D4) and a brittle (D5) faulting events.
The first tectonic phase (D1) is marked by a relic slaty cleavage (S1) associated with locally preserved isoclinal F1 folds, strongly refolded by F2 folds. The S1 cleavage is commonly parallelized to the bedding (S0) (a) and occurs mostly within microlithons of the S2 cleavage (see below). The S1 cleavage is commonly parallelized to the bedding (S0).
Figure 4. Mesoscale features of the PBU: (a) Quartz-rich metasandstone in the Filladi e Quarziti di Buti Fm. showing a continuous S1 foliation preserved between S2 cleavage domains. (b) SW-verging tight F2 folds developed in the Metacalcari, Calcescisti e Filladi Fm. (c) Relationships between bedding (S0) and the main foliation (S2), defined by flattened marble and phyllite clasts in the Metabrecce Poligeniche Fm. (d) Chocolate tablet boudinage of a grey dolomite vein in the Metacalcari, Calcescisti e Filladi Fm. Gaps are filled by calcite.

The second tectonic phase (D2) forms recumbent non-cylindrical F2 folds with tight to isoclinal geometry (b) and subhorizontal N–S to NW–SE trending A2 axes. F2 folds constitute the major map-scale features, forming hectometer-sized structures characterized by SW- (cross section D-D’; ) and NE-vergence (cross section A–A’), respectively in the western and eastern part of the study area. Mesoscale F2 folds display S- and M-type geometries, forming parasitic structures around map-scale structures (c and b). The hinge zones and reverse limbs of F2 folds are commonly sheared along top-to-W–SW shear zones (SZD2) with 1–10 mm thickness and displacements in the range of a few centimeters. Object lineations (L2), associated to SZD2 are defined by stretched aggregates of quartz and calcite and trend predominantly SW–NE. The S2 foliation represents the main mesoscale foliation. The S2 foliation is a crenulation cleavage defined by the preferred orientation of mineral grains and aggregates and by flattened and re-oriented objects, such as vesicles (Metavulcaniti Fm.), clasts of carbonatic material (c; Marmi di Punta Bianca Fm.), and clasts of quartzite, schist, and carbonates (Metabrecce Poligeniche Fm.). Finite strain analyses on these markers allowed to estimate deformation by dominant flattening, plotting within the flattening field of Flinn's diagram (1965) with K values ranging from 0.013 and 0.373 (Main Map).
An additional marker of deformation in the flattening field is represented by chocolate tablet boudinage structures that are commonly developed in dolomite veins parallel to the S2 foliation (d). These veins are boudinated along roughly perpendicular NW–SE and NE–SW directions, defined by oriented calcite and quartz fibers that fill the gaps between the boudins. Boudinage of strong and competent layers (e.g. Anageniti Fm.) surrounded by incompetent phyllites, where the S2 foliation is necked, is also common (a). Upright to gently NE-verging open F3 folds with weakly non-cylindrical geometry mark the third tectonic phase (D3). F3 folds refold at map-scale both the S2 foliation, which dips up to 20° to the SW- and NE respectively in the western and eastern part of the study area (), and the tectonic contact between the PBU and the TN (see Section 5.1). A3 axes trend NW–SE and are almost coaxial with A2 axes. The F2–F3 interference pattern is of type 3, according to the classification by CitationRamsay and Huber (1987). The S3 axial plane foliation is a crenulation cleavage that strikes NW–SE and dips towards the W (30–60°) (b). The S3 is characterized by millimetric to centimetric spacing and is well-developed only in fine-grained lithologies, such as phyllites. Locally, D2 boudins are inverted by the D3 phase, producing small-scale W-verging thrusts (c).
Figure 5. Mesoscale features of the PBU: (a) Symmetric boudinage in the Anageniti Fm. Quartz veins separate boudins of a metaconglomerate layer, whereas the S2 foliation is necked in the surrounding phyllite. (b) Detail of the S2 foliation, crenulated by steep W-dipping S3 foliation planes in a metapelitic interlayer of the Metarenarie e Metapeliti Fm. (c) Small scale D3 thrusts with centimetric westward displacements affecting pre-existing boudins of metasandstone layers at the base of the Metabrecce Poligeniche Fm. (d) D4 shear zones developed in phyllite and quartzite (Quarziti e Filladi Fm.). Top-to-NE sigmoidal structures, containing deformed S3 and S2 foliations, are dragged along top-to-NE low-angle Riedel shears.
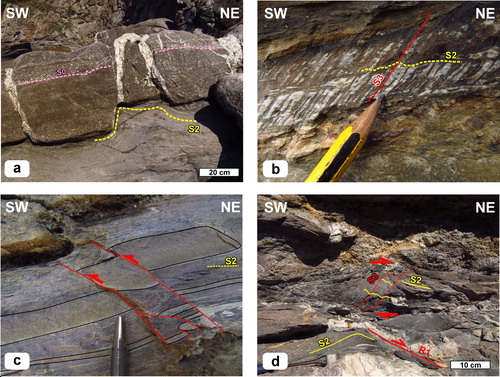
The fourth tectonic phase (D4) is associated with gently SW-dipping to subhorizontal top-to-ENE shear zones (SZD4), with displacements, ranging from a few centimeters up to hundreds of meters. This phase is responsible for the eastward tectonic slicing of the Verrucano group over the Anisian-Ladinian sequence, affecting also the Tuscan Nappe (). The architecture of D4 shear zones, well observed in the southern part of the study area (Cala Marola, Main Map), displays: (1) a core zone with a foliated cataclasite that contains sheared sigmoidal lenses of wall rocks with deformed S3 foliation; (2) a fractured damage zone in the host rocks (d); (3) synthetic P and R1 and antithetic R2 Riedel shears (nomenclature after CitationLogan et al., 1992), associated with drag folds and sigmoidal objects indicating top to-E/NE kinematics (d).
The latest structures in the area, marking the D5 tectonic phase, are represented by sets of N–S to NW–SE and E–W high-angle faults with normal to transcurrent kinematics. These structures are only locally exposed along the coast (e.g. e) and their orientation is extrapolated from their attitude at map scale (Main Map).
6. Petrography and microstructures
The PBU sequence experienced greenschist-facies metamorphism and polyphase deformation, resulting in a wide range of microstructures. For descriptive purposes, we grouped the investigated suite of selected thin sections into seven main lithotypes: (i) phyllite and metasandstone, (ii) calcschist, (iii) polymictic metabreccia, (iv) monomictic metacarbonate breccia, (v) marble, (vi) metabasalt and (vii) quartz-rich metaconglomerate.
A foliated microfabric characterizes phyllite and metasandstone (i) with fine-grained Wmca + Chl + Qtz + Ab + Ep + Fe-Ti oxides (abbreviations after CitationSiivola & Schmid, 2007) defining the S1 and S2 foliations (a). The S1 cleavage is preserved in quartz-rich microlithons between S2 cleavage domains. Spaced, opaque-rich surfaces (a) define a younger crenulation cleavage (S3). Epidote, albite, and tourmaline form fine-grained porphyroblasts in mica-rich domains. Quartz shows granoblastic microstructure with serrated boundaries and small bulges.
Figure 6. Micrographs collected at (a–b–c–d–g–h) crossed and (e–f) plane polarized light. Mineral abbreviations after CitationSiivola and Schmid (2007). (a) Relationships between the S1 slaty cleavage and the S2–S3 crenulation cleavages in a metasandstone (Metarenarie e Metapeliti Fm.). (b) Intertectonic epidote grain that overgrows the S1 foliation, crenulated by the S2 in the surrounding matrix, observed in a calcschist (Metacalcari, Calcescisti e Filladi Fm.). (c) Deformed quartz and calcite grains in a calcschist (Marmi di Punta Bianca Fm.) showing a shape preferred orientation parallel to the S2 foliation. (d) Face-controlled quartz strain fringes developed around euhedral pyrite grains, parallel to the S2 foliation (Metabrecce Poligeniche Fm.). (e) Flattened calcite-quartz vesicles surrounded by a greenish opaque-Ca-amphibole-chlorite matrix in a metabasalt (Metavulcaniti Fm.). (f) Relic euhedral magmatic plagioclase grain pseudomorphosed to a mix of sericite and chlorite, surrounded by a chlorite + opaque + Ca-amphibole matrix (Metavulcaniti Fm.). (g) Bulging (red arrow) of quartz in a vesicle within metabasalts (Metavulcaniti Fm.). (h) Example of the heterogeneous microstructures shown by quartzite clasts in the Anageniti Fm. Polygonal grain boundaries coexist with cracks, subgrains, trails of recrystallized quartz grains, and deformation bands.
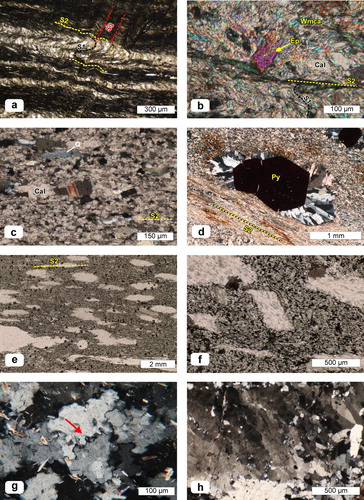
Subparallel quartz-rich, calcite-rich and white mica + chlorite + Fe-Ti oxide rich layers with a preferred orientation parallel to the S2 foliation define the foliated structure of calcschist (ii). The S1 cleavage, defined by Wmca + Chl + Qtz + Cal + Fe-Ti oxide grains, occurs locally preserved in microlithons. Epidote is present as intertectonic porphyroblasts (b). Quartz and calcite grains show serrated grain boundaries and a moderate shape preferred orientation parallel to the S2 foliation (c). Undulose extinction and small bulges, indicative of bulging recrystallization (CitationStipp et al., 2002), are common in quartz. Calcite displays undulose extinction and mechanical e-twinning. Common mineral accessories in phyllite, metasandstone and calcschist (i-ii) consist of Tur, Py, Ap, Zrn, and Rt/Ttn. Barite and LREE (light rare earth elements)-phosphate are observed within phyllite and metasandstone. Pyrite grains commonly display euhedral habit and occur surrounded by displacement-controlled and face-controlled quartz fringes, elongated parallel to the S2 foliation (d).
Polymictic metabreccia (iii) consists of heterometric clasts of phyllite, quartzite and marble, flattened along the S2 foliation, embedded in a Qtz + Ab + Wmca + Chl + opaque + piemontite metapsammitic matrix. Clasts preserve internal structures, such as bedding planes, inherited from their sedimentary protolith. Competency contrast between strongly flattened calcite-rich clasts and quartz-rich clasts is common.
Monomictic metacarbonate breccia (iv) consists of strongly flattened and recrystallized carbonate clasts, oriented parallel to the S2 foliation, that are made up of fine-grained calcite-rich aggregates that are wrapped by a greenish Wmca + Chl + Cal + Fe-Ti oxide-bearing matrix.
Marble (v) shows fine-grained calcite grains with granoblastic to locally polygonal microstructure with a weak shape preferred orientation parallel to the S2 foliation and serrated grain boundaries. Mechanical e-twins occur within some grains. The S3 crenulation cleavage is well-developed only in rare white mica + chlorite-bearing lepidoblastic layers.
Metabasalt (vi) is characterized by a fine-grained Chl + Ca-Am + Wmca + Qtz + Ttn + Ep + Ilm + Mag assemblage. The earlier S1 cleavage is, seldom, preserved in some layers, marked by oriented Chl, Ca-Am and Wmca. The main foliation (S2) is defined by flattened quartz + calcite aggregates, interpreted as former magmatic vesicles, whereas the rock matrix display limited preferred orientation along the S2 foliation (e). Plagioclase phenocrysts, replaced by Ep + Chl + Wmca, and magmatic ophitic textures are still recognizable (f). Quartz grains show deformation bands, patchy undulose extinction and serrated grain boundaries with small bulges (g), indicative of bulging recrystallization (e.g. CitationStipp et al., 2002).
Quartz-rich metaconglomerate (vii) mainly consists of quartz polycrystalline clasts and minor tourmaline grains surrounded by a very fine-grained Qtz + Ser + Chl matrix. The S2 foliation is barely defined by oriented phyllosilicates. Quartz clasts show highly variable internal microstructures, ranging from polygonal to strongly lobate grain boundaries and grains with patchy to undulose extinction patterns and trails of quartz grains (h). Some clasts contain also cracks and healed fractures, as well as small bulges, indicative of bulging recrystallization (e.g. CitationStipp et al., 2002). We interpret the heterogeneous microstructures preserved within clasts as inherited from the protolith, only partially related to the Alpine deformation of the PBU.
7. Discussion and conclusion
Detailed structural geological mapping, coupled with microstructural observations, allowed defining the geometry and deformation pattern of the Punta Bianca Unit. The PBU represents an example of polyphase deformation that occurred during the evolution of an orogenic wedge, recording underplating and subsequent exhumation. In summary, the main structural features of the PBU are:
Presence of a relic earlier foliation (S1) defined by greenschist-facies paragenesis (e.g. Wmca + Chl + Ab + Ep)
Recumbent SW- and NE-verging F2 folds associated with a S2 foliation, marked by greenschist-facies paragenesis and flattened strain markers
Upright F3 folds that refold the S2 foliation and the tectonic contact with the Tuscan Nappe, associated with a steeply W-dipping S3 crenulation cleavage defined by opaque-rich surfaces. This phase is also associated with small scale thrusts and inversion of D2 boudins
Top-to-E/NE semibrittle shear zones (D4) that slice the Verrucano group over the older formations of the PBU
Late high-angle normal and transcurrent faults (D5), affecting the whole structural architecture
The D1 and D2 tectonic phases are linked to deformation in the middle crust, as testified by the associated greenschist-facies parageneses indicative of ∼300–400°C and P ∼0.4–0.7 GPa (CitationFranceschelli et al., 1986; CitationLo Pò et al., 2016; CitationMolli et al., 2018a, Citationb). D1 structures were strongly obliterated during the D2 phase and, therefore, it is not possible to constrain with precision their kinematics and geometry. According to CitationCarosi et al. (1991) and CitationStorti (1995), the D1 phase can be related to NE-verging underthrusting, based on a comparison with the nearby Alpi Apuane Unit and Massa Unit (CitationCarmignani & Kligfield, 1990; CitationMolli, 2008; CitationMolli et al., 2000, Citation2018a).
The overall geometry of D2 structures and the flattened finite strain ellipsoid are consistent with vertical shortening (e.g. CitationPlatt, 1986). Vertical shortening fits well with syn-orogenic extension, as also suggested by CitationStorti (1995). Therefore, we suggest that the D2 phase marks the main exhumation event in the area, although it is possible that exhumation started as early as during the D1 phase, as in the Alpi Apuane (e.g. CitationMolli et al., 2018a). W-verging structures, such as D2 folds and shear zones, might have formed during W-directed extension in the area, driving the exhumation to shallower levels within the orogenic wedge. Although no unambiguous kinematic indicators are preserved within the Brecce di Caprona Fm., these breccia likely marks a W-dipping and W-SW verging extensional structure probably reworking a former thrust fault, juxtaposing sub-greenschist-facies rocks of the TN (T ∼ 200°C; CitationMontomoli et al., 2001) in its hanging wall, from the greenschist-facies PBU.
Post-D2 phase deformation of the PBU occurred after its exhumation and coupling with the TN at shallow crustal levels. Indeed, (1) the coaxial involvement of the base of the Tuscan Nappe and the S2 foliation in large-scale F3 folds and (2) the presence of D4 shear zones affecting both units, suggest that the TN and the PBU were already coupled before D3–D4 deformation phases took place. Opaque-rich surfaces, marking the S3 foliation, represent stress-induced pressure solution surfaces (e.g. CitationGray & Durney, 1979a, Citationb; CitationEngelder & Marshak, 1985; CitationSchweitzer & Simpson, 1986) and are compatible with deformation at very-low metamorphic grade (e.g. CitationKanagawa, 1991; CitationKisch, 1991). Such conditions of deformation are comparable with the deformation mechanisms recognized in the Tuscan Nappe in the La Spezia area (CitationCarosi et al., 2003). Coupling of the TN with the underlying metamorphic units, suggested by the presence of clasts of metamorphic rocks in the tectonic breccia at the base of the TN (CitationCarosi et al., 2002, Citation2004) has been timely constrained at regional scale to the late Miocene-early Pliocene (CitationBalestrieri et al., 2011; CitationFellin et al., 2007). F3 folding is indicative of regional shortening and antiformal stacking (e.g. CitationMolli et al., 2018b), likely in association with out-of-sequence thrusting, as reported in other sectors of the Northern Apennines (CitationBonini & Sani, 2002; CitationClemenzi et al., 2014; CitationMassa et al., 2017; CitationMusumeci et al., 2008; CitationPapeschi et al., 2017). Out-of-sequence thrusting has also been proposed to explain the structures present in the offshore area of the La Spezia gulf (CitationAbbate et al., 2005). Consequently, D4 faults and shear zones may be alternatively interpreted as thrust fault flats, shallow ramps or as extensional detachments (as in the nearby Tellaro detachment; CitationClemenzi et al., 2015; CitationMolli et al., 2018a). In the former scenario, post-orogenic extension linked to the opening of the Northern Thyrrhenian Sea (e.g. CitationJolivet et al., 1998) would have been accommodated entirely by D5 high-angle normal faults in the area.
As a whole, the polyphase history recorded by the PBU, summarized in this study, highlights the complex tectonic history recorded during continental subduction and exhumation in the hinterland sector of the Northern Apennines. The new and revised structural geological map of the PBU constitute the basis for further studies in the area.
Software
The geological map and the tectonic sketch were produced using ESRI ArcGIS 10.0 and CorelDraw X4. The stereographic projections were realized using OpenStereo and Stereonet. Vector-based versions of the topographic maps were realized from the rasters of the Carta Tecnica Regionale – Regione Liguria (www.regione.liguria.it).
PIMR1_Geological_Map.pdf
Download PDF (4.7 MB)Acknowledgements
We thank Giovanni Raggi and Stefano Palandri for assistance during fieldwork and Daniele Nannini for the technical support during digitalization of the geological map. We are grateful to Maria Di Rosa, Michele Marroni, and Thomas Pingel for their constructive reviews and to Arthur Merschat for editorial handling.
Supplementary material
Geographic coordinated and details of the samples selected for this study are available on SESAR (www.geosamples.org). The finite strain analysis dataset, including high-resolution scans of polished slabs, tables and finite strain data, is available for download at https://doi.org/10.17632/hyxw2shy3z.1.
Disclosure statement
No potential conflict of interest was reported by the authors.
Additional information
Funding
References
- Abbate, E., Fanucci, F., Benvenuti, M., Bruni, P., Cipriani, N., Falorni, P., … Sagri, M. (2005). Note illustrative della Carta Geologica d’italia alla scala 1: 50.000-Foglio 248-La Spezia, 1-204. Agenzia per la Protezione dell’Ambiente e per i Servizi Tecnici, Dipartimento Difesa del Suolo. S.EL.CA srl.
- Baldacci, F., Elter, P., Giannini, E., Giglia, G., Lazzarotto, A., Nardi, R., & Tongiorgi, M. (1967). Nuove osservazioni sul problema della Falda Toscana e sulla interpretazione dei flysch arenacei tipo “macigno” dell’Appennino settentrionale. Memorie Della Società Geologica Italiana, 6(2), 213–244. https://pascal-francis.inist.fr/vibad/index.php?action=getRecordDetail&idt=GEODEBRGM6813007370
- Balestrieri, M. L., Pandeli, E., Bigazzi, G., Carosi, R., & Montomoli, C. (2011). Age and temperature constraints on metamorphism and exhumation of the syn-orogenic metamorphic complexes of Northern Apennines, Italy. Tectonophysics, 509(3-4), 254–271. https://doi.org/10.1016/j.tecto.2011.06.015
- Boccaletti, M., Elter, P., & Guazzone, G. (1971). Plate tectonic models for the development of the Western Alps and Northern Apennines. Nature Physical Science, 234(49), 108. https://doi.org/10.1038/physci234108a0
- Bonini, M., & Sani, F. (2002). Extension and compression in the Northern Apennines (Italy) hinterland: Evidence from the late Miocene–Pliocene Siena–Radicofani Basin and relations with basement structures. Tectonics, 21(3), 3. https://doi.org/10.1029/2001TC900024
- Burckhardt, C. E. (1947). Il sondaggio del Belagajo (Grosseto) ed il suo significato geologico. Bollettino Della Società Geologica Italiana, 65, 97–107.
- Carmignani, L., & Kligfield, R. (1990). Crustal extension in the Northern Apennines: The transition from compression to extension in the Alpi Apuane core complex. Tectonics, 9(6), 1275–1303. https://doi.org/10.1029/TC009i006p01275
- Carmignani, L., Giglia, G., & Kligfield, R. (1978). Structural evolution of the Apuane Alps: An example of continental margin deformation in the Northern Apennines, Italy. The Journal of Geology, 86(4), 487–504. https://doi.org/10.1086/649714
- Carmignani, L., Decandia, F. A., Disperati, L., Fantozzi, P. L., Kligfield, R., Lazzarotto, A., … Meccheri, M. (2001). Inner Northern Apennines. In F. Vai, & I. P. Martini (Eds.), Anatomy of an Orogen: The Apennines and adjacent Mediterranean Basins (pp. 197–213). Springer.
- Carosi, R., Marroni, M., & Storti, F., (1991). Structural setting of Punta Bianca area (Northern Apennines, Italy). Abstract del Convegno di Geologia del basamento Italiano, Siena, 21–22 Marzo 1991, 127–129.
- Carosi, R., Montomoli, C., Bertuccelli, N., & Profeti, M. (2002). The structural evolution of the southern Apuan Alps: New constraints on the tectonic evolution of the Northern Apennines (Italy). Comptes Rendus Geoscience, 334(5), 339–346. https://doi.org/10.1016/S1631-0713(02)01741-8
- Carosi, R., Leoni, L., Montomoli, C., & Sartori, F. (2003). Very low-grade metamorphism in the Tuscan Nappe, Northern Apennines, Italy: Relationships between deformation and metamorphic indicators in the La Spezia mega-fold. Swiss Bulletin of Mineralogy and Petrology, 83(1), 15–32. https://doi.org/0036-7699/2003/83/15
- Carosi, R., Montomoli, C., & Pertusati, P. C. (2004). Late tectonic evolution of the Northern Apennines: The role of contractional tectonics in the exhumation of the Tuscan units. Geodinamica Acta, 17(4), 253–273. https://doi.org/10.3166/ga.17.253-273
- Carosi, R., Pertusati, P. C., Montomoli, C. (2018). Foglio 273 “Pisa” della Carta Geologica d’Italia in scala 1:50.00. Istituto Superiore per la Protezione e Ricerca Ambientale ISPRA. https://www.isprambiente.gov.it/Media/carg/273_PISA/Foglio.html
- Carter, K. E. (1992). Evolution of stacked, ductile shear zones in carbonates from mid-crustal levels: Tuscan Nappe, N. Apennines, Italy. Journal of Structural Geology, 14(2), 181–192. https://doi.org/10.1016/0191-8141(92)90055-2
- Cassinis, G., Perotti, C., & Santi, G. (2018). Post-Variscan Verrucano-like deposits in Italy, and the onset of the alpine tectono-sedimentary cycle. Earth-Science Reviews, 185, 476–497. https://doi.org/10.1016/j.earscirev.2018.06.021
- Ciarapica, G., & Passeri, L. (2005). Late Triassic and Early Jurassic sedimentary evolution of the Northern Apennines; an overview. Bollettino Della Società Geologica Italiana, 124(1), 189–201.
- Clemenzi, L., Molli, G., Storti, F., Muchez, P., Swennen, R., & Torelli, L. (2014). Extensional deformation structures within a convergent orogen: The Val di Lima low-angle normal fault system (Northern Apennines, Italy). Journal of Structural Geology, 66, 205–222. https://doi.org/10.1016/j.jsg.2014.05.019
- Clemenzi, L., Storti, F., Balsamo, F., Molli, G., Ellam, R., Muchez, P., & Swennen, R. (2015). Fluid pressure cycles, variations in permeability, and weakening mechanisms along low-angle normal faults: The Tellaro detachment, Italy. Geological Society of America Bulletin, 127(11-12), 1689–1710. https://doi.org/10.1130/B31203.1
- Dahlen, F. A. (1990). Critical taper model of fold-and-thrust belts and accretionary wedges. Annual Review of Earth and Planetary Sciences, 18(1), 55–99. https://doi.org/10.1146/annurev.ea.18.050190.000415
- Davis, D., Suppe, J., & Dahlen, F. A. (1983). Mechanics of fold-and-thrust belts and accretionary wedges. Journal of Geophysical Research: Solid Earth, 88(B2), 1153–1172. https://doi.org/10.1029/JB088iB02p01153
- Di Pisa, A., Franceschelli, M., Leoni, L., & Meccheri, M. (1985). Regional variation of the metamorphic temperatures across the Tuscanid I Unit and its implications on the alpine metamorphism (Apuan Alps, N Tuscany). Neues Jahrbuch fur Mineralogie-Abhandlungen, 151, 197–211.
- Dunnet, D. (1969). A technique of finite strain analysis using elliptical particles. Tectonophysics, 7(2), 117–136. https://doi.org/10.1016/0040-1951(69)90002-X
- Elter, P. (1960). I lineamenti tettonici dell’Appennino a Nord Ovest delle Apuane. Bollettino Della Società Geologica Italiana, 79(2), 273–312.
- Elter, P. (1975). Introduction à la géologie de l'Apennin septentrional. Bulletin de la Société Géologique de France, 7(6), 956–962. https://doi.org/10.2113/gssgfbull.S7-XVII.6.956
- Engelder, T., & Marshak, S. (1985). Disjunctive cleavage formed at shallow depths in sedimentary rocks. Journal of Structural Geology, 7(3-4), 327–343. https://doi.org/10.1016/0191-8141(85)90039-2
- Federici, P. R., & Raggi, G. (1975). Una nuova interpretazione della tettonica dei monti della Spezia. Bollettino Della Società Geologica Italiana, 94(4), 945–960.
- Fellin, M. G., Reiners, P. W., Brandon, M. T., Wüthrich, E., Balestrieri, M. L., & Molli, G. (2007). Thermochronologic evidence for the exhumational history of the Alpi Apuane metamorphic core complex, northern Apennines, Italy. Tectonics, 26(6), 6. https://doi.org/10.1029/2006TC002085
- Franceschelli, M., Leoni, L., Memmi, I., & Puxeddu, M. (1986). Regional distribution of Al-silicates and metamorphic zonation in the low-grade Verrucano metasediments from the Northern Apennines, Italy. Journal of Metamorphic Geology, 4(3), 309–321. https://doi.org/10.1111/j.1525-1314.1986.tb00353.x
- Franceschelli, M., Gianelli, G., Pandeli, E., & Puxeddu, M. (2004). Variscan and Alpine metamorphic events in the northern Apennines (Italy): a review. Periodico di Mineralogia, 73(2), 43–56.
- Gandin, A., Giamello, M., Guasparri, G., Mugnaini, S., & Sabatini, G. (2000). The calcare cavernoso of the Montagnola Senese (Siena, Italy): mineralogical-petrographic and petrogenetic features. Mineralogica et Petrographica Acta, 43, 271–289.
- Gianmarino, S., & Giglia, G. (1990). Gli elementi strutturali della piega di La Spezia nel contesto geodinamico dell'Appennino settentrionale. Bollettino Della Società Geologica Italiana, 109(4), 683–692.
- Giorgetti, G., Goffe, B., Memmi, I., & Nieto, F. (1998). Metamorphic evolution of Verrucano metasediments in Northern Apennines; new petrological constraints. European Journal of Mineralogy, 10(6), 1295–1308. https://doi.org/10.1127/ejm/10/6/1295
- Gray, D. R., & Durney, D. W. (1979a). Crenulation cleavage differentiation: Implications of solution-deposition processes. Journal of Structural Geology, 1(1), 73–80. https://doi.org/10.1016/0191-8141(79)90023-3
- Gray, D. R., & Durney, D. W. (1979b). Investigations on the mechanical significance of crenulation cleavage. Tectonophysics, 58(1-2), 35–79. https://doi.org/10.1016/0040-1951(79)90321-4
- Grujic, D., Casey, M., Davidson, C., Hollister, L. S., Kündig, R., Pavlis, T., & Schmid, S. (1996). Ductile extrusion of the Higher Himalayan Crystalline in Bhutan: Evidence from quartz microfabrics. Tectonophysics, 260(1-3), 21–43. https://doi.org/10.1016/0040-1951(96)00074-1
- Jolivet, L., Faccenna, C., Goffé, B., Mattei, M., Rossetti, F., Brunet, C., … Parra, T. (1998). Midcrustal shear zones in postorogenic extension: Example from the northern Tyrrhenian Sea. Journal of Geophysical Research: Solid Earth, 103(B6), 12123–12160. https://doi.org/10.1029/97JB03616
- Kanagawa, K. (1991). Change in dominant mechanisms for phyllosilicate preferred orientation during cleavage development in the Kitakami slates of NE Japan. Journal of Structural Geology, 13(8), 927–943. https://doi.org/10.1016/0191-8141(91)90088-Z
- Kearey, P., Klepeis, K. A., & Vine, F. J. (2009). Global tectonics. John Wiley & Sons.
- Kisch, H. J. (1991). Development of slaty cleavage and degree of very-low-grade metamorphism: A review. Journal of Metamorphic Geology, 9(6), 735–750. https://doi.org/10.1111/j.1525-1314.1991.tb00562.x
- Konstantinovskaya, E., & Malavieille, J. (2011). Thrust wedges with décollement levels and syntectonic erosion: A view from analog models. Tectonophysics, 502(3-4), 336–350. https://doi.org/10.1016/j.tecto.2011.01.020
- Law, R. D., Searle, M. P., & Simpson, R. L. (2004). Strain, deformation temperatures and vorticity of flow at the top of the Greater Himalayan Slab, Everest Massif, Tibet. Journal of the Geological Society, 161(2), 305–320. https://doi.org/10.1144/0016-764903-047
- Lo Pò, D., & Braga, R. (2014). Influence of ferric iron on phase equilibria in greenschist facies assemblages: The hematite-rich metasedimentary rocks from the Monti Pisani (Northern Apennines). Journal of Metamorphic Geology, 32(4), 371–387. https://doi.org/10.1111/jmg.12076
- Lo Pò, D., Braga, R., & & Massonne, H. J. (2016). Petrographic, mineral and pressure-temperature constraints on phyllites from the Variscan basement at Punta Bianca, Northern Apennines, Italy. Italian Journal of Geosciences, 135(3), 489–502. https://doi.org/10.3301/IJG.2015.29
- Logan, J. M., Dengo, C. A., Higgs, N. G., & Wang, Z. Z. (1992). Fabrics of experimental fault zones: Their development and relationship to mechanical behavior. In A. Festschrift (Ed.), Fault Mechanics and Transport Properties of Rocks. International geophysics (Vol. 51, pp. 33–67). Academic Press.
- Marroni, M., Molli, G., Ottria, G., & Pandolfi, L. (2001). Tectono-sedimentary evolution of the External Liguride units (Northern Apennines, Italy): Insights in the pre-collisional history of a fossil ocean-continent transition zone. Geodinamica Acta, 14(5), 307–320. https://doi.org/10.1080/09853111.2001.11432449
- Martini, I. P., Rau, A., & Tongiorgi, M. (1986). Syntectonic sedimentation in a middle Triassic rift, northern Apennines, Italy. Sedimentary Geology, 47(3-4), 191–219. https://doi.org/10.1016/0037-0738(86)90084-9
- Massa, G., Musumeci, G., Mazzarini, F., & Pieruccioni, D. (2017). Coexistence of contractional and extensional tectonics during the northern Apennines orogeny: The late Miocene out-of sequence thrust in the Elba Island nappe stack. Geological Journal, 52(3), 353–368. https://doi.org/10.1002/gj.2761
- Molli, G. (2008). Northern Apennine–Corsica orogenic system: An updated overview. Geological Society, London, Special Publications, 298(1), 413–442.
- Molli, G., Giorgetti, G., & Meccheri, M. (2000). Structural and petrological constraints on the tectono-metamorphic evolution of the Massa Unit (Alpi Apuane, NW Tuscany, Italy). Geological Journal, 35(3-4), 251–264. https://doi.org/10.1002/gj.860
- Molli, G., White, J. C., Kennedy, L., & Taini, V. (2011). Low-temperature deformation of limestone, Isola Palmaria, northern Apennine, Italy–the role of primary textures, precursory veins and intracrystalline deformation in localization. Journal of Structural Geology, 33(3), 255–270. https://doi.org/10.1016/j.jsg.2010.11.015
- Molli, G., Carlini, M., Vescovi, P., Artoni, A., Balsamo, F., Camurri, F., … Torelli, L. (2018a). Neogene 3D structural architecture of The North-West Apennines: The role of the low-angle normal faults and basement thrusts. Tectonics, 37(7), 2165–2196. https://doi.org/10.1029/2018TC005057
- Molli, G., Vitale Brovarone, A., Beyssac, O., & Cinquini, I. (2018b). RSCM thermometry in the Alpi Apuane (NW Tuscany, Italy): New constraints for the metamorphic and tectonic history of the inner northern Apennines. Journal of Structural Geology, 113, 200–216. https://doi.org/10.1016/j.jsg.2018.05.020
- Montomoli, C. (2002). Vein development and fluid inclusion data: Insight on the evolution of the Tuscan Nappe in the Northern Apennines. Bollettino Della Società Geologica Italiana, 1(2), 801–817.
- Montomoli, C., Ruggieri, G., Boiron, M. C., & Cathelineau, M. (2001). Pressure fluctuation during uplift of the Northern Apennines (Italy): a fluid inclusions study. Tectonophysics, 341(1-4), 121–139. https://doi.org/10.1016/S0040-1951(01)00197-4
- Musumeci, G., Mazzarini, F., & Barsella, M. (2008). Pliocene crustal shortening on the Tyrrhenian side of the northern Apennines: Evidence from the Gavorrano antiform (southern Tuscany, Italy). Journal of the Geological Society, 165(1), 105–114. https://doi.org/10.1144/0016-76492007-004
- Papeschi, S., Musumeci, G., & Mazzarini, F. (2017). Heterogeneous brittle-ductile deformation at shallow crustal levels under high thermal conditions: The case of a synkinematic contact aureole in the inner northern Apennines, southeastern Elba Island, Italy. Tectonophysics, 717, 547–564. https://doi.org/10.1016/j.tecto.2017.08.020
- Papeschi, S., Musumeci, G., Massonne, H. J., Mazzarini, F., Ryan, E., & Viola, G. (2020). High–pressure (P = 1.5–1.8 GPa) blueschist from Elba: Implications for underthrusting and exhumation of continental units in the Northern Apennines. Journal of Metamorphic Geology, https://doi.org/10.1111/jmg.12530
- Passchier, C. W., & Trouw, R. A. (2005). Microtectonics (Vol. 1). Springer Science & Business Media.
- Platt, J. P. (1986). Dynamics of orogenic wedges and the uplift of high-pressure metamorphic rocks. Geological Society of America Bulletin, 97(9), 1037–1053. https://doi.org/10.1130/0016-7606(1986)97<1037:DOOWAT>2.0.CO;2
- Ramsay, J. G., & Huber, M. I. (1987). The techniques of modern structural geology (Vol. 2). Academic Press.
- Rau, A., Tongiorgi, M., & Martini, I. P. (1985). La successione di Punta Bianca: Un esempio di rift ‘abortivo’ nel Trias Medio del Dominio Toscano. Memorie Della Società Geologica Italiana, 30, 115–125.
- Ricci, C. A., & Serri, G. (1975). Evidenze geochimiche sulla diversa affinità petrogenetica delle rocce basiche comprese nelle serie a facies Toscana. Bollettino Della Società Geologica Italiana, 94(5), 1187–1198.
- Ring, U., & Glodny, J. (2010). No need for lithospheric extension for exhuming (U) HP rocks by normal faulting. Journal of the Geological Society, 167(2), 225–228. https://doi.org/10.1144/0016-76492009-134
- Saffer, D. M., & Bekins, B. A. (2002). Hydrologic controls on the morphology and mechanics of accretionary wedges. Geology, 30(3), 271–274. https://doi.org/10.1130/0091-7613(2002)030<0271:HCOTMA>2.0.CO;2
- Schweitzer, J., & Simpson, C. (1986). Cleavage development in dolomite of the Elbrook formation, southwest Virginia. Geological Society of America Bulletin, 97(6), 778–786. https://doi.org/10.1130/0016-7606(1986)97<778:CDIDOT>2.0.CO;2
- Siivola, J., & Schmid, R. (2007). List of mineral abbreviations. In D. Fettes, & J. Desmons (Eds.), Metamorphic rocks: A classification and Glossary of Terms. Recommendations of the International Union of geological Sciences Subcommission on the Systematics of metamorphic rocks (pp. 93–110). Cambridge University Press.
- Stern, R. J. (2002). Subduction zones. Reviews of Geophysics, 40(4), 3–1. https://doi.org/10.1029/2001RG000108
- Stipp, M., Stünitz, H., Heilbronner, R., & Schmid, S. M. (2002). The eastern Tonale fault zone: A ‘natural laboratory’ for crystal plastic deformation of quartz over a temperature range from 250 to 700 C. Journal of Structural Geology, 24(12), 1861–1884. https://doi.org/10.1016/S0191-8141(02)00035-4
- Stoppa, F. (1985). Problematiche petrologiche e geologiche delle prasiniti di Punta Bianca (La Spezia). Memorie Della Società Geologica Italiana, 30, 127–134.
- Storti, F. (1995). Tectonics of the Punta Bianca promontory: Insights for the evolution of the Northern Apennines-Northern Tyrrhenian Sea basin. Tectonics, 14(4), 832–847. https://doi.org/10.1029/95TC01203
- Theye, T., Reinhardt, J., Goffé, B., Jolivet, L., & Brunet, C. (1997). Ferro-and magnesiocarpholite from the Monte Argentario (Italy): first evidence for high-pressure metamorphism of the metasedimentary Verrucano sequence, and significance for PT path reconstruction. European Journal of Mineralogy, 9(4), 859–874. https://doi.org/10.1127/ejm/9/4/0859
- Vighi, L. (1958). Sulla serie triassica “Cavernoso-Verrucano” presso Capalbio (Orbetello, Toscana) e sulla brecciatura tettonica delle serie evaporitiche “rocce madri” del Cavernoso. Bollettino Della Società Geologica Italiana, 77, 221–235.