ABSTRACT
This paper presents the geomorphological mapping at a 1:100,000 scale of fluvial deposits in the middle Tocantins River. The region preserves an important sedimentary archive of environmental changes of the Amazon and Cerrado biomes. Yet, the region is under influence of diverse anthropogenic activities, including planned or operational hydropower plants, and detailed geomorphological mapping is lacking. The mapping combined interpretation of surface geomorphic features, morphometric analysis, and field surveys. Three main geomorphic units were defined: (i) fluvial plain, (ii) fluvial terraces, and (iii) paleo-alluvial fans. The detailed mapping survey allowed a hierarchical organization of geomorphological units as well as their relative chronology of formation. Our results improve the understanding on the complex geomorphological processes which shape the current fluvial landscape. Specifically, improving the understanding of the Tocantins River floodplains is crucial to support conservation of flooded forests, sustainable use of natural resources and minimize socio-economic losses and damages.
1. Introduction
Geomorphological mapping of floodplains, terraces and their associated sub-environments provides information for understanding the functioning of river systems (CitationBrierley & Fryirs, 2005; CitationLewin et al., 1977; CitationNanson & Croke, 1992), which is critical to decisions in territorial planning, flood risk assessment, surface water management and river restoration programs (CitationFryirs & Brierley, 2012). Additionally, floodplains of large tropical rivers host unique biodiversity adapted to flooding (e.g. CitationThom et al., 2020) and the detailed mapping of landforms and surface fluvial deposits is important to predict future landscape changes with implications for biodiversity conservation (e.g. CitationHamilton et al., 2007). These studies have been developed under a perspective of process-form relationships to support the interpretation of ancient fluvial sequences (CitationSinha et al., 2005).
Historically, studies about river terrace landform sequences significantly contributed to understand Quaternary landscape changes (CitationAntoine et al., 2007; CitationBridgland & Westaway, 2014; CitationRossetti et al., 2005). Detailed geomorphological maps are important components of such fluvial systems studies because they allow to connect surface landforms to fluvial processes (CitationMiklín & Galia, 2017).
The detailed characterization of the fluvial systems in the Amazon is still a hard task due to the large extension of the area and ubiquitous forest vegetation cover, but the remote sensing data has allowed to improve the knowledge on the alluvial deposits (e.g. CitationHayakawa et al., 2010; CitationPassos et al., 2020). Currently, easy access to emerging remote sensing methods and tools such as high-resolution spatial data such as aerial and satellite imagery or digital elevation models provides enormous opportunities to produce more accurate and detailed geomorphological maps (CitationBangen et al., 2014; CitationJones et al., 2007; CitationWilliams et al., 2014).
The Tocantins River and its main tributary, the Araguaia River, are among the largest rivers in eastern Amazon () and they stand out due to host areas with significant accumulation of Quaternary sediments (CitationMMA, 2006). The geomorphological history of the Tocantins River, in turn, is mostly associated with the geological evolution of the Brazilian Shield (CitationLima & Caires, 2011; CitationLima & Ribeiro, 2011). The middle stretch of the Tocantins River consists of wide floodplains and fluvial terraces with numerous lakes and wetlands that provide diverse wildlife habitats and important ecological services (CitationMerona et al., 2010) and due to its evolutionary history among Amazonian tributaries (CitationAkama, 2017), has one of the highest endemicity and species richness rates (CitationDagosta & Pinna, 2019).
Figure 1. Geological setting of the middle Tocantins River, including the study area. (A) Main geological units of the area include high-grade metamorphic rocks of the Cajazeiras Complex, low-grade metamorphic rocks of the Couto Magalhães Formation, sedimentary deposits of the Itapecuru Group and Codó Formation (CitationVasquez & Rosa-Costa, 2008). (B) Location of the study area near the boundary between the Amazon and Cerrado biomes.
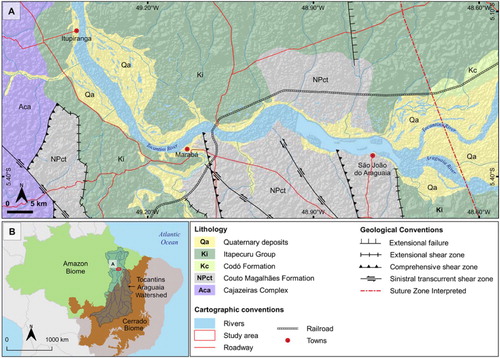
The middle Tocantins River also includes Marabá city, one of the major cities of the eastern Amazon, and extensive agriculture areas in wetlands experiencing seasonal flooding, which causes recurrent socio-economic damages (CitationDeus et al., 2018).
Despite the ecological and socio-economic relevance of the middle Tocantins River and its floodplains, there are few studies available for planning of conservation initiatives and there are still enormous gaps on detailed spatially-resolved geomorphological information about this area. Recent studies described the geomorphological aspects of the middle Tocantins River (CitationFelipe & Morales, 2017; CitationMascarenhas et al., 2013), but only on a regional scale, classifying the terrains as depositional, dissected and surface planation.
In this context, this study aims to produce a detailed geomorphological map (1:100,000) of the middle Tocantins River in order to improve the understanding of the link between surface landforms and fluvial processes. Therefore, the geomorphological mapping of the middle Tocantins River was carried out through field surveys combined with remote sensing and geographical information system (GIS) methods. The results are discussed to constrain landform changes in terms of fluvial processes operating in large tropical rivers. Moreover, our results also have potential to support decision makers in conservation programs in a region under constant threat. The possibility of installing a large hydroelectric plant in the Tocantins River near the Marabá city (CitationBrasil, 2012) reinforces the importance of this study because of predicted reduction of floodplains and unknown threats to the aquatic environments (CitationAkama, 2017).
2. Physical setting
The Tocantins River watershed extends from 46° to 55° west and from 2° to 18° south. It has a drainage area of 306,310 km², upstream from the confluence with the Araguaia River and a total area of 764,996 km², including the drainage area of the Araguaia River (; CitationANA, 2017). The Tocantins River drains distinct biomes, which are mainly represented by savannas (Cerrado) of the Central Plateau of Brazil, Amazon rainforest and a transition zone between both vegetation biomes ((B); CitationOliveira-Filho, 1995).
The study area comprises a wide (20 km × 80 km) fluvial plain located downstream of the confluence of the Araguaia and Tocantins Rivers, until the Itupiranga city (). This region is locally named ‘Bico do Papagaio’ (Parrot beak; eastern area) and ‘Paleocanal do Tocantins’ (Tocantins Paleochannel; western area), which is characterized by a low-altitude (< 110m) and low relief terrains developed in fluvial sediments deposited during the Quaternary (; CitationRadamBrasil, 1986). These deposits are incised by the Tocantins River that flow over metamorphic rocks and alluvial beds. CitationVasquez and Rosa-Costa (2008) proposed two units for these Quaternary deposits: (1) fluvial terraces: composed of unconsolidated and consolidated clay, sand and gravel, topographically higher than the current alluvial plain; and (2) alluvial deposits: composed of grey clay and sand corresponding to channel and floodplain deposits related to the current alluvial plain. Upstream of the Marabá City, the riverbed is dominated by rapids and rocky islands that expose low-grade metamorphic rocks related to the Araguaia Fold Belt (CitationHerz et al., 1989). Downstream, the channel of the Tocantins River is dominated by fluvial bars and forms a wide floodplain with lakes, paleo-channels and swamps (CitationRibeiro et al., 1995).
Regional geology is represented by four distinct geological units ((A)): Archean high-grade metamorphic rocks of the Cajazeira Complex (CitationBrito Neves & Cordani, 1991); Neoproterozoic low-grade metamorphic rocks of the Couto Magalhães Formation (CitationAlmeida et al., 1977); Cretaceous sedimentary rocks related with the Itapecuru Group (CitationRossetti & Truckenbrodt, 1997) and Codó Formation (CitationMesner & Wooldridge, 1964) of the Marajó Basin (CitationCosta et al., 2002; CitationVillegas, 1994) and; sandstones from Parnaíba Basin (CitationBigarella, 1973; CitationGóes, 1995).
The rainfall in the eastern Amazon is concentrated during the austral summer and autumn due to the activity of the South American Summer Monsoon (SASM, CitationZhou & Lau, 1998) and the Intertropical Convergence Zone (ITCZ, CitationGarreaud et al., 2009; CitationMarengo & Espinoza, 2016), which southward shift during the austral summer bring moisture from the equatorial Atlantic to inland areas. The climate across the Tocantins watershed is tropical, with an annual average temperature of 26° C and two well-defined climatic periods: the rainy, October to April, with more than 90% of precipitation, and the dry one, from May to September, with low relative humidity (CitationMMA, 2006). The average rainfall in the watershed is around 1869 mm yr−1 (ANA, Citation2002), but can reach up to 2565 mm near the river mouth on the estuary (CitationANA, 2005), and the average evapotranspiration is 1371 mm yr−1.
3. Methods and data
3.1. Geomorphological mapping and classification
Identification and mapping of surface landforms were based on visual interpretation of optical and radar satellite images from different sensors and resolutions based in a constructivist (building block) approach to reading the landscape (CitationFryirs & Brierley, 2012). To standardize the mapping on the scale of 1:100,000, we used Tobler’s rule (CitationTobler, 1987). Although remotely sensed or modeled data provide critical guidance in our efforts to interpret landscapes, it is highlighted that robust validation of surface features identified through remote sensing methods is derived from field-based analyses (CitationFryirs & Brierley, 2012).
The criteria for image interpretation and terminology of fluvial landforms were applied as suggested by CitationWheaton et al. (2015), CitationFryirs and Brierley (2012), John CitationLewin and Ashworth (2014) and CitationAkter et al. (2018). Geomorphic units were defined by analysis of the drainage networks, erosive and depositional features, and visual interpretation of satellite images. The geomorphological classification was based on the hierarchical system proposed by Zinck (Citation2016), which is constructed under the basic criteria of configuration, composition and hierarchical organization that reflects the level of the geomorphological units in the landscape.
3.2. Remote sensing
3.2.1. Image processing
We use Landsat-8 images, Path 223 / Row 64, dated of on May 29th, 2018. Images were obtained from NASA’s repository. Images resulting from the fusion of multispectral image (Red – B4 640–690 nm; Green – B3 530–590 nm; Blue – B2 450–510 nm) with medium spatial resolution (30 m) and Panchromatic image (B8 500–680 nm) with higher spatial resolution (15 m), using HIS transformation, were particularly useful in obtaining a better contrast and enhancement of fluvial features. High resolution imagery from the Google Earth Pro© (CitationGoogle Earth Pro©, 2019) application and Esri World Imagery (CitationEsri, 2019) were used to improve the remote sensing interpretation of smaller geomorphic features (e.g. paleo-channels, fluvial bars and small lakes). The Multi-Error Removed Improved-Terrain Digital Elevation Model (MERIT DEM), with 3 arc second resolution (∼90 m at the equator), was used for identification and mapping of landforms, morphometric analysis, and elaboration of topographic profiles. The MERIT DEM was developed by processing existing spaceborne DEMs, and it represents elevation in meter, referenced to WGS84 and EGM96 (MERIT DEM accessed on January 6th, 2019; CitationYamazaki et al., 2017). Processing techniques were applied to enhance landforms of low topographic amplitude, following CitationMerino et al. (2015). The color palettes were customized with local elevation intervals between 3 and 15 m. Image processing techniques and the map design were performed in the software QGIS® (Version 2.18.19, QGIS Development Team, 2019). The graphic adjustments, as color enhancement and brightness, were made in the software Inkscape™ 0.92 (CitationInkscape, 2007) and the fusion of the optical images by the Spectral Transformer GUI Landsat-8 Imagery® (CitationGeosage, 2018). The cross-section topographic profile was performed using the ‘Profile Tool’ plugin in QGIS and exported to Inkscape™ to add lithological information and perform graphical adjustments.
3.2.2. Morphometric classification and topographic profiles
Morphometric characterization and topographic profiles were derived from MERIT DEM. The determination of the relative chronology of mapping units was performed through the analysis of morphological and topographic attributes, such as the presence of active or paleo-channels and lakes, preservation degree of forms, forms superposition and truncations and topographic level of terraces surfaces (e.g. CitationPupim et al., 2017; CitationVerstappen, 1977). In general, higher topographic levels are older than lower levels, as well as more preserved morphological features are newer than more degraded features (CitationPazzaglia, 2013).
3.3. Field surveys
Field surveys were performed to verify and improve the geomorphic interpretation through satellite images and aerial photographs. Fieldworks were carried out in September of 2018 and comprised the description of sedimentary deposits in outcrops and trenches using a facies analysis approach according to CitationMiall (2014).
4. Description of the geomorphological map units
The presented geomorphological map integrates erosive and depositional landforms observed in the middle Tocantins River, at a 1:100,000 scale. The mapping units are organized into three categories based on interpreted active and paleo depositional environments: (i) fluvial plain; (ii) fluvial terraces (T1, T2) and (iii) paleo-alluvial fan (Main Map – Appendices). The distribution of these geomorphological mapping units is restricted to the Tocantins River valley ( and ).
Figure 2. Geomorphological aspects of the middle Tocantins River: (A) Landsat-8 image true-color (September 2018); (B) MERIT digital elevation model; (C) Main landforms mapped that represents the relief/molding categorical levels.
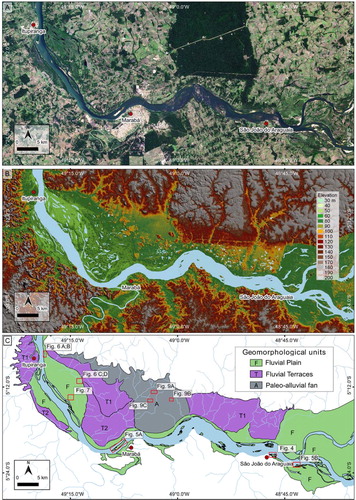
4.1. Geomorphological units
4.2. Fluvial plain
4.2.1. Channel
The studied reach of the Tocantins River comprises a mixed bedrock-alluvial channel. The main channel is cutting into bedrock substrates in the upstream reach (), with rapids and some lateral sediment bars ((B)). The river style changes to a predominately sand-rich channel and wide floodplain from Marabá to Itupiranga cities ((A)). The smallest geomorphic units recognized within the channel are longitudinal and lateral bars. Longitudinal bars are elongated sand bodies in the direction of the water flow and with and/or without vegetation cover. The deposits are predominately sandy with decimetric sets of crossbedding, cross-lamination, and levels of well-selected and sub-rounded granules ((A)). Vegetated bars associated with longitudinal bars, have accumulation of deposition build up leading to a relatively stable and high-altitude landform suitable for vegetation growth. This condition keeps the surface free of the inundation for long periods and supports the development of forest vegetation ((A)). Lateral bars are sand bodies attached to rocky outcrops in the riverbed that consist in obstacles to accumulation of sediments. Vegetation cover is incipient but can occur in the wider bars ((B)). The bars are composed of gravel in the bottom part and coarse sand in the upper portion whose morphology is controlled by joints, bedding and outcrops of slates, shales and phyllites (). This structural control is prominent in the upper reach of the mapping area, leading the development of irregularities in the channel substrate and the formation potholes, steps, and pools.
Figure 4. Bedrock rapids upstream middle Tocantins River. Digital Globe, GeoEye, CNES/Airbus DS. Change updated: August 8, 2019 to Accessed: August 8, 2019. Location in .
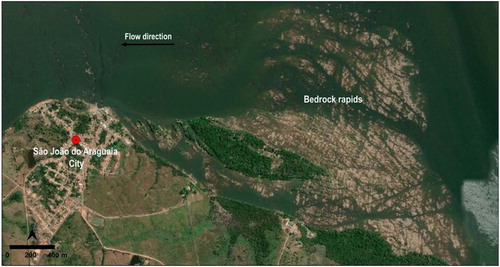
Figure 5. Geomorphic features within the channel unit. Location of photos and images in (C): (A) Longitudinal bar attached in vegetated bar; (B) Lateral bar with vegetated and non-vegetated. Digital Globe, GeoEye, CNES/Airbus DS Change updated: August 8, 2019 to Accessed: August 8, 2019. Location in .
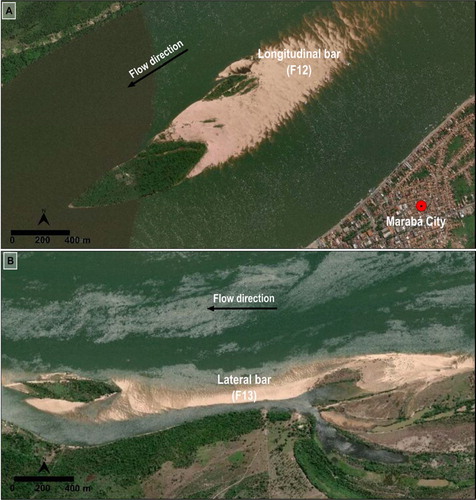
4.2.2. Floodplain
Floodplains were recognized in both margins of the Tocantins River, but the plain of the right margin is wider and more complex in terms of landforms, with the presence of numerous lakes, paleo-channels, swamp areas, levees, and crevasse splays. The terrain is almost flat, with smooth local relief in which the highest elevations vary from 84 to 79 m.a.s.l. (meters above sea level based on MERIT DEM data) and the lowest from 78 to 66 m.a.s.l. The floodplain areas have deposits of mud and fine sand due to seasonal inundation. The fluvial plain was divided in proximal floodplain and distal floodplain considering the presence of morphological elements as scroll bars, lakes, and paleo-channels. Lower level units were also mapped, reflecting the complexity of this environment.
Distal floodplain is representing the lowest area of this geomorphic unit, with elevation ranging from 66 to 78 m.a.s.l. The surface sediments are dominated by clay and silt and the sedimentation occurs during high magnitude floods that reach the distal parts of the plain. Perennial lakes, paleo-channels and ridge and swale are diagnostic features of this geomorphic unit, but they also occur in proximal floodplains and younger terraces. Perennial lakes are circular or semi-circular forms permanently flooded. Perennial lakes have been described as ‘rounded lakes’ which are particularly important in geomorphic mapping, because they are the major ‘sink of sediment’ in the large fluvial systems of South America (CitationDunne et al., 1998; CitationMertes et al., 1996). Paleo-channels are elongated features corresponding to abandoned channels ((C and D)). The paleo-channels are commonly formed through channel avulsion that promotes the development of perennial or ephemeral lakes (CitationSlingerland & Smith, 2004). They are filled with fine-grained sediments (silt and clay) and organic matter supplied by the channel and nearby floodplain areas during flooding. These forms can connect water between floodplains and main channel during the flood seasons, playing a key role in ecological processes (CitationStevaux et al., 2013). This seasonal flood is complex process not only affect the floodplain ecology but also controls hydrogeomorphology of the floodplains documented in CitationPark and Latrubesse (2017). Ridge and swale are a landform consisting of regular, parallel sand-ridges alternating with marshy depressions.
Proximal floodplain is periodically flooded terrains immediately adjacent to the main channel. It has elongated shape parallel to the main channel, with changes in width controlled by marginal bedrocks. Sedimentation in swampy areas is dominated by silt and clay, but with presence of fine sands in other associated features as levees, paleo-levees, and crevasse splays. Levees are narrow and elongated ridges that occur irregularly along the main channel ((A and B)). They have asymmetric morphology, with low angle slopes towards the floodplain and high angles to the channel, and are composed of clay, silt and very fine to fine sands. Levees crests have the highest elevation (from 85 to 90 m.a.s.l.) in the floodplain, and they are entirely covered by forest vegetation ((B)). A Crevasse splay is recognized in the proximal floodplain (). This landform is a local high and has three main lobes that probably were attached to a past position of the main channel (). Rossetti et al. (Citation2015) revealed paleoenvironments including active channel, abandoned channel, point bar, crevasse splay and floodplain, which are altogether compatible with meandering fluvial systems, thus indicating evolution processes of the evolution plan. Paleo-levees occur decoupled of the main channel and they are frequently associated with paleo-channels in the floodplains and lower terrace levels.
Figure 6. Geomorphic features of the floodplain. Location of photos and images in (C): (A) Levee within the proximal floodplain; (B) Levee located on the right bank of the main channel and covered by arboreal vegetation; (C) Lakes formed by paleo-channels within the floodplain; (D) Field view of paleo-channel. Figure (A) and (B) with images from Digital Globe, GeoEye, change updated: August 8, 2019 to Accessed: August 8, 2019.
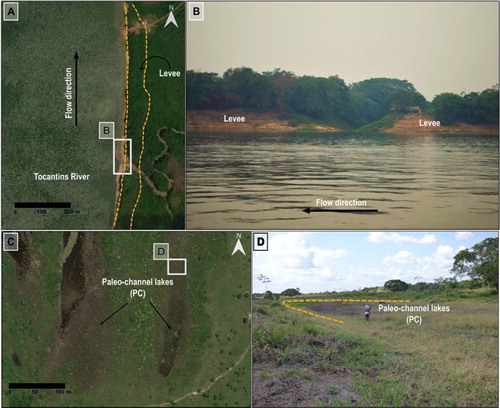
4.2.3. Fluvial terraces
Fluvial Terraces are relatively flat terrains not susceptible to seasonal flooding. In the studied area, three terrace levels were mapped based on their morphological characteristics and elevation (). The Upper Terrace (T1) level is related to fluvial deposits occurring between 43 and 66 m above the water level of the Tocantins River channel (105–110 m.a.s.l.). The surface is almost flat with low drainage density (70 m/km²) that carved narrow (17 m width) and shallow valleys (5 m depth). The deposits are predominantly composed of massive medium sands. The sediment color in outcrops varies from dark to light gray at the top and yellowish to the base, suggesting pedogenetic processes, with an upper horizon rich in organic matter and a lower horizon enriched in iron-minerals. The shallow water table (1.6 m depth at September 2018) maintains numerous perennial lakes in this unit, displaying circular morphology with average radius about 116 m. At the base of T1, there are conglomerate layers with around 1 m thick, but eventually reaching up to 3 m. Conglomerate layers are well-exposed at the base of terraces in the northwest and west portions of the area. At the base of this unit, the conglomerates have polymictic and well-rounded clasts and they occur as layers with incipient grading, tabular geometry, and wavy top. Grain size of the framework varies from granules to cobbles of sandstones, iron-rich duricrust, granites, gneiss and quartzite immersed in a reddish matrix of sandy mud.
Figure 8. Geomorphic features of the terraces and paleo alluvial fan: (A and B) Regional relationship between fluvial terraces (T1 and T2), paleo alluvial fan and the fluvial plain, where an abrupt slope break marks the inner boundary of the proximal floodplain. Image A from Esri World Imagery, updated at Aug 8, 2019; Image B MERIT-DEM. Location in .
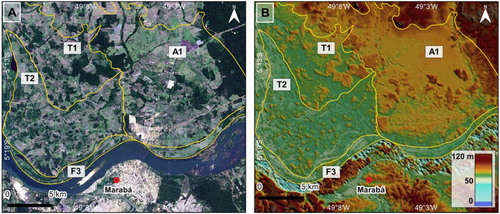
Lower Terraces (T2) are between 27 and 36 m above the river level (84 and 86 m.a.s.l.), with surface slightly tilted to the main channel. This unit is marked by high density of paleo-channels that give origin to lakes in the modern floodplain. These lakes can be connected to the main channel during the flood season. The paleo-channels are gradually filled by suspended fine-grained sediments (clay and silt) during flooding events and can show meander scrolls-bars in an advanced stage of degradation. The drainage network has low density, with channels showing shallow incision. The contact between lower level terraces and floodplains is marked by an abrupt slope. Flooding of the lower terraces may occur during high magnitude precipitation events. Most of the lower terraces are composed of massive fine sand with clay. Sediment color ranges from red-yellowish at the top to yellowish at the bottom.
4.2.4. Paleo-alluvial fans
The paleo-alluvial fans unit is made up of unconsolidated massive medium sands accumulated in a relatively high topographic position (90–100 m.a.s.l.). These sediments appear to derive from streams that drain hillslopes in the north portion of the area, and which spread out over a gently slope surface related to the ancient floodplain of the Tocantins River. The present morphology includes deposits with a convex-up transversal profile between the Flexeiras and Geladinho rivers and ephemeral lakes (). Ephemeral lakes are semicircular closed depressions showing seasonal flooding, usually disappear during drought and fill up again with water in rainy seasons. The lakes are concentrated in the north portion of the unit and are filled by dark brown mud over grayish fine sands. The seasonal variation of water table controls the vegetation distribution, with grasses in the center and shrub in the margins of the lakes. This unit is dissected by low density drainage network, with streams incised in narrow (17 m) and shallow valleys (5 m). The sedimentary deposit consists of metric-thick layers of quartz-rich white sands intercalated with three horizons of the 10–20 cm organic paleosols. The sandy layers are dominated by well-sorted fine to medium sands, with well-rounded grains. An upper layer of medium sands occurs in the paleo-alluvial fan unit as well as in the surrounding fluvial terraces (T2). This upper sand sheet might represent the eolian or alluvial reworking of the underlaying units ((B)).
Figure 9. Geomorphic features of the paleo-alluvial fan unit. Longitudinal and transversal topographic profiles showing upward convex shape. Map showing the boundaries of the main paleo-alluvial fan, with indication of the longitudinal (line A-A’) and transverse (line B-B’ and line C-C’) profiles. Topographic data are from MERIT-DEM.
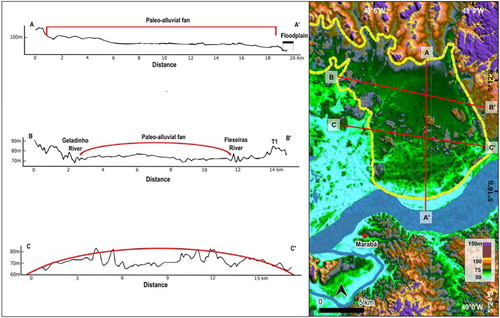
Figure 10. General aspects of the paleo-alluvial fans geomorphological unit. (A) Patches of diverse vegetation that comprises a mosaic of grasslands, shrubs, and arboreal forest spots; (B) Partial view of the sand sheet; (C) View of grasses and palm trees. Location in .
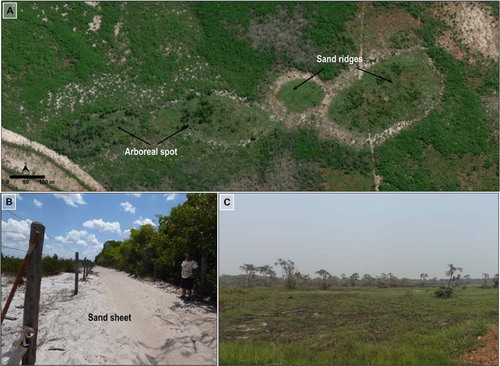
Most of the paleo-alluvial fan surfaces are covered by patches of diverse vegetation that comprises a mosaic of grasslands, shrubs and arboreal forest spots ((A and C)). The sand ridges arboreal spots are small areas (103–105 m2) with substrates with elevation higher (∼1 m higher) than adjacent grasslands and supporting a dense forest cover ((A)). The forests spots are developed over soils with an upper thin layer of gray mud covering quartz-rich sands ((B)). Open vegetation areas have been identified across the Amazon and they can be associated with the development of alluvial fans (CitationCordeiro et al., 2016) and eolian deposits (CitationZular et al., 2019).
5. Discussion and conclusions
Three mapping categories representing different depositional environments and their specific landforms were described in the middle Tocantins River. The mapping and elevation surveys of landforms suggest that the evolution of the plain comprised stages of sediment aggradation and erosion. In addition, the tributaries of the Tocantins River influenced the physiography of the modern and ancient alluvial valley by segmenting the floodplain into patches of different environments, including the development of paleo-alluvial fans, which contrasts with the modern contributary fluvial system.
The alternation between stages of aggradation and erosion can be the result of variations in water and sediment supply due to climate changes affecting precipitation in the watershed (e.g. CitationVandenberghe, 2003). The hierarchical classification of geomorphic units allows to define a relative chronology of the mapped landforms. The geomorphic map presented in this study can be useful in environmental and socio-economic planning of activities connected with the Tocantins River and its floodplains since the delineation and characterization of mapping units give information about physical dynamics of the landscape under human interventions, including for example substrate types and susceptibility to seasonal or historical flooding. Floodplain areas commonly host urban populations or agriculture activities due to the favorable characteristics of relief and soils (CitationRoss, 2016). Part of the city of Marabá is located on the Proximal Floodplain (Main Map), which is seasonally flooded by the Tocantins and Itacaiúnas Rivers. This floodplain is the most extensive floodplain of the Tocantins River, and the place where recruitment occurs for migratory and non-migratory fishes (CitationAkama, 2017). Therefore, it is an important habitat for conservation of aquatic ecosystems.
Historically, the Tocantins River main stem is the most impacted tributary of the Amazon fluvial system, with several already built dams, while the Araguaia River is still well-preserved due to absence of dams (CitationLatrubesse et al., 2019). However, the building of the planned Marabá hydropower plant in the Tocantins River will affect both rivers, with potential severe effects on the studied floodplains and related aquatic ecosystems (CitationICMBio, 2018; CitationLees et al., 2016; CitationWinemiller et al., 2016).
In summary, the geomorphological and morphometric analyses from satellite images, DEMs and field surveys allow us to map three main geomorphic units named fluvial plain, fluvial terraces, and paleo-alluvial fans. The origin of the mapped landforms and their sedimentary deposits are related to active and ancient fluvial systems. The hierarchical organization of the geomorphic units provides new information about relative timing of landform formation, being potential archives to reconstruct environmental conditions of the terrains drained by Tocantins River and its tributaries.
Software
QGIS 2.18.19 and Global Mapper 18 software was used for map data visualization and analysis purposes. Some output maps were redrawn using Inkscape 0.92. The map is designed to be color printed at A1 (594 × 841 mm) size for optimum clarity of font, point size and shading.
Financing
This research had financial support of the projects ‘Dimensions US-BIOTA-São Paulo: Assembly and evolution of the Amazonian biota and its environment: an integrated approach’ (São Paulo Research Foundation-FAPESP grant#2012/50260-6, US National Science Foundation and NASA grant#1241066) and ‘The response of sedimentary dynamics of the Xingu and Tapajós Rivers to climate changes and hydropower dams: risks for biodiversity conservation and energy production in Amazonia’ (FAPESP grant#2016/02656-1). JJ is thankful to the Master’s scholarship from Coordination of Superior Level Staff Improvement, Brazil (CAPES) – Finance Code 001. FNP was supported for a postdoctoral fellowship FAPESP grant#2014/23334-4. FNP and AOS are supported by National Council for Scientific and Technological Development (CNPq, Brazil) grants #302411/2018-6 and #304727/2017-2, respectively. The opinions, hypotheses, conclusions, and recommendations expressed in this material are responsibility of the author (s) and do not necessarily reflect FAPESP’s and CAPES vision.
main_map_24julho2020_finale.pdf
Download PDF (112 MB)Acknowledgements
JJ is grateful for Federal University of South and Southeast Pará (Unifesspa) for car support for field activity and Geologist Elianne Conde from Unifesspa for their help during field work.
Disclosure statement
No potential conflict of interest was reported by the author(s).
Additional information
Funding
References
- Akama, A. (2017). Impacts of the hydroelectric power generation over the fish fauna of the Tocantins River, Brazil: Marabá Dam, the Final Blow. Oecologia Australis , 21 (3 Special Issue), 222–231. https://doi.org/10.4257/oeco.2017.2103.01
- Akter, S. , Md, R. , Ali, E. , Karim, S. , Khatun, M. , & Alam, M. (2018). Geomorphological, geological and engineering geological aspects for sustainable urban planning of Mymensingh City, Bangladesh. Open Journal of Geology , 08 (07), 737–752. https://doi.org/10.4236/ojg.2018.87043
- Almeida, F. F. M. , Hasui, Y. , Brito Neves, B. B. , & Fuck, R. A. (1977). Províncias Estruturais Brasileiras. Ln: Simpósio de Geologia Do Nordeste, 8, Campina Grande, PB.
- ANA . (2002). A Evolução da Gestão dos Recursos Hídricos no Brasil, Agência Nacional de Águas . Brasília-DF. http://arquivos.ana.gov.br/institucional/sge/CEDOC/Catalogo/2002/AEvolucaodaGestaodosRecursosHidricosnoBrasil.pdf.
- ANA . (2005). Cadernos de Recursos Hídricos – Aproveitamento Do Potencial Hidráulico Para Geração de Energia. Brasília-DF. http://arquivos.ana.gov.br/planejamento/planos/pnrh/VF GeraçaoEnergia.pdf
- ANA, Agência Nacional de Águas . (2017). Plano Estratégico Da Bacia Hidrográfica Dos Rios Tocantins e Araguaia. 204. http://www3.ana.gov.br/portal/ANA/todos-os-documentos-do-portal/documentos-spr/planos-de-bacia/plano-estrategico-de-recursos-hidricos-da-bacia-hidrografica-dos-rios-tocantins-e-araguaia-relatorio-sintese
- Antoine, P. , Lozouet, N. L. , Chaussé, C. , Lautridou, J. P. , Pastre, J. F. , Auguste, P. , Bahain, J. J. , Falguères, C. , & Galehb, B. (2007). Pleistocene fluvial terraces from Northern France (Seine, Yonne, Somme): Synthesis, and new results from Interglacial deposits. Quaternary Science Reviews , 26 (22–24), 2701–2723. https://doi.org/10.1016/j.quascirev.2006.01.036
- Bangen, S. G. , Wheaton, J. M. , Bouwes, N. , Bouwes, B. , & Jordan, C. (2014). A methodological intercomparison of topographic survey techniques for characterizing wadeable streams and rivers. Geomorphology , 206 , 343–361. https://doi.org/10.1016/j.geomorph.2013.10.010
- Bigarella, J. J. (1973). Geology of the Amazon and Parnaiba Basins. In Nairn, A. E. M. & Stehli, F. G. (Eds.), The South Atlantic (pp. 25–86). Springer US. https://doi.org/10.1007/978-1-4684-3030-1_2
- Brasil . (2012). Plano Decenal de Expansão de Energia 2021 . Ministério de Minas e Energia, Empresa de Pesquisa Energética.
- Bridgland, D. R. , & Westaway, R. (2014). Quaternary fluvial archives and landscape evolution: A global synthesis. Proceedings of the Geologists’ Association , 125 (5–6), 600–629. https://doi.org/10.1016/j.pgeola.2014.10.009
- Brierley, G. J. , & Fryirs, K. A. (2005). Geomorphology and River management: Applications of the River Styles framework . Blackwell Publishing.
- Brito Neves, B,B , & Cordani, U. G. (1991). Tectonic evolution of South America during the Late Proterozoic. Precambrian Research , 53 (1–2), 23–40. https://doi.org/10.1016/0301-9268(91)90004-T
- Cordeiro, C. L. O. , Rossetti, D. F. , Gribel, R. , Tuomisto, H. , Zani, H. , Ferreira, C. A. C. , & Coelho, L. (2016). Impact of sedimentary processes on white-sand vegetation in an Amazonian Megafan. Journal of Tropical Ecology , 32 (6), 498–509. https://doi.org/10.1017/S0266467416000493
- Costa, J. B. S. , Hasui, Y. , Bemerguy, R. L. , Soares-Júnior, A. V. , & Villegas, J. M. C. (2002). Tectonics and paleogeography of the Marajó Basin, Northern Brazil. Anais Da Academia Brasileira de Ciencias , 74 (3), 519–531. https://doi.org/10.1590/S0001-37652002000300013
- Dagosta, F. C. P. , & Pinna, M. (2019). The fishes of the Amazon : distribution and Biogeographical Patterns, with a Comprehensive List of species. Bulletin of the American Museum of Natural History , 2019 (431), 1–163. https://doi.org/10.1206/0003-0090.431.1.1
- Deus, S. C. S. , Neves, R. J. J. , Jauche, E. , Almeida, C. , Faial, K. R. F. , Medeiro, A. C. , Mendes, R. A. , Faial, K. C. F. , Leite, J. C. , & Deus, R. J. A. (2018). Streamflow forecasts due precipitation water in a tropical large watershed at Brazil for flood Early warning, based on SWAT model. ITEGAM – Journal of Engineering and Technology for Industrial Applications , 4 (14), 4–14. https://doi.org/10.5935/2447-0228.20180027
- Dunne, T. , Mertes, L. A. K. , Meade, R. H. , Richey, J. E. , & Forsberg, B. R. (1998). Exchanges of sediment between the flood plain and channel of the Amazon River in Brazil. Bulletin of the Geological Society of America , 110 (4), 450–467. https://doi.org/10.1130/0016-7606(1998)110<0450:EOSBTF>2.3.CO;2
- Esri . (2019). World imagery.
- Felipe, L. B. , & Morales, N. (2017). Influência Neotectônica Na Evolução Geomorfológica e Geológica Da Região de Marabá-PA. Contribuições à Geologia Da Amazônia , 10 , 145–161. http://www.sbg-no.org.br/
- Fryirs, K. A. , & Brierley, G. J. (2012). Geomorphic analysis of river systems. Geomorphic Analysis of River Systems. https://doi.org/10.1002/9781118305454
- Garreaud, R. D. , Vuille, M. , Compagnucci, R. , & Marengo, J. (2009). Present-day South American climate. Palaeogeography, Palaeoclimatology, Palaeoecology , 281 (3–4), 180–195. https://doi.org/10.1016/j.palaeo.2007.10.032
- Geosage . (2018). Spectral transformer GUI landsat-8 Imagery®. http://www.geosage.com/highview/features_landsat8
- Google Earth Pro© . (2019). Google Earth Pro©. 7.3.2.5776 (64-bit). kh.google.com
- Góes, A. M. (1995). Formação Poti (Carbonífero Inferior) Da Bacia Do Parnaiba. Universidade de São Paulo. https://doi.org/10.11606/T.44.1995.tde-11022014-105309
- Hamilton, S. K. , Kellndorfer, J. , Lehner, B. , & Tobler, M. (2007). Remote sensing of floodplain geomorphology as a surrogate for biodiversity in a tropical River system (Madre de Dios, Peru). Geomorphology , 89 (1-2 SPEC. ISS.), 23–38. https://doi.org/10.1016/j.geomorph.2006.07.024
- Hayakawa, E. H. , Rossetti, D. F. , & Valeriano, M. M. (2010). Applying DEM-SRTM for Reconstructing a Late Quaternary Paleodrainage in Amazonia. Earth and Planetary Science Letters , 297 (1–2), 262–270. https://doi.org/10.1016/j.epsl.2010.06.028
- Herz, N. , Hasui, Y. , Costa, J. B. S. , & Matta, M. A. S. (1989). The Araguaia Fold Belt, Brazil: A Reactivated Brasiliano-Pan-African Cycle (550 Ma) Geosuture. Precambrian Research , 42 (3–4), 371–386. https://doi.org/10.1016/0301-9268(89)90020-X
- ICMBio . (2018). Livro Vermelho Da Fauna Brasileira Ameaçada de Extinção: Volume VI – Peixes. In Instituto Chico Mendes de Conservação Da Biodiversidade (Org.). Livro Vermelho Da Fauna Brasileira Ameaçada de Extinção, 1st ed., VI-Peixes:1232. Brasília, DF.
- Inkscape . (2007). Inkscape ProjectTM 0.92.3. GNU General Public License Version 3.
- Jones, A. F. , Brewer, P. A. , Johnstone, E. , & Macklin, M. G. (2007). High-resolution interpretative geomorphological mapping of river valley environments using airborne LiDAR data. Earth Surface Processes and Landforms , 32 (10), 1574–1592. https://doi.org/10.1002/esp.1505
- Latrubesse, E. , Arima, E. , Ferreira, M. , Nogueira, S. , Wittmann, F. , Dias, M. S. , Dagosta, F. , & Baye, M. (2019). Fostering water Resource Governance and conservation in the Brazilian Cerrado Biome. Conservation Science and Practice , 1 (9), 1–8. https://doi.org/10.1111/csp2.77
- Lees, A. C. , Peres, C. A. , Fearnside, P. M. , Schneider, M. , & Zuanon, J. A. S. (2016). Hydropower and the future of Amazonian biodiversity. Biodiversity and Conservation , 25 (3), 451–466. https://doi.org/10.1007/s10531-016-1072-3
- Lewin, J. , & Ashworth, P. J. (2014). The negative relief of large river floodplains. Earth Science Reviews , 129 , 1–23. https://doi.org/10.1016/j.earscirev.2013.10.014
- Lewin, J. , Davies, B. , & Wolfenden, P. (1977). Interactions between channel change and Historic Mining sediments. River Channel Changes , 353–367. https://hwbdocuments.env.nm.gov/Los Alamos National Labs/General/14434.pdf
- Lima, F. C. T. , & Caires, R. A. (2011). Fishes from the Serra Geral Do Tocantins ecological Station, Rio Tocantins and Rio São Francisco Basins, with Remarks on the Biogeographical implications of the Common Headwater between the Rio Sapão and Rio Galheiros. Biota Neotropical , 11 (1), http://www.biotaneotropica.org.br/v11n1/en/abstract?article+bn03411012011.%0AAbstract
- Lima, F. C. T. , & Ribeiro, A. C. (2011). Continental-scale tectonic controls of biogeography and ecology continental-scale tectonic controls of biogeography and ecology. Historical Biogeography of Neotropical Freshwater Fishe , 145–164. https://doi.org/10.1525/california/9780520268685.003.0009
- Marengo, J. A. , & Espinoza, J. C. (2016). Extreme seasonal droughts and floods in Amazonia: Causes, trends and impacts. International Journal of Climatology , 36 (3), 1033–1050. https://doi.org/10.1002/joc.4420
- Mascarenhas, A. L. S. , Vidal, M. R. , & Silva, E. V. (2013). O Uso Do SIG Para Definição de Aspectos Geomorfológicos No Médio Curso Do Rio Tocantins Parte Oriental Da Bacia Amazônica. Revista Geoamazônia , 2 (1), 68–78. https://doi.org/10.17551/2358-1778/geoamazonia.n1v2p68-78
- Merino, E. R. , Pupim, F. N. , Macedo, H. A. , & Assine, M. L. (2015). Enhancement and integration of optical satellite images with SRTM data for mapping and study of large fluvial plains: Examples in the Brazilian Pantanal. Revista Brasileira de Geomorfologia , 16 (1), 49–62. https://doi.org/10.20502/rbg.v16i1.626
- Merona, B. (1987). Aspectos Ecológicos Da Ictiofauna No Baixo Tocantins. Acta Amazonica Único , 17 , 109–124. https://doi.org/10.1590/1809-43921987171124
- Mertes, L. A. K. , Dunne, T. , & Martinelli, L. A. (1996). Channel-Floodplain geomorphology along the Solimões-Amazon River, Brazil. Bulletin of the Geological Society of America , 108 (9), 1089–1107. https://doi.org/10.1130/0016-7606(1996)108<1089:CFGATS>2.3.CO;2
- Mesner, J. C. , & Wooldridge, L. C. P. (1964). Maranhão Paleozoic Basin and Cretaceous Coastal Basins, North Brazil. Bulletin of the American Association of Petroleum Geologists , 48 (9), 1475–1512. https://doi.org/10.1306/BC743D99-16BE-11D7-8645000102C1865
- Mérona, B. , Juras, A. A. , Santos, G. M. , & Cintra, I. H. A. (2010). Os Peixes e a Pesca No Baixo Rio Tocantins Vinte Anos Depois Da UHE Tucuruí.
- Miall, A. D. (2014). Fluvial depositional systems. Springer Geology. Toronto. https://doi.org/10.1007/978-3-319-00666-6
- Miklín, J. , & Galia, T. (2017). Detailed fluvial-geomorphologic mapping of Wadeable streams: A proposal of universal map symbology. Journal of Maps , 13 (2), 698–706. https://doi.org/10.1080/17445647.2017.1355275
- MMA . (2006). Caderno Da Região Hidrográfica Do Tocantins-Araguaia. Projects B. Brasília-DF: Ministério do Meio Ambiente, Secretaria de Recursos Hídricos.
- Nanson, G. C. , & Croke, J. C. (1992). A genetic classification of floofplains. Geomorphology , 4 (October), 459–486. https://doi.org/169-555X/92/
- Oliveira-Filho, A. T. (1995). A study of the origin of Central Brazilian forests by the analysis of plant species distribution Patterns. Edinburgh Journal of Botany , 55 , https://doi.org/10.1017/S0960428600000949
- Park, E. , & Latrubesse, E. (2017). The hydro-geomorphologic complexity of the lower Amazon River floodplain and hydrological connectivity assessed by remote sensing and field control. Remote Sensing of Environment , 198 , 321–332. https://doi.org/10.1016/j.rse.2017.06.021
- Passos, M. , Soares, E. , Tatumi, S. , Yee, M. , Mittani, J. , Hayakawa, E. , & Salazar, C. (2020). Pleistocene-Holocene sedimentary deposits of the Solimões-Amazonas fluvial system, western Amazonia. Journal of South American Earth Sciences , 98 (November 2019), 102455. https://doi.org/10.1016/j.jsames.2019.102455
- Pazzaglia, F. J. (2013). Fluvial Terraces. In Treatese on Geomorphology, edited by J. F. Shroder, 1st ed., 379–412. http://citeseerx.ist.psu.edu/viewdoc/download?doi=10.1.1.211.535&rep=rep1&type=pdf
- Pupim, F. , M, N. , Assine, L. , & Sawakuchi, A. O. (2017). Late Quaternary Cuiabá Megafan, Brazilian Pantanal: Channel Patterns and Paleoenvironmental changes. Quaternary International , 438 , 108–125. https://doi.org/10.1016/j.quaint.2017.01.013
- RadamBrasil . (1986). RadamBrasil Folha SB.22-X-D Marabá. Programa Levantamentos Geológicos Básicos Do Brasil. Carta Geólogica – Escala 1:250.000 – Anexo I SB.22-X-D.
- Ribeiro, M. C. L. B. , Petrere, M. , & Juras, A. (1995). Ecological Integrity and Fisheries ecology of the Araguaia—Tocantins River Basin, Brazil. Regulated Rivers: Research & Management , 11 (3–4), 325–350. https://doi.org/10.1002/rrr.3450110308
- Ross, J. L. S. (2016). Relevo Brasileiro No Contexto Da América Do Sul. Revista Brasileira de Geografia , 61. https://doi.org/10.21579/issn.2526-0375_2016_n1_art_2
- Rossetti D. F. , Cohen, M. C. L. , Tatumi, S. H. , Sawakuchi, A. O. , Cremon, É. H. , Mittani, J. C. R. , Bertani, T. C. , Munita, C. J. A. S. , Tudela, D. r. G. , Yee, M. , & Moya, G. (2015). Mid-Late Pleistocene OSL chronology in western Amazonia and implications for the transcontinental Amazon pathway. Sedimentary Geology , 330 , 1–15. https://doi.org/10.1016/j.sedgeo.2015.10.001
- Rossetti, D. F. , Toledo, P. M. , & Góes, A. M. (2005). New geological framework for western Amazonia (Brazil) and implications for Biogeography and evolution. Quaternary Research , 63 (1), 78–89. https://doi.org/10.1016/j.yqres.2004.10.001
- Rossetti, D. F. , & Truckenbrodt, W. (1997). Revisão Estratigráfica Para Os Depósitos Do Albiano-Terciário Inferior (?) Na Bacia de São Luís, Maranhão. Bol. Mus. Para. Emílio Goeldi, Sér. Ciênc. Da Terra , 9 , 29–41.
- Sinha, R. , Jain, V. , Babu, G. P. , & Ghosh, S. (2005). Geomorphic characterization and Diversity of the fluvial systems of the Gangetic Plains. Geomorphology , 70 (3-4 SPEC. ISS.), 207–225. https://doi.org/10.1016/j.geomorph.2005.02.006
- Slingerland, R. , & Smith, N. D. (2004). River Avulsions and their deposits. Annual Review of Earth and Planetary Sciences , 32 (1), 257–285. https://doi.org/10.1146/annurev.earth.32.101802.120201
- Stevaux, J. C. , Corradini, F. A. , & Aquino, S. (2013). Connectivity processes and Riparian vegetation of the upper Paraná River, Brazil. Journal of South American Earth Sciences , 46 , 113–121. https://doi.org/10.1016/j.jsames.2011.12.007
- Thom, G. , Xue, A. , Sawakuchi, A. O. , Ribas, C. C. , Hickerson, M. J. , Aleixo, A. , & Miyaki, C. (2020). Quaternary climate changes as Speciation Drivers in the Amazon floodplains. Science Advances , 6 (11), eaax4718. https://doi.org/10.1126/sciadv.aax4718
- Tobler, W. (1987 January). Measuring spatial resolution. Land Resources Information Systems Conference , 1 , 12–16.
- Vandenberghe, J. (2003). Climate Forcing of fluvial system development: An evolution of Ideas. Quaternary Science Reviews , 22 (20), 2053–2060. https://doi.org/10.1016/S0277-3791(03)00213-0
- Vasquez, M. L. , & Rosa-Costa, L. T. (2008). Geologia e Recursos Mineirais Do Estado Do Pará: Sistema de Informações Geográficas – SIG. Edited by Programa Geologia do Brasil (PGB). Integração e Difusão de Dados da Geologia do Brasil. Mapas Geológicos Estaduais. 1st ed. Belém: CPRM.
- Verstappen, H. T. (1977). Remote sensing in geomorphology . Amsterdam: Elsevier Scientific Publishing Company, 4, 214 p. ISBN 13: 9780444410863.
- Villegas, J. M. C. (1994). Geologia Estrutural Da Bacia Do Marajó. Universidade Federal do Pará.
- Wheaton, J. M. , Fryirs, K. A. , Brierley, G. , Bangen, S. G. , Bouwes, N. , & O’Brien, G. (2015). Geomorphic mapping and Taxonomy of fluvial landforms. Geomorphology , 248 , 273–295. https://doi.org/10.1016/j.geomorph.2015.07.010
- Williams, R. D. , Brasington, J. , Vericat, D. , & Hicks, D. M. (2014). Hyperscale terrain Modelling of Braided rivers: Fusing Mobile Terrestrial Laser Scanning and optical Bathymetric mapping. Earth Surface Processes and Landforms , 39 (2), 167–183. https://doi.org/10.1002/esp.3437
- Winemiller K. O. , McIntyre, P. B. , Castello, L. , Fluet-Chouinard, E. , Giarrizzo, T. , Nam, S. , Baird, I. G. , Darwall, W. , Lujan, N. K. , Harrison, I. , Stiassny, M. L. J. , Silvano, R. A. M. , Fitzgerald, D. B. , Pelicice, F. M. , Agostinho, A. A. , Gomes, L. C. , Albert, J. S. , Baran, E. , Petrere Jr. M. , … Sáenz, L. (2016). Balancing hydropower and biodiversity in the Amazon, Congo, and Mekong. Science , 351 (6269), 128–129. https://doi.org/10.1126/science.aac7082
- Yamazaki, D. , Ikeshima, D. , Tawatari, R. , Yamaguchi, T. , O’Loughlin, F. , Neal, J. C. , Sampson, C. C. , Kanae, S. , & Bates, P. D. (2017). A high-Accuracy Map of Global terrain elevations. Geophysical Research Letters , 44 (11), 5844–5853. https://doi.org/10.1002/2017GL072874
- Zhou, J. , & Lau, K. M. (1998). Does a Monsoon climate Exist over South America? Journal of Climate , 11 (5), 1020–1040. https://doi.org/10.1175/1520-0442(1998)011<1020:DAMCEO>2.0.CO;2
- Zinck, J. A. (2016). Geopedology: An Integration of Geomorphology and Pedology for Soil and Landscape Studies . Cham: Springer International Publishing. https://doi.org/10.1007/978-3-319-19159-1
- Zular, A. , Sawakuchi, A. O. , Chiessi, C. M. , D’Horta, F. M. , Cruz, F. W. , Demattê, J. , Ribas, C. C. , Hartmann, G. , Giannini, P. C. , & Amaral Soares, E. A. (2019). The role of abrupt climate change in the Formation of an Open vegetation Enclave in Northern Amazonia during the Late Quaternary. Global and Planetary Change , 172 , 140–149. https://doi.org/10.1016/j.gloplacha.2018.09.006