ABSTRACT
This work presents the outcomes of the geomorphological investigation and mapping carried out within the Cinque Terre National Park (38 km2), an outstanding example of a human-modified landscape. Over the centuries, the natural landscape of Cinque Terre has been almost completely modified by slope terracing for agricultural purposes. Extensive field surveys, topographic maps examination and aerial photographs interpretation have led to the production, within a GIS environment, of a new geomorphological map at 1:18,000 scale which outlines the main genesis and related landforms and deposits: (i) gravity-induced features, (ii) fluvial and runoff features, (iii) coastal features and (iv) anthropogenic features. Special attention has been given to the mapping of terraced slopes, which at present are extremely vulnerable to gravity-driven processes and running water because of extensive farmland abandonment. The output map is a fundamental basis for future activities of hazard assessment and zonation and the definition of land management strategies.
1. Introduction
Geomorphological maps are a fundamental source of knowledge about the Earth’s surface dynamics since they provide the spatial distribution of landforms along with the description of their interrelations with geology, surficial geomorphic processes and climatic conditions (CitationGriffiths et al., 2011; CitationVerstappen, 2011). In the last decades, numerous geomorphological mapping activities addressed the comprehension of the interaction between natural processes and the anthroposphere, therefore, assuming an increasingly important role in the field of geo-hazards prevention and mitigation (CitationAlcántara-Ayala, 2002; CitationDramis et al., 2011; CitationPetley, 1998; CitationRosenfeld, 1994). At the same time, landforms mapping has undergone a period of great development, mainly due to the advent of both Geographical Information Systems (GIS) (CitationGustavsson et al., 2006) and remote sensing techniques (CitationSmith, 2011). Consequently, geomorphological mapping currently represents an essential step for risk assessment procedures in a wide spectrum of geo-hazards such as floods (CitationFurdada et al., 2008; CitationHo & Umitsu, 2011; CitationMandarino et al., 2019; Citation2020), landslides (CitationCardinali et al., 2002; CitationCevasco et al., 2018; CitationGuerriero et al., 2019; CitationParise, 2001; Citationvan Westen et al., 2008), earthquakes (CitationErdik et al., 1999; CitationGanapathy & Rajawat, 2012; CitationOrdaz & Reyes, 1999), volcanic-related processes (CitationIntrieri et al., 2013; CitationThouret, 2010; CitationVerstappen, 1992) and sea storms (CitationDe Pippo et al., 2008; CitationFerrari et al., 2019; CitationShort, 2007).
Anthropogenic landscapes are widespread worldwide, representing a challenging aspect of geomorphological mapping (CitationLi et al., 2017). These landscapes are produced by the extensive transformations of the natural morphology by human activities (CitationSzabó et al., 2010). A geomorphological map capable of grasping processes and landforms related to natural and anthropic geomorphologic agents can provide crucial contributions to both hazard assessment and land management (CitationBrandolini et al., 2019; CitationDel Monte et al., 2016; CitationLatocha, 2009; CitationMandarino et al., 2020).
The Cinque Terre area (eastern Liguria, north-western Italy) can be considered as one of the most representative examples of human-modified landscape. Starting from the early centuries of the Middle Ages, local farmers have almost completely modified the original slope topography through the construction of dry-stone walls, creating an outstanding terraced coastal landscape (CitationBrandolini, 2017). Based on the environmental, scenic and historical significance, such an artificial landscape has been acknowledged since 1997 as a World Heritage Site by UNESCO and subsequently, in 1999, it has been declared National Park. The Cinque Terre territory is extremely dynamic since it is characterized by a complex geological and geomorphological setting, where many surficial geomorphic processes coexist, along with peculiar weather conditions (CitationCevasco et al., 2015; CitationScopesi et al., 2015). Moreover, this artificial landscape experienced important land-use changes over the last century (CitationAgnoletti et al., 2019; CitationBrandolini, 2017), mostly related to the abandonment of agricultural activity. However, there are no updated works describing the geomorphology at the scale of the whole national park.
This work presents the outcomes of geomorphological investigations carried out within the territory of the Cinque Terre National Park (hereafter CTNP). Extensive field surveys, integrated with the collection of ancillary information and the analysis of remotely sensed data, allowed to prepare a geomorphological map and a related database which outlined the wide variety of processes and landforms of the study area. Concerning man-made landforms, particular emphasis has been placed on the degree of abandonment of agricultural terraces. It is known that terraced landscapes, when no longer properly maintained, become more prone to erosion processes and mass movements (CitationBrandolini, Cevasco, et al., 2018; CitationLesschen et al., 2008; CitationMoreno-de-las-Heras et al., 2019; CitationSeeger et al., 2019). The output map represents a fundamental basis for future hazard assessment and zonation procedures, and it may also promote the geo-heritage of an already popular and visited area.
2. General setting of the study area
Located in the easternmost part of the Liguria region, the CTNP is a 38 km2 wide area that includes a narrow coastal zone and five small hamlets, representing an important national and international tourist captivation, and a small portion of territory belonging to the inner Vara Valley (). Notwithstanding its proximity to the sea, this area shows the peculiar characteristics of mountain landscapes due to its rugged morphology (CitationBrandolini, 2017). The highest reliefs, ranging on average from 400 to 800 m a.s.l., follow an approximately NW-SE trending alignment that runs parallel and very close to the shoreline, which is prevalently formed by rocky cliffs.
Geologically, the CTNP territory is characterized by the presence of five overlapping tectonics units belonging to the Tuscan, Sub-Ligurian and Ligurian domains of the Northern Apennine (from top to bottom): Gottero Unit, Ottone Unit, Marra Unit, Canetolo Unit and Tuscan Nappe (CitationAbbate et al., 2005). These units belong to an NW-SE oriented segment of the chain, which during the orogenic phases of the Tertiary age, were arranged in a large SW-verging anti-form fold (CitationGiammarino & Giglia, 1990). The Gottero Unit crops out in the westernmost part of the Park and mainly consists of an ophiolitic complex followed by a turbidite sequence (Late Cretaceous). The Ottone, Canetolo and Marra units crop out along thin and NW-SE trending strips of territory placed in the western and the central sectors of the CTNP. The first one is prevalently constituted of pelitic rocks (i.e. Monte Veri Complex) while the other ones are formed by claystones with limestones and silty-sandy turbiditic rocks (e.g. Canetolo shales and limestones Fm., Torrente Pignone marls Fm.). The largest extension of the park’s territory is occupied by the Tuscan Nappe, which primarily includes thick sandstone-claystone turbidites, diffusely cropping out both along the coast and inland and locally known as Macigno Fm. (upper Oligocene), and secondly by limestones, only visible in the proximity of the easternmost border of the CTNP. From a geomorphological point of view, the study area is characterized by small catchments with steep and very steep slopes (approximately 57% of the area shows slope gradient higher than 30°). These slopes are mantled by thin covers of eluvial-colluvial deposits, extensively reworked by human activities over the past centuries (CitationCevasco, Pepe, et al., 2013; CitationScopesi et al., 2020). The catchments, very small in extent (from less than 1 km2 up to approximately 6 km2), are drained by short and steep fluvial stems, whose mouths are often occupied by the main villages (CitationBrandolini, 2017). The most common landscape feature of the study area is represented by agricultural terraces, here sustained by dry-stone walls, and mainly built by local inhabitants since the 11th–12th century for agricultural purposes (CitationRellini et al., 2019; CitationTerranova, 1984, Citation1989; CitationTerranova et al., 2002). The physiographical features of the CTNP deeply control the local climate setting. The closeness to the sea produces a topographic effect that locally enhances the features of the peculiar Mediterranean climate, with warm dry summers and temperate winters. The average annual rainfall is about 1000 mm, with the rainiest period commonly comprised between October and November, when intense rainstorms often can occur (CitationCevasco et al., 2015). Extraordinary rainfall events are also very frequent due to the geomorphological setting, especially in the ongoing climate change context (CitationCevasco et al., 2015; CitationGalanti et al., 2018).
3. Materials and methods
Geomorphological mapping of CTNP was carried out through different phases. A first stage was achieved by analysing both unpublished and published geomorphological data (e.g. CitationCevasco, 2007; CitationFederici et al., 2001; CitationTerranova, 1984; Citation1989) and urban planning reports (CitationRegione Liguria, 2020a). The lithological and geo-structural features were derived from available official geological maps (CitationAbbate, 1969; CitationAbbate et al., 2005). The interpretation of sets of aerial-photos taken from 2010 to 2016 (CitationRegione Liguria, 2020b) and the most recent Google Earth image provided further support to the mapping activities (). The main geomorphological features identified with remotely sensed and archive data were then verified in the field. Indeed, accurate field surveys, conducted at a 1:2000 scale and distributed between October 2018 and March 2019, allowed a complete analysis of the whole CTNP territory. Surveyed landforms and related GNSS-points were, respectively, manually digitized and imported in a GIS environment to produce the 1:18,000 scale Main Map. Mapped features were overlaid on a DEM-derived shaded-relief map, along with general vector topographic features (CitationRegione Liguria, 2020b) (). The aforementioned DEM was also used to compute some basic morphometric parameters such as slope, aspect and terrain ruggedness index (CitationWilson et al., 2007). The digitalization of punctual, linear and areal processes and landforms was useful to generate a database containing qualitative (e.g. type, state of activity, etc.) and quantitative (e.g. length, extension, soil thickness, etc.) information. Terminology, symbology and landform classification criteria were adopted following the guidelines provided by the Italian Institute for Environmental Protection and Research (CitationCampobasso et al., 2018). Four categories of landforms and deposits were hence distinguished according to genesis: (i) gravity-induced landforms and deposits, (ii) fluvial and run-off landforms and deposits, (iii) coastal landforms and deposits and (iv) anthropogenic landforms and deposits.
Table 1. List of cartographic data used in this research.
Particular attention has been devoted to the identification and mapping of agricultural terraces by distinguishing four different land use and land cover classes: cultivated terraced area, (ii) terraced area covered by shrubs, (iii) terraced area covered by forest tree species and (iv) unterraced area, including mainly woods and minimally urban areas. This choice was made to evaluate the extent of terraced slopes over the whole CTNP and to underline the degree of abandonment of agricultural terraces.
4. Results and discussion
In this section, the landforms from natural and anthropogenic processes identified in the CTNP are described and their implications in terms of hazard and related risk are discussed.
4.1. Gravity-induced landforms and deposits
Landforms and deposits related to mass movements are extremely widespread revealing that gravity is one of the most important geomorphologic agents within the CTNP (CitationRaso et al., 2018; CitationRaso et al., 2019). More than 450 landslide deposits were mapped, showing that the landslide index within the whole CTNP is approximately equal to 11%. As recently reported by CitationRaso et al. (2019), the broad variety of gravity-induced phenomena, both in terms of mechanisms and magnitude (i.e. size and involved volumes) has different reasons (e.g. geological, geomorphological, land-use changes). The geostructural features significantly influence the occurrence of gravitational phenomena involving rock masses ((a)) such as falls, topples and planar slides (CitationRaso et al., 2016). The high relief of slopes, commonly mantled by thin soil covers, represents a crucial factor in favouring translational slides, whose action is often enhanced by diffuse and channelled erosion operated by running water. These dynamics often produces eluvial-colluvial deposits of moderate extension located at the foot of slopes. In some cases, mass movements can be controlled by human activity, such as along road cuts ((b)) or in old quarrying areas. Moreover, terraced slope degradation due to land abandonment influences the occurrence of shallow landslides such as debris slides and debris avalanches ((c)) (CitationCevasco, Brandolini, et al., 2013; CitationDi Napoli et al., 2020; CitationGalve et al., 2015; Citation2016; CitationSchilirò et al., 2018). The largest deposits are represented by ancient landslide bodies ((d)), in some cases affected by residual kinematics related to complex movement mechanisms (CitationFederici et al., 2001; CitationRaso et al., 2017; CitationTerranova, 1984, Citation1989). As regards the triggering factors, high-intensity rainfalls and sea-wave action along the coast are of primary importance. A more complete overview of landslide processes can be obtained from recent studies reported in the literature (e.g. CitationCevasco, Brandolini, et al., 2013; Raso et al. Citation2018; CitationRaso et al. 2019).
Figure 2. Examples of typical landslides in the CTNP. (a) Planar slides and falls involving sedimentary rock masses along the coast west of the Vernazza village; (b) slope instability affecting a road cut along the provincial road n° 51 in the upper sector of the Vernazza catchment; (c) rainfall-induced shallow landslides triggered on abandoned terraced slopes at Vernazza (modified from CitationCevasco et al. 2012); (d) frontal view of the ancient Guvano landslide body, whose toe is currently undermined by marine erosion; (e) active escarpment edges at the headland of Punta Merlino.
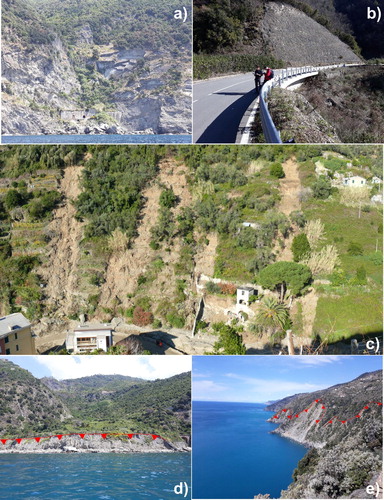
Erosion landforms related to gravity mainly concern active and dormant escarpment edges, as well as relict escarpment edges and slope breaks. Furthermore, debris/rock flow channels are often present along the coastal sectors showing very steep profiles. Coastal slopes are generally characterized by the presence of active and dormant scarps, close to and far from the shoreline, respectively. Specifically, in the eastern sector of the CTNP, some main morphological breaks and escarpments often mark the boundary between the sectors closest to the shoreline, characterized by steep profiles and wide extents of disrupted terraces, and the less steep upstream areas ((e)).
4.2. Fluvial and run-off landforms and deposits
Steep slopes and creeks along with high relief energy and small catchments of the CTNP highlight a clear prevalence of fluvial and surface-runoff-related landforms shaped by erosional processes. The CTNP territory is entirely made up of a series of main and minor valleys, whose characteristics reflect the litho-structural features of the region (CitationCevasco, 2007; CitationFanucci & Nosengo, 1979). Three geomorphologically homogeneous sectors were identified: (i) main catchments, (ii) minor valleys draining to the sea and (iii) areas belonging to the Vara basin ((a)).
Figure 3. (a) Valleys-related geomorphologically homogeneous sectors of the CTNP; (b) example of V-shaped valley upstream of Monterosso; (c) an example of very steep channel head at Mt. Pizzolo, Vara Catchment; (d) bedrock channel presenting rock steps and plunge pools in the Pastanelli Creek Catchment; (e) typical confined semi-alluvial channel of the main valleys characterized by widespread bedrock outcrops and coarse sediments, Vernazza Creek upstream of Vernazza; (f) reworked eluvial-colluvial deposits behind a collapsed dry-stone wall at Manarola.
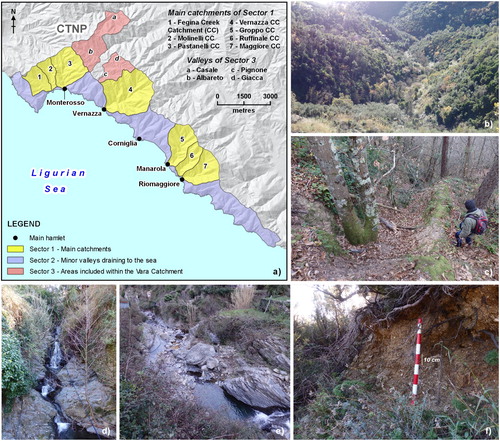
In the first sector, the main catchments ((a)) present a dense network of V-shaped valleys drained by steep and rather straight fluvial stems organized in a sub-dendritic pattern (CitationTwidale, 2004; CitationZernitz, 1932); the main fluvial stem direction is overall perpendicular to the shoreline. The second sector is characterized by small and steep V-shaped valleys that are approximately parallel to each other, perpendicular to the coast and drained by low-order channels. The latter often blend in debris/rock flow channels, especially along their downstream-most portion. The third sector presents first-sector-like V-shaped valleys (i.e. Casale and Giacca valleys) along with a gentler topography (i.e. Pignone and Albareto valleys). Such arrangement reflects the outcropping bedrock, here represented by the Macigno sandstones Fm. and by the Canetolo shales and limestones Fm., respectively. The trellis and sub-dendritic hydrographic network patterns characterizing the upper Pignone catchment and the Casale valley, respectively, are most probably associated with the local litho-structural features. The geomorphic evolution of all V-shaped valleys ((b)) is strictly related to both fluvial and gravity-induced processes. On the one hand, headward erosion and slope toe scouring promote slope instability processes. On the other hand, the latter affect slopes and often involve fluvial stems increasing locally the in-channel sediment supply or evolving into debris flows. These dynamics were particularly significant during the 25 October 2011 geo-hydrological event (CitationCevasco, Brandolini, et al., 2013; CitationCevasco & Brandolini, 2015). Fluvial- and runoff-related morphogenesis is generally characterized by impulsive and discontinuous dynamics associated with severe rainfalls that may result in widespread erosion processes, intense sediment mobilization along channels and dangerous flash/debris floods (CitationCevasco, Brandolini, et al., 2013; CitationZingaro et al., 2019).
Some trough-shaped valleys modelled by fluvial, gravitational and anthropogenic processes were also mapped over the whole CTNP. Deeply-incised channel heads and gullies were identified at numerous valleys heads ((c)). Moreover, areas affected by intense surficial erosion were mapped over sparsely-vegetated or cultivated slopes. Bedrock and semi-alluvial confined channels (CitationRinaldi et al., 2015) prevail over the whole study area ((d,e)). Generally, in-channel sediments range from gravels to boulders. The main valley floors at Fegina, Monterosso and Vernazza hamlets, present small sub-flat sections made up of thin layers of fluvial deposits. Furthermore, currently-stabilized alluvial fans were mapped at some secondary valley outlets. Slopes are largely covered by eluvial-colluvial deposits, commonly from few centimetres to several metres thick ((f)). Such deposits are composed of angular, non-orientated, and unsorted pebble- and cobble-sized clasts in a silty-sandy matrix. Over the last centuries, these deposits were heavily reworked by human intervention to obtain narrow strips of land supported by dry-stone walls for agricultural purposes ((f)).
4.3. Coastal landforms and deposits
At a large scale, the general morphology of the coast shows the alternation of linear stretches along with sectors characterized by slightly pronounced headlands enclosing small bays. This coastline pattern is strongly controlled by geo-structural elements correlated to the Plio-Quaternary tectonic evolution of the Ligurian Sea, as suggested by many coastal stretches oriented according to systems of normal faults mainly NW-SE and NE-SW oriented (CitationCevasco, 2007; CitationFanucci & Nosengo, 1979). Moreover, some evidence of the combined effect of tectonic uplift and eustatic changes are revealed by flat or gently-inclined surfaces located over some ridges and occasionally hosting small hamlets (e.g. Corniglia and Volastra). These surfaces have been interpreted as relict wave-cut terraces (CitationPirazzoli, 2005), although their marine origin is still debated (CitationTerranova, 1984). Within the CTNP boundaries, the shoreline develops for about 20 km and is dominated by rocky cliffs (about 78%) and to a less extent by depositional shores (about 12%), while the remaining portions are artificial (about 10%). Between the western border of the park and the hamlet of Riomaggiore (e.g. Cala di Montenegro and Punta del Cavo headlands) the rocky coast is dominated by high vertical and sub-vertical plunging cliffs (CitationSunamura, 1992), without shore platform at the cliff-foot. These outstanding cliffs are cut in sedimentary rock masses, sometimes reaching maximum heights greater than 100 m, as at the Corniglia Promontory ((a)). Moving eastwards, rocky cliffs generally show lower steepness and height, seldom with small wave-cut platforms at the cliff base and more often with talus deposits produced by cliff retreat or coming from surficial erosion and mass movements affecting the upper coastal slopes ((b)). These deposits, mainly pebbly and gravelly, are continuously reworked by the wave action, often producing very narrow ephemeral beaches. In some cases, small beaches are produced by marine erosion acting at the toe of large ancient coastal landslides (e.g. Guvano area). However, the main beaches, albeit small in size, are fed by the main creeks (e.g. Monterosso and Vernazza) or created through past artificial nourishments (e.g. Fegina and Corniglia) ((c)). Such beaches, mainly located in the proximity of the most important coastal hamlets, are often affected by severe sea storms during the Autumn season, especially those exposed to storm surges coming from the SW directions. The most severe storms can cause serious damage to coastal engineering works (e.g. seawalls, groynes and detached breakwaters) and local bath houses (CitationMucerino et al., 2019). The rocky cliff dynamics are currently active due to the strong action of sea waves, which is more intense in the coastal stretches exposed to dominant winds blowing from SW directions and less frequently from SE sectors. The assailing force of sea waves is favoured by the geometrical and structural features of discontinuities (i.e. joints, cleavage, faults and bedding surfaces) affecting sedimentary rock masses, causing the occurrence of instability mechanisms, chiefly rock falls, topples and rock slides (CitationCevasco, 2007). In some cases, cliff edge retreat processes affecting the path of coastal hiking trails and the small beaches at the base of the unstable cliffs can represent a source of hazard for bathers and hikers ((d)).
Figure 4. Examples of typical coastal landforms and deposits in the CTNP: (a) plunging cliff at the Corniglia headland (photo from CitationRegione Liguria, 2020b); (b) active cliff at the base of coastal terraced slopes affected by intense erosional processes and mass movements along the coast east of Schiara; (c) beach fed by the Pastanelli Creek at the Monterosso hamlet (photo from CitationRegione Liguria, 2020b); (d) active edge of a retreating cliff affecting an hiking coastal trail at the headland of Punta del Persico.
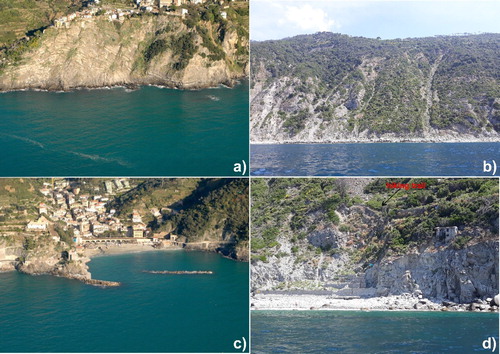
4.4. Anthropogenic landforms and deposits
Anthropogenic landforms are the most significant feature of the CTNP, contributing to create an unusual and deeply human-modified landscape. Man-made agricultural terraces constitute the main morphological element of the area. Steep coastal slopes have been modelled over the centuries in flat sections, supported by dry stone walls, disposed parallel to the contour lines to provide larger surfaces for cultivation. Moreover, extensive reaches of V-shape valleys were strongly involved in slope terracing. Local farmers built numerous river banks consolidation works along with a capillary secondary drainage network connected to the main one. Slope terracing practices established a new anthropogenic slope profile that allowed to improve runoff along with to reduce erosion processes (CitationArnáez et al., 2015). Slope portions covered by agricultural terraces sum up to 41% (about 16 km2) out of the whole CTNP area ((a)). Agricultural terraces are widely distributed from a few metres above the sea level up to altitudes comprised between 450 and 500 m, showing a different state of conservation and maintenance. About 33% of terraced areas results currently cultivated, 48% is affected by shrub covers whereas about 19% presents dense forest covers ((b)). Cultivated terraced areas are characterized by vineyards, olive and citrus groves and they are widespread near urban centres and along S-SW exposed slopes ((a,b)). Terraced areas covered by shrubs ((c,d)) can be assumed as recently abandoned while terraced lands presenting dense forest covers ((e,f)) as abandoned since decades or centuries. Abandoned terraced slopes are common in areas not easily accessible by agricultural vehicles and in the most fragmented farming properties (CitationPepe et al., 2019). Human intervention along fluvial stems is also remarkable: the final reaches of high-energy creeks were often culverted, especially when they cross coastal hamlets. In the last decades, along the upstream fluvial reaches, several geo-hydrological risk mitigation works were built ((a,b)) with the purpose of intercepting the solid charge and mass transport during intense rainfall events (e.g. debris flows barriers and check dams). Furthermore, the construction of railways and roads has determined a significant impact in terms of anthropogenic landforms. Railway fills and embankments ((c,d)) were built between 1880 and 1960 along the coastline between Monterosso al Mare (e.g. Fegina fill), Vernazza and Riomaggiore (e.g. Macereto, Rodalabia and Batternara embankments). Roads construction, started from 1950s until late 1960s, strongly influences the slope dynamics in terms of runoff water discharge and gravity-related processes. However, the abandonment of agricultural practices, and the consequent lack of maintenance of dry-stone walls and drain channels, have been playing a fundamental role in the current evolution of the landscape. This promotes the restoration of natural conditions through surficial slope instability processes that tend to level terraces (CitationBrandolini, Cevasco, et al., 2018; CitationPepe et al., 2019). Extensive portions of terraced slopes have thus become extremely vulnerable to the action of morphological processes such as landslides and mass transports, particularly during intense or prolonged rainfall (CitationCevasco, Brandolini, et al., 2013, Citation2014; CitationSchilirò et al., 2018). During these events, the geometric and stratigraphic setting of reworked slope deposits can promote the creation of a perched groundwater table starting from the contact between the bedrock and the overlying loose cover (CitationCamera et al., 2012, Citation2014; CitationCrosta et al., 2003), which in turn can trigger slope instability processes. Moreover, unmaintained drainage structures can cause the formation of new channels and thus concentrated erosion processes (CitationMoreno-de-las-Heras et al., 2019). The geomorphological trend connected to the abandonment of agricultural activities has arisen serious issues concerning land management along with producing new geo-hazard scenarios affecting hamlets, inhabitants and tourists (CitationBrandolini, Pepe, et al., 2018).
Figure 5. (a) Plot showing the percentage of CTNP territory occupied by terraced areas (TA); (b) land use/land cover of terraced areas.
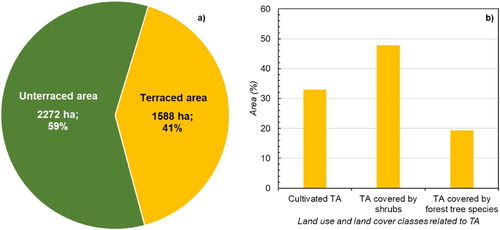
Figure 6. Examples of terraced areas in the CTNP. (a, b) Examples of cultivated vineyards at Vernazza; (c) terraced areas covered by shrubs surrounded by cultivated vineyards along the slopes in back of the Manarola village; (d) detailed view of terraced areas covered by shrubs showing dry-stone walls partially collapsed along the coastal slopes east of Riomaggiore; (e) terraced areas covered by forest tree species and cultivated vineyards along the coastal slopes east of Corniglia; (f) example of dry-stone walls in a terraced area covered by forest tree species in the proximity of Fossola.
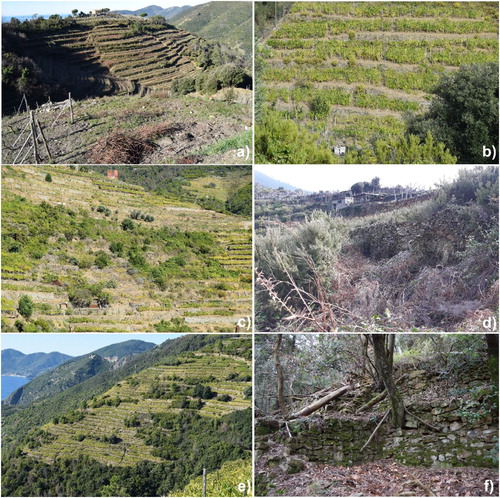
Figure 7. Geo-hydrological risk mitigation measures and engineering works in the CTNP: (a) debris flow barrier along the Vernazza Creek, at the Vernazzola area; (b) selective concrete check-dam along the Vernazza Creek, at the Vernazzola area; (c, d) railway embankments at the Macereto area (east of Vernazza) and at the Rodalabia area (east of Corniglia), respectively.
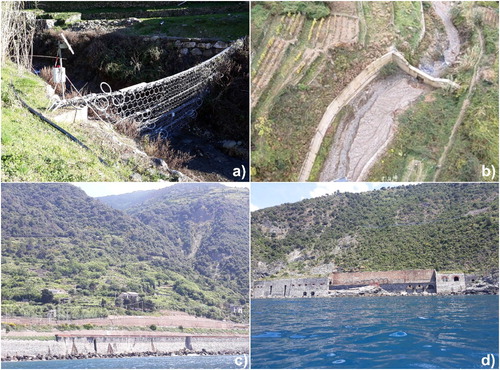
5. Conclusions
The geomorphological map of the CTNP presented in this work is the result of an integrated geomorphological analysis carried out through accurate field surveys, analysis of ancillary information and remotely sensed data. Landforms and deposits were mapped at a 1:18,000 scale to describe all geomorphological features having a major role in the dynamic of the territory. The results of this work indicated that numerous natural landforms (e.g. V-shaped valleys, sea cliffs and coastal landslides) can be source of important hazard scenarios. Particular attention has been given to the anthropogenic landforms, which strongly characterize the Cinque Terre territory. Specifically, the map deeply addresses the degree of abandonment of agricultural terraces, aiming at highlighting the connections between land-use changes and geomorphological processes that severely modified the landscape over the centuries. Indeed, terraced landscapes, when scarcely maintained, are more susceptible to erosion processes and mass movements, thus representing a major issue for the safety of people and properties. Therefore, this geomorphological map represents a significant tool for land-use policies and geo-hazard assessment that could be suitable in developing effective management strategies of landscape and cultural heritage.
Software
The geomorphological map presented in this study together with the topographic features and the related layout were developed using the free and open-source software QGIS. In the field, landforms and deposits were mapped using QField and Garmin BaseCamp GPS software.
Supplemental Material
Download PDF (49.3 MB)Acknowledgments
The authors are pleased to thank the Centro Studi Rischi Geologici (Geologic Risks Studies Center) of the Cinque Terre National Park for the useful suggestions provided during the map production and Professor Mariolina Besio for providing information and data about terraced slopes of Cinque Terre. They would like also to acknowledge the Cinque Terre National Park and its Director, Patrizio Scarpellini, for funding this research and giving logistic support during field surveys. Furthermore, the authors wish to thank the reviewers Álvaro Gómez Gutiérrez, Chandra Jayasuriya and Tommaso Piacentini, and the Editors Edoardo Rotigliano and Alessandro Chelli, for their useful comments and suggestions that helped to improve this manuscript and the related map.
Disclosure statement
No potential conflict of interest was reported by the author(s).
Additional information
Funding
References
- Abbate, E. (1969). Geologia delle Cinque Terre e dell’entroterra di Levanto (Liguria orientale). Memorie della Societa Geologica Italiana, 8, 923–1014.
- Abbate, E., Fanucci, F., Benvenuti, M., Bruni, P., Cipriani, N., Falorni, P., Fazzuoli, M., Morelli, D., Pandeli, E., Papini, M., Sagri, M., Reale, V., & Vannucchi, P. (2005). Carta Geologica d’Italia alla scala 1:50.000-Foglio 248-La Spezia con note illustrative. Regione Liguria-Dipartimento Ambiente Edilizia e Lavori Pubblici, Servizio Politiche dell’Assetto Territoriale, Genova, 204 pp.
- Agnoletti, M., Errico, A., Santoro, A., Dani, A., & Preti, F. (2019). Terraced landscapes and Hydrogeological risk. Effects of land abandonment in Cinque Terre (Italy) during severe rainfall events. Sustainability, 11(1), 235. https://doi.org/10.3390/su11010235
- Alcántara-Ayala, I. (2002). Geomorphology, natural hazards, vulnerability and prevention of natural disasters in developing countries. Geomorphology, 47(2-4), 107–124. https://doi.org/10.1016/S0169-555X(02)00083-1
- Arnáez, J., Lana-Renault, N., Lasanta, T., Ruiz-Flaño, P., & Castroviejo, J. (2015). Effects of farming terraces on hydrological and geomorphological processes. A review. Catena, 128, 122–134. https://doi.org/10.1016/j.catena.2015.01.021
- Brandolini, P. (2017). The outstanding terraced landscape of the Cinque Terre coastal slopes (eastern Liguria). In M. Soldati, & M. Marchetti (Eds.), Landforms and landscapes of Italy (pp. 235–244). Springer International. https://doi.org/10.1007/978-3-319-26194-2_20
- Brandolini, P., Cappadonia, C., Luberti, G. M., Donadio, C., Stamatopoulos, L., Di Maggio, C., Faccini, F., Stanislao, C., Vergari, F., Paliaga, G., Agnesi, V., Alevizos, G., & Del Monte, M. (2019). Geomorphology of the Anthropocene in Mediterranean urban areas. Progress in Physical Geography: Earth and Environment, 44, https://doi.org/10.1177/0309133319881108
- Brandolini, P., Cevasco, A., Capolongo, D., Pepe, G., Lovergine, F., & Del Monte, M. (2018). Response of terraced slopes to a very intense rainfall event and relationships with land abandonment: A case study from Cinque Terre (Italy). Land Degradation and Development, 29(3), 630–642. https://doi.org/10.1002/ldr.2672
- Brandolini, P., Pepe, G., Capolongo, D., Cappadonia, C., Cevasco, A., Conoscenti, C., Marsico, A., Vergari, F., & Del Monte, M. (2018). Hillslope degradation in representative Italian areas: Just soil erosion risk or opportunity for development? Land Degradation & Development, 29(9), 3050–3068. https://doi.org/10.1002/ldr.2999
- Camera, C., Apuani, T., & Masetti, M. (2014). Mechanisms of failure on terraced slopes: The Valtellina case (northern Italy). Landslides, 11(1), 43–54. https://doi.org/10.1007/s10346-012-0371-3
- Camera, C., Masetti, M., & Apuani, T. (2012). Rainfall, infiltration, and groundwater flow in a terraced slope of Valtellina (Northern Italy): field data and modelling. Environmental Earth Sciences, 65(4), 1191–1202. https://doi.org/10.1007/s12665-011-1367-3
- Campobasso, C., Carton, A., Chelli, A., D’Orefice, M., Dramis, F., Graciotti, R., Guida, D., Pambianchi, G., Peduto, F., & Pellegrini, L. (2018). Aggiornamento ed integrazioni delle linee guida della Carta geomorfologica d’Italia alla scala 1:50.000. Servizio Geologico d’Italia – ISPRA.
- Cardinali, M., Reichenbach, P., Guzzetti, F., Ardizzone, F., Antonini, G., Galli, M., Cacciano, M., Castellani, M., & Salvati, P. (2002). A geomorphological approach to the estimation of landslide hazards and risks in Umbria, Central Italy. Natural Hazards and Earth System Sciences, 2(1/2), 57–72. https://doi.org/10.5194/nhess-2-57-2002
- Cevasco, A. (2007). I fenomeni di instabilità nell’evoluzione della costa alta delle Cinque Terre (Liguria Orientale). Studi Costieri, 13, 93–109.
- Cevasco, A., & Brandolini, P. (2015). Rapid debris volume estimation by LiDAR data derived DEMs: Applications to the 25 October 2011 debris flood event at Vernazza (Cinque Terre, Italy). Rendiconti Online Società Geologica Italiana, 35, 62–65. https://doi.org/10.3301/ROL.2015.64
- Cevasco, A., Brandolini, P., Scopesi, C., & Rellini, I. (2013). Relationships between geo-hydrological processes induced by heavy rainfall and land-use: The case of 25 October 2011 in the Vernazza catchment (Cinque Terre. NW Italy). Journal of Maps, 9(2), 289–298. https://doi.org/10.1080/17445647.2013.780188
- Cevasco, A., Diodato, N., Revellino, P., Fiorillo, F., Grelle, G., & Guadagno, F. M. (2015). Storminess and geo-hydrological events affecting small coastal basins in a terraced Mediterranean environment. Science of the Total Environment, 532, 208–219. https://doi.org/10.1016/j.scitotenv.2015.06.017
- Cevasco, A., Pepe, G., & Brandolini, P. (2012). Shallow landslides induced by heavy rainfall on terraced slopes: The case study of the October 25th, 2011 event in the Vernazza catchment (Cinque Terre, NW Italy). Rendiconti Online Della Società Geologica Italiana, 21, 384–386. ISSN 2035-8008.
- Cevasco, A., Pepe, G., & Brandolini, P. (2013). Geotechnical and stratigraphic aspects of shallow landslides at Cinque Terre (Liguria, Italy). Rendiconti Online Società Geologica Italiana, 24, 52–54.
- Cevasco, A., Pepe, G., & Brandolini, P. (2014). The influences of geological and land use settings on shallow landslides triggered by an intense rainfall event in a coastal terraced environment. Bulletin of Engineering Geology and the Environment, 73(3), 859–875. https://doi.org/10.1007/s10064-013-0544-x
- Cevasco, A., Termini, F., Valentino, R., Meisina, C., Bonì, R., Bordoni, M., Chella, G. P., & De Vita, P. (2018). Residual mechanisms and kinematics of the relict Lemeglio coastal landslide (Liguria, northwestern Italy). Geomorphology, 320, 64–81. https://doi.org/10.1016/j.geomorph.2018.08.010
- Crosta, G. B., Dal Negro, P., & Frattini, P. (2003). Soil slips and debris flows on terraced slopes. Natural Hazard and Earth System Science, 3(1/2), 31–42. https://doi.org/10.5194/nhess-3-31-2003
- De Pippo, T., Donadio, C., Pennetta, M., Petrosino, C., Terlizzi, F., & Valente, A. (2008). Coastal hazard assessment and mapping in Northern Campania, Italy. Geomorphology, 97(3-4), 451–466. https://doi.org/10.1016/j.geomorph.2007.08.015
- Del Monte, M., D’Orefice, M., Luberti, G. M., Marini, R., Pica, A., & Vergari, F. (2016). Geomorphological classification of urban landscapes: The case study of Rome (Italy). Journal of Maps, 12(1), 178–189. https://doi.org/10.1080/17445647.2016.1187977
- Di Napoli, M., Carotenuto, F., Cevasco, A., Confuorto, P., Di Martire, D., Firpo, M., Pepe, G., Raso, E., & Calcaterra, D. (2020). Machine learning ensemble modelling as a tool to improve landslide susceptibility mapping reliability. Landslides, 1–18. https://doi.org/10.1007/s10346-020-01392-9
- Dramis, F., Guida, D., & Cestari, A. (2011). Nature and aims of geomorphological mapping. In Developments in Earth Surface Processes, 15, 39–73. https://doi.org/10.1016/B978-0-444-53446-0.00003-3
- Erdik, M., Biro, Y. A., Onur, T., Sesetyan, K., & Birgoren, G. (1999). Assessment of earthquake hazard in Turkey and neighboring. Annals of Geophysics, 42(6), 1125–1138. https://doi.org/10.4401/ag-3773
- Fanucci, F., & Nosengo, S. (1979). Rapporti tra neotettonica del versante marittimo dell'Appennino Ligure e margine continentale e fenomeni morfogenetici. Bollettino della Società Geologica Italiana, 96, 41–51.
- Federici, P. R., Baldacci, F., Petresi, A., & Serani, A. (2001). Atlante dei Centri Abitati Instabili della Liguria. I. Provincia della Spezia. C.N.R. G.N.D.C.I, Regione Liguria, Università degli Studi di Pisa.
- Ferrari, M., Carpi, L., Pepe, G., Mucerino, L., Schiaffino, C. F., Brignone, M., & Cevasco, A. (2019). A geomorphological and hydrodynamic approach for beach safety and sea bathing risk estimation. Science of the Total Environment, 671, 1214–1226. https://doi.org/10.1016/j.scitotenv.2019.03.378
- Furdada, G., Calderón, L. E., & Marqués, M. A. (2008). Flood hazard map of La Trinidad (NW Nicaragua). Method and results. Natural Hazards, 45(2), 183–195. https://doi.org/10.1007/s11069-007-9156-8
- Galanti, Y., Barsanti, M., Cevasco, A., D’Amato Avanzi, G., & Giannecchini, R. (2018). Comparison of statistical methods and multi-time validation for the determination of the shallow landslide rainfall thresholds. Landslides, 15(5), 937–952. https://doi.org/10.1007/s10346-017-0919-3
- Galve, J. P., Cevasco, A., Brandolini, P., Piacentini, D., Azañon, J. M., Notti, D., & Soldati, M. (2016). Cost-based analysis of mitigation measures for shallow-landslide risk reduction strategies. Engineering Geology, 213, 142–157. https://doi.org/10.1016/j.enggeo.2016.09.002
- Galve, J. P., Cevasco, A., Brandolini, P., & Soldati, M. (2015). Assessment of shallow landslide risk mitigation measures based on land use planning through probabilistic modelling. Landslides, 12(1), 101–114. https://doi.org/10.1007/s10346-014-0478-9
- Ganapathy, G. P., & Rajawat, A. S. (2012). Evaluation of liquefaction potential hazard of Chennai city, India: Using geological and geomorphological characteristics. Natural Hazards, 64(2), 1717–1729. https://doi.org/10.1007/s11069-012-0331-1
- Giammarino, S., & Giglia, G. (1990). Gli elementi strutturali della piega di La Spezia nel contesto geodinamico dell'Appennino Settentrionale. Bollettino della Società Geologica Italiana, 109, 683–692.
- Griffiths, J. S., Smith, M. J., & Paron, P. (2011). Introduction to applied geomorphological mapping. In Developments in Earth Surface Processes, 15, 3–11. https://doi.org/10.1016/B978-0-444-53446-0.00001-X
- Guerriero, L., Confuorto, P., Calcaterra, D., Guadagno, F. M., Revellino, P., & Di Martire, D. (2019). PS-driven inventory of town-damaging landslides in the Benevento, Avellino and Salerno Provinces, southern Italy. Journal of Maps, 15(2), 619–625. https://doi.org/10.1080/17445647.2019.1651770
- Gustavsson, M., Kolstrup, E., & Seijmonsbergen, A. C. (2006). A new symbol and GIS based detailed geomorphological mapping system: Renewal of a scientific discipline for understanding landscape development. Geomorphology, 77(1-2), 90–111. https://doi.org/10.1016/j.geomorph.2006.01.026
- Ho, L. T., & Umitsu, M. (2011). Micro-landform classification and flood hazard assessment of the Thu Bon alluvial plain, central Vietnam via an integrated method utilizing remotely sensed data. Applied Geography, 31(3), 1082–1093. https://doi.org/10.1016/j.apgeog.2011.01.005
- Intrieri, E., Di Traglia, F., Del Ventisette, C., Gigli, G., Mugnai, F., Luzi, G., & Casagli, N. (2013). Flank instability of Stromboli volcano (Aeolian Islands, Southern Italy): integration of GB-InSAR and geomorphological observations. Geomorphology, 201, 60–69. https://doi.org/10.1016/j.geomorph.2013.06.007
- Latocha, A. (2009). The geomorphological map as a tool for assessing human impact on landforms. Journal of Maps, 5(1), 103–107. https://doi.org/10.4113/jom.2009.1047
- Lesschen, J. P., Cammeraat, L. H., & Nieman, T. (2008). Erosion and terrace failure due to agricultural land abandonment in a semi-arid environment. Earth Surface Processes and Landforms, 33(10), 1574–1584. https://doi.org/10.1002/esp.1676
- Li, J., Yang, L., Pu, R., & Liu, Y. (2017). A review on anthropogenic geomorphology. Journal of Geographical Sciences, 27(1), 109–128. https://doi.org/10.1007/s11442-017-1367-7
- Mandarino, A., Luino, F., Turconi, L., & Faccini, F. (2020). Urban geomorphology of a historical city straddling the Tanaro River (Alessandria, NW Italy). Journal of Maps, 1–13. https://doi.org/10.1080/17445647.2020.1746420
- Mandarino, A., Maerker, M., & Firpo, M. (2019). Channel planform changes along the Scrivia River floodplain reach in northwest Italy from 1878 to 2016. Quaternary Research, 91(2), 620–637. https://doi.org/10.1017/qua.2018.67
- Mandarino, A., Pepe, G., Maerker, M., Cevasco, A., & Brandolini, P. (2020). Short-term GIS analysis for the assessment of the recent active channel planform adjustments in a widening, highly altered river: the Scrivia River, Italy. Water, 12, 514–536. https://doi.org/10.3390/w12020514
- Moreno-de-las-Heras, M., Lindenberger, F., Latron, J., Lana-Renault, N., Llorens, P., Arnáez, J., Romero-Díaz, A., & Gallart, F. (2019). Hydro-geomorphological consequences of the abandonment of agricultural terraces in the Mediterranean region: Key controlling factors and landscape stability patterns. Geomorphology, 333, 73–91. https://doi.org/10.1016/j.geomorph.2019.02.014
- Mucerino, L., Albarella, M., Carpi, L., Besio, G., Benedetti, A., Corradi, N., Firpo, M., & Ferrari, M. (2019). Coastal exposure assessment on Bonassola bay. Ocean & Coastal Management, 167, 20–31. https://doi.org/10.1016/j.ocecoaman.2018.09.015
- Ordaz, M., & Reyes, C. (1999). Earthquake hazard in Mexico City: Observations versus computations. Bulletin of the Seismological Society of America, 89(5), 1379–1383.
- Parise, M. (2001). Landslide mapping techniques and their use in the assessment of the landslide hazard. Physics and Chemistry of the Earth, Part C, 26(9), 697–703. https://doi.org/10.1016/S1464-1917(01)00069-1
- Pepe, G., Mandarino, A., Raso, E., Scarpellini, P., Brandolini, P., & Cevasco, A. (2019). Investigation on farmland abandonment of terraced slopes using multitemporal data sources comparison and its implication on hydro-geomorphological processes. Water, 11(8), 1552. https://doi.org/10.3390/w11081552
- Petley, D. N. (1998). Geomorphological mapping for hazard assessment in a neotectonic terrain. Geographical Journal, 164(2), 183–201. https://doi.org/10.2307/3060369
- Pirazzoli, P. A. (2005). Marine terraces. Encyclopedia of Coastal Science. Springer, pp. 632–633.
- Raso, E., Brandolini, P., Faccini, F., Realini, E., Caldera, S., & Firpo, M. (2017). Geomorphological evolution and monitoring of San Bernardino – Guvano coastal landslide (eastern Liguria, Italy). Geografia Fisica e Dinamica Quaternaria, 40, 197–210. https://doi.org/10.4461/GFDQ2017.40.12
- Raso, E., Cevasco, A., Di Martire, D., Pepe, G., Scarpellini, P., Calcaterra, D., & Firpo, M. (2019). Landslide-inventory of the Cinque Terre National Park (Italy) and quantitative interaction with the trail network. Journal of Maps, 15(2), 818–830. https://doi.org/10.1080/17445647.2019.1657511
- Raso, E., Faccini, F., Brandolini, P., & Firpo, M. (2016). The lovers’ lane (“Via dell’Amore”) rockfall events: A history of dangerous collapses. Rock Mechanics and Rock Engineering: From the Past to the Future, 2, 1287–1292. https://doi.org/10.1201/9781315388502-225
- Raso, E., Mandarino, A., Pepe, G., Di Martire, D., Cevasco, A., Calcaterra, D., & Firpo, M. (2018, September 17–21). Landslide inventory of the cinque terre national park, Italy. In A. Shakoor, & K. Kato, (Eds.), Slope stability: Case histories, landslide mapping, emerging technologies, proceedings of the IAEG/AEG annual meeting proceedings (Vol. 1, pp. 201–205). Springer International Publishing.
- Regione Liguria. (2020a). Piani di bacino stralcio per l'assetto idrogeologico. Piani di bacino della provincia della Spezia – Ambito 19. http://www.pianidibacino.ambienteinliguria.it/SP/spezia.html
- Regione Liguria. (2020b). Geoportale Regione Liguria. https://geoportal.regione.liguria.it/catalogo/mappe.html
- Rellini, I., Scopesi, C., Piazza, P., Olivari, S., Perrone, M., & Firpo, M. (2019). First contributions to the definition of the “cinque Terre” DOC wines Terroir (NW-Italy). Bulletin of Environmental and Life Science, 1, 60–74. https://doi.org/10.15167/2612-2960/BELS2019.1.1.1070
- Rinaldi, M., Belletti, B., Comiti, F., Nardi, L., Bussettini, M., Mao, L., & Gurnell, A. M. (2015). The Geomorphic Units survey and classification System (GUS). Deliverable 6.2, Part 4, of REFORM (REstoring rivers FOR effective catchment Management), a Collaborative project (large-scale integrating project) funded by the European Commission within the 7th Framework Programme under Grant Agreement 282656.
- Rosenfeld, C. L. (1994). The geomorphological dimensions of natural disasters. Geomorphology, 10(1-4), 27–36. https://doi.org/10.1016/B978-0-444-82012-9.50007-4
- Schilirò, L., Cevasco, A., Esposito, C., & Scarascia Mugnozza, G. (2018). Shallow landslide initiation on terraced slopes: Inferences from a physically-based approach. Geomatics, Natural Hazards and Risk, 9(1), 295–324. https://doi.org/10.1080/19475705.2018.1430066
- Scopesi, C., Olivari, S., Firpo, M., Scarpellini, P., Pini, S., & Rellini, I. (2020). Land capability classification of Vernazza catchment, Cinque Terre National Park, Italy. Journal of Maps, 16(2), 357–362. https://doi.org/10.1080/17445647.2020.1750067
- Scopesi, C., Rellini, I., Firpo, M., Schmaltz, E., Maerker, M., & Olivari, S. (2015). Assessment of an extreme flood event using rainfall-runoff simulation based on terrain analysis in a small Mediterranean catchment (Vernazza, Cinque Terre National Park). In Geomorphometry for Geosciences, J. Jasiewicz, Z. B. Zwolinski, H. Mitasova, & T. Hengl (Eds.), Adam Mickiewicz University in Poznan - Institute of Geoecology and Geoinformation, International Society for Geomorphometry (pp. 263–266). Bogucki Wydawnictwo Naukowe.
- Seeger, M., Rodrigo-Comino, J., Iserloh, T., Brings, C., & Ries, J. B. (2019). Dynamics of runoff and soil erosion on abandoned steep vineyards in the Mosel Area, Germany. Water, 11(12), 2596. https://doi.org/10.3390/w11122596
- Short, A. D. (2007). Beaches of the New South Wales coast (2nd ed., p. 406). Sydney University Press.
- Smith, M. J. (2011). Digital mapping: Visualisation, interpretation and quantification of landforms. Developments in Earth Surface Processes, 15, 225–251. https://doi.org/10.1016/B978-0-444-53446-0.00001-X
- Sunamura, T. (1992). Geomorphology of rocky coasts (Vol. 3). Wiley.
- Szabó, J., Dávid, L., & Lóczy, D. (2010). Anthropogenic geomorphology: A guide to man-made landforms. Springer.
- Terranova, R. (1984). Aspetti geomorfologici e geologico-ambientali delle Cinque Terre: Rapporti con le opere umane (Liguria orientale). Studi e Ricerche di Geografia, 7, 39–90.
- Terranova, R. (1989). Il paesaggio costiero agrario terrazzato delle Cinque Terre in Liguria. Studi e Ricerche di Geografia, 12(1), 1–58.
- Terranova, R., Brandolini, P., Spotorno, M., Rota, M., Montanari, C., Galassi, D., Nicchia, P., Leale, S., Bruzzo, R., Renzi, L., Seronello, G., Dagorne, A., Bally, E., Castex, J. M., Reynes-Trias, A., Alomar-Canyelles, G., Ferrer-García, I., Rodriguez-Gomila, R., Grimalt-Gelabert, M., & Mus-Amezquita, M. (2002). Patrimoni de marjades a la Mediterrania Occidental. Una proposta de catalogaciò. Commissiò Europea DGX, Programa Raphael, Palma Di Mallorca, Fodesma, pp 243.
- Thouret, J. C. (2010). Volcanic hazards and risks: A geomorphological perspective. In Irasema Alcántara-Ayala, & Andrew S. Goudie (Eds.), Geomorphological hazards and disaster prevention (pp. 13–32). Cambridge University Press. https://doi.org/10.1017/CBO9780511807527.003
- Twidale, C. R. (2004). River patterns and their meaning. Earth-Science Reviews, 67(3-4), 159–218. https://doi.org/10.1016/j.earscirev.2004.03.001
- van Westen, C. J., Castellanos, E., & Kuriakose, S. L. (2008). Spatial data for landslide susceptibility, hazard, and vulnerability assessment: An overview. Engineering Geology, 102(3-4), 112–131. https://doi.org/10.1016/j.enggeo.2008.03.010
- Verstappen, H. (2011). Old and new trends in geomorphological and landform mapping. Developments in Earth Surface Processes, 15, 13–38. https://doi.org/10.1016/B978-0-444-53446-0.00002-1
- Verstappen, H. T. (1992). Volcanic hazards in Colombia and Indonesia; lahars and related phenomena. In G. J. H. McCall, D. D. C. Laming, & S. C. Scott (Eds.), Geohazards: Natural and man-made (pp. 33–42). Chapman & Hall.
- Wilson, M. F., O’Connell, B., Brown, C., Guinan, J. C., & Grehan, A. J. (2007). Multiscale terrain analysis of multibeam bathymetry data for habitat mapping on the continental slope. Marine Geodesy, 30(1-2), 3–35. https://doi.org/10.1080/01490410701295962
- Zernitz, E. R. (1932). Drainage patterns and their significance. The Journal of Geology, 498–521. https://doi.org/10.1086/623976
- Zingaro, M., Refice, A., Giachetta, E., D’Addabbo, A., Lovergine, F., De Pasquale, V., Pepe, G., Brandolini, P., Cevasco, A., & Capolongo, D. (2019). Sediment mobility and connectivity in a catchment: A new mapping approach. Science of the Total Environment, 672, 763–775. doi:10.1016/j.scitotenv.2019.03.461