ABSTRACT
Fluvial and coastal landforms are indicative of landscape river delta evolution over time and provide clues for understanding coastal adjustments to sea-level and fluvial dynamics fluctuations, tectonic displacements, and extreme waves. We have mapped the surface and sub-surface footprints of fluvial and coastal geomorphological features in the Aceh River delta, northern Sumatra, using imagery dataset, vertical facies logging and helicopter electromagnetic surveys. The result is a geomorphological map at the scale of 1:75.000 which outlines the main features of the deltaic plain, including rivers, tidal and buried channels, fluvial levees, beach ridges, swales, tidal flats and lagoons. We compare their spatial distribution to the geometry of buried sediment bodies, revealed by boreholes and resistivity maps. Buried channel belts and floodplain deposits document the former locations of the distributary channels of the Aceh River. Coastal-parallel beach ridges evidence 7–8 km of asymmetric delta progradation since the mid-Holocene sea-level high stand.
1. Introduction
In the context of the current worldwide increase in population along coastlines and of rapid climate change, reconstructing the past evolution of deltaic landscapes helps predicting coastal adjustments to the current phase of sea-level rise (CitationEricson et al., 2006; CitationFanget et al., 2016; CitationStanley & Warne, 1994), to fluvial dynamic alterations (CitationSyvitski et al., 2005), to tectonics (CitationHiggins, 2016; CitationMathers & Zalasiewicz, 1999), and to extreme storm events (CitationMay et al., 2018, Citation2015; CitationAagaard et al., 2012). The spatio-temporal mapping of deltaic landforms provides insights into delta dynamics and can reveal their main controlling parameters (CitationAiello et al., 2013; CitationTarragoni et al., 2011). It also evidences the long-term evolutionary trajectories of fluvial-coastal systems (CitationAinsworth et al., 2011; CitationRossetti et al., 2015; CitationGiacomelli et al., 2018; CitationMathers & Zalasiewicz, 1999; CitationRao et al., 2015; CitationRossetti et al., 2015). So far, the main targets have been the major deltaic systems (CitationAmorosi & Milli, 2001; CitationAnthony et al., 2014; CitationCaldwell et al., 2019; CitationEricson et al., 2006; CitationKorus & Fielding, 2015; CitationStanley & Warne, 1994), because they echo to broader research interest and have global environmental significance, thus determining funding allocations. Delta studies in Indonesia have focused on historical and short-term evolutions (CitationArjasakusuma et al., 2021; CitationBeselly et al., 2021; CitationChapkanski, Brocard, Lavigne, Tricot, et al., 2022; CitationMalawani et al., 2018; CitationSeptiangga and Mutaqin, 2021). Less attention has been paid to their Holocene evolution, except in the Mahakam delta (CitationStorms et al., 2005). The need for background information regarding the long-term dynamics of these deltas is pressing, as they are now being rapidly affected by intensive land management, and can also rapidly evolve in response to volcanic eruptions (CitationBeselly et al., 2021; CitationKuenzi et al., 1979), earthquakes (CitationKelsey et al., 2015; CitationMeltzner et al., 2010; CitationPre et al., 2012) and permanent vertical displacement of the land surface, ground shaking, ground liquefaction, and tsunami landing (CitationLatief et al., 2000; CitationMonecke et al., 2008; CitationRubin et al., 2017; CitationSieh et al., 2015; CitationWassmer et al., 2015). Here, we conducted the first step study of a smaller delta in Indonesia, which long-term dynamics remains little studied.
The Aceh River delta, in Sumatra, was, by far, the delta most severely affected by the 2004 Indian Ocean tsunami, one of the largest tsunami recorded in human history (CitationDoocy et al., 2007; CitationLevy & Gopalakrishnan, 2005). In this specific context, scientific studies have been conducted to understand its recent dynamics (decennial timescales), in order to address societal (CitationScawthorn et al., 2006), sanitary (CitationPrasetiyawan et al., 2006; CitationRedwood-Campbell & Riddez, 2006) and environmental (CitationChapkanski, Brocard, Lavigne, Meilianda, et al., 2022; CitationMcLeod et al., 2010; CitationParis et al., 2009; CitationSiemon et al., 2007) issues after the tsunami. Less attention has been paid to its long-term (centennial to millennial timescales) evolution, and how such evolution influences the current response of the delta to tsunami disturbances (CitationUmitsu et al., 2007).
This study aims at delineating of the spatial distribution of various fluvial and coastal landforms in the Aceh River delta in order to: (i) provide a geomorphological map of the Aceh River delta at the scale of 1:75.000 (refer to the Main Map), (ii) provide a framework to reconstruct its evolution since the mid-Holocene sea-level high stand and (iii) identify the prevailing processes that control its geomorphological evolution and sedimentary architecture. We first provide a detailed mapping of the delta surface and then compare the spatial distribution of landforms to the geometry of buried sediment bodies revealed by resistivity maps and boreholes. Borehole data and resistivity maps are used to assess the correlation between sediment grain size and resistivity values. We finally briefly outline the Holocene evolution of the delta.
2. General context
The Aceh River delta is located five degrees north of the equator in the northernmost part of Sumatra Island (Indonesia, (A)). It lies between 0 and ∼+20 m above sea level ((B)). Based on the Ulee Lhue tide station (https://srgi.big.go.id), tides along the delta coasts are semi-diurnal with minimum neap, mean and maximum Spring tide range of 0.4, 1.2 and 2.1 m, respectively. Waves along the shoreface track from north-west from October to March, and from north-east from April to September, inducing a long-shore drift toward the north-east and south-west, respectively (CitationDiposaptono & Mano, 1998). The delta is fed mostly by the Aceh River in its central part, by small intermittent karst-sourced rivers along its southwest border, and by the minor Angan River and its tributaries along its northeastern border ((C)). The Aceh River, which is partly diverted into the Alue Naga Floodway Canal a few kilometers from its mouth, is the major freshwater source of the delta, with a catchment of 1568 km2 (CitationSyvitski et al., 2014). It originates in the Barisan Mountains ((B)), and then runs through a terraced valley for ∼130 km (CitationMontagne, 1963) before reaching the delta plain ∼20 km from the sea. The hydrological regime of the Aceh River is influenced by equatorial convective and monsoonal rainfall, with mean and maximum flood discharge at the Banda Aceh gauging station (period of 9 years) of 176 m3/s and 1700 m3/s, respectively (CitationSyvitski et al., 2014). Climate is tropical with annual temperatures ranging within 25–32°C, and high humidity (80–90%). Mean annual rainfall in the delta is ∼1600 mm/y but reaches up to 5000 mm/y in the headwaters (Barisan Mountains, CitationMuis et al., 2016; CitationPloethner & Siemon, 2006). The driest season stretches from June to July, with less than 100 mm/month. The rainy season stretches from September to March, and peaks in November and December at 300 mm/month.
Figure 1. Location map of the Aceh fluvial-deltaic environment at different spatial scales. (A) The Sumatra Island, (B), the Aceh catchment and (C) the Aceh delta. Bathymetry, altitude, hydrological network and major toponyms are reported.
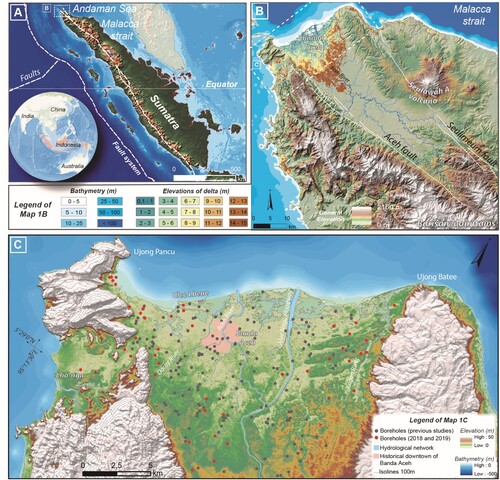
The Aceh River valley is cross-cut by two major left-lateral strike-slip faults, the Aceh fault in the southwest, and the Seulimeum fault in the northeast ((B)). They represent the north-westernmost segments of the Great Sumatra fault (CitationFernández-Blanco et al., 2016; CitationGenrich et al., 2000; CitationIto et al., 2012; CitationTabei et al., 2015). The Aceh River valley is bordered to the west by steep mountains made of massive Cretaceous limestones, and to the east by andesitic tuffs and flows, and to the south by Pliocene-Pleistocene fossiliferous tuffaceous sandstone and mixed lithic conglomerates (refer to the Main Map in Supplementary Material; CitationBennett et al., 1981; CitationCulshaw et al., 1979). The Aceh River delta has filled the drowned lower reaches of this valley during the Holocene (CitationCulshaw et al., 1979; CitationFarr & Djaeni, 1975). The thickness of Quaternary sediment fill reaches 180 m in the center part of the Aceh delta (CitationCulshaw et al., 1979).
3. Material and methods
Landforms and sub-surface sedimentary deposits of the Aceh River delta were mapped using a GIS-based approach (). The dataset () consists of (i) topographic and geological maps, (ii) aerial photographs, (iii) satellite images and (iv) digital elevation models (DEM), summarized, treated and georeferenced by CitationChapkanski, Brocard, Lavigne, Tricot, et al. (2022). In addition, this study integrates (v) bathymetric data, (vi) sub-surface vertical facies logging and (vii) helicopter electromagnetic data. To ensure the accurate overlay of each data layer, the entire dataset has been projected in WGS 84-UTM-Zone 46N. Uncertainties on georeferencing accuracy, digitizing and local hydrological context are provided in CitationChapkanski, Brocard, Lavigne, Tricot, et al. (2022).
Figure 2. Schematic demonstration of the methodological proceeding.
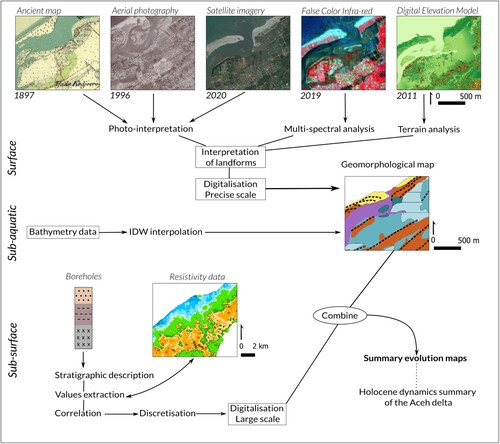
Table 1. Overview of the database used in the study.
3.1. Imagery pretreatments and feature recognitions
Sentinel-2 Multi-Spectral images at 10 m resolution () were processed to enhance the spatial contrasts in visible and near-infrared bands generated by lateral variations in soil moisture and hydric stress on vegetation (CitationHuete, 2004; CitationLillesand et al., 2015). These variations help identifying buried paleochannels (CitationGiacomelli et al., 2018). Combinations of red, green and blue (RGB normal color composite) and near-infrared, red and green (as RGB color-infrared composite) were chosen as they best reveal the landforms. Bathymetry was interpolated from sounding points (; CitationMeilianda et al., 2010) by inverse distance weighted interpolation to generate bathymetric isobaths. Hillshading of the 2 m resolution DEM was used to improve the delineation of beach ridges and dunes across the delta plain. ArcGIS Hydrology tools and 8 m resolution DEM were used to generate the drainage network and delineate the watersheds of the rivers that feed the delta. Geomorphological features were manually drawn in GIS environment in order to avoid the misclassification of automatic procedures (CitationBrandolini et al., 2020). The resulting shapefiles were assigned to the following geomorphological groups: fluvial, coastal, and marine ( and ), following an architectural classification approach (CitationAinsworth et al., 2011; CitationNanson et al., 2013; CitationVakarelov & Ainsworth, 2013). Sinuosity ratios of the palaeochannels were calculated following CitationMalavoi and Bravard (2010) and compared to the sinuosity ratios of the sub-contemporary (1884–2019) Aceh River channels, as reported in CitationChapkanski, Brocard, Lavigne, Tricot, et al. (2022).
Figure 3. Simplified version of the geomorphological map of the Aceh River delta. Field observations and snapshot orientation are displayed (). Borehole locations and associated topographic cross-sections (T1: SW – NE and T2: SE – NW) are shown ().
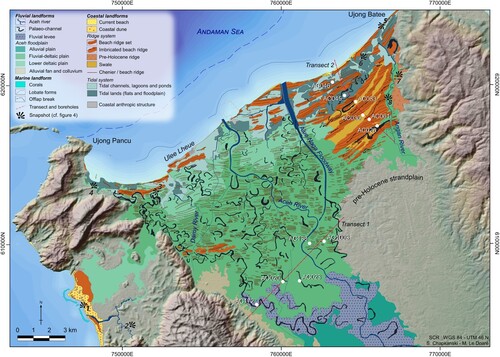
Figure 4. Fieldwork observations. Locations of snapshots are shown in . 1: White beach consisting of biogenic carbonate sands; 2: Paddy fields in the foreground and steep limestone hills in the background; 3: Dry paddy fields in former palaeochannel; 4: Tidal flats of the western delta; 5: Beach ridge and sand dunes in the eastern delta; 6: Lagoons and tidal flats downstream of the Angan River; 7: Pre-Holocene layered terraces; 8: Angan River.
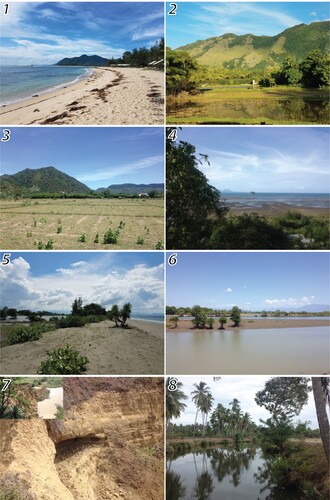
3.2. Airborne geophysical data
A helicopter-borne electromagnetic (HEM) survey of the Aceh delta was flown during the summer (from the 23th of August to the 12th of September) of 2005 by the German Federal Institute for Geoscience and Natural Resources (BGR), as part of the German-Indonesian cooperation project HELP ACEH (HELicopter Project ACEH, CitationSiemon, Röttger, et al., 2006). The project aimed finding suitable locations for drilling new freshwater wells after the Boxing Day tsunami, because electrical conductivity correlates positively with water mineralization, and therefore helps assessing the landward penetration of the coastal saline wedge (CitationPloethner & Siemon, 2006). Geophysical data processing is detailed in CitationSiemon, Röttger, et al. (2006). Here, we used resistivity maps derived from 1D inversion models of these data at 3, 4, 5, 10, 15 and 20 m below ground level (BGL, CitationSiemon, Ploethner, et al., 2006; CitationSiemon et al., 2007; CitationSiemon & Steuer, 2011). We use the resistivity maps to gain insight into the underground architecture of the delta, using the difference in resistivity between floodplains (relatively lower resistivity values of finer sediments) and sand bodies (relatively higher resistivity values of coarser sediments) such as river channels and beach ridges.
3.3. Sub-surface stratigraphy database
We dug 50 hand-auger boreholes as deep as 4 m into the Aceh plain in 2018 and 2019, targeting geomorphological elements such as palaeochannels, beach ridges, flood plains and swales, in order to provide a sub-surface validation of surface landforms. To log the boreholes, sediments were characterized in the field, based on sediment color, hand texture, hardness, the presence of oxidation, shells, diffuse organic matter, and plant remains (). Stratigraphic information published in former surveys and reports (CitationCulshaw et al., 1979; CitationFarr & Djaeni, 1975; CitationIwaco, 1993) was collected, and standardized to be compared and combined with the 2018–2019 boreholes. The entire dataset comprises 890 stratigraphic units from 206 core logs, up to 20 m long (see locations on (C)).
Figure 5. Topographic cross-sections and borehole stratigraphy. For better readability, boreholes are presented with independent scale frames from the topographic cross-sections. Resistivity values at 2, 4, 6 and 8 m below the ground level were extracted at specific core locations and reported along the boreholes.
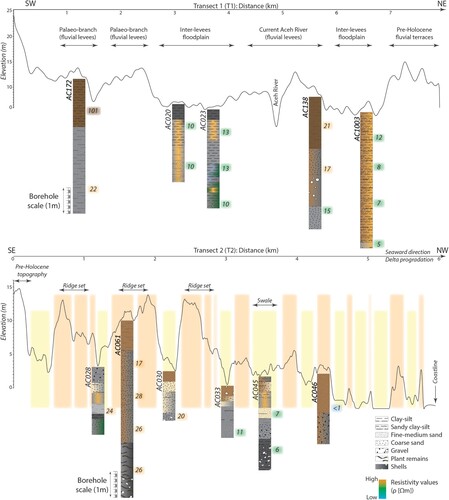
3.4. Correspondence between resistivity maps and borehole stratigraphic data
The resistivity at 3, 4, 5, 8, 10 and 20 m was extracted in GIS from the resistivity map at each borehole location. Borehole logs were then compared to the extracted resistivity values. Stratigraphic data relative to grain size were grouped into three classes: clay–silt, sand and clay-to-sand. Resistivity values are expected to vary with grain size (CitationSiemon et al., 2007). Therefore, the two data matrices (resistivity values and grain size) were combined and subjected to descriptive statistics using the software IBM SPSS statistics 20.0 (Armonk, NY, USA). Minimum, first 1st and 3rd quartiles, median and mean values were calculated to quantify the spread of resistivity values in clay–silt, sand and clay to sand units at different depths below the ground surface (). The results were used to evaluate down-depth resistivity attenuation and to select values used for the discretization of resistivity values on corresponding resistivity maps. Despite the low resolution of the maps and saltwater intrusions in the lower delta, sands in logs were found to consistently exhibit higher resistivity than silty-clays (). We therefore assumed that resistant bodies are composed of coarser sediment and we used this property to map buried sandy fluvial levees and beach ridges hosting freshwater (). Similar observations were made by CitationSiemon et al. (2007) demonstrating that the current Aceh River channel spatially correlates with relatively high values in resistivity maps. That also coincides with observations in other survey areas in Germany where low resistivity values correlate with clay–silt deposits in former lakes and higher resistivity rates with sandy to gravel deposits (CitationSiemon et al., 2020).
Figure 6. Boxplots of resistivity value distributions for specific textures and at different depths below the ground level.
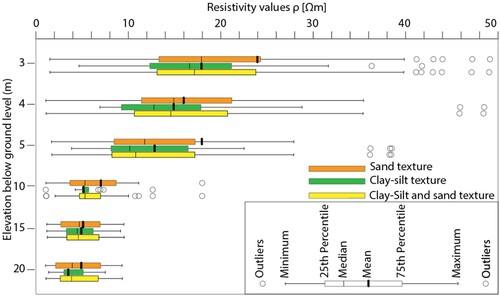
4. Results and discussion
4.1. General architecture of delta landforms
The lower delta is a ∼14-km wide coastal strip. It is made of tidal flats, lagoons, aquaculture ponds, and tidal channels (; see the Geomorphological map in Supplementary Material). Upstream, the delta plain surfaces rise up to 20 m above sea level. West and south-west of the Aceh River, we found palaeochannel belts and associated natural levee deposits that run parallel to the west delta margin, and are separated from the margin by eroded terraces rising ∼ +30 m above the delta plain, and by small alluvial fans. Irregularly spaced beach and chenier ridges, sub-parallel to the current coastline, were identified, across the delta plain, as far as 8 km inland (). East of the Aceh River in the upper delta, we identified pre-Holocene strandplain standing ∼+20 m above the Holocene delta plain and incised by a dense network of small valleys ().
4.1.1. Fluvial channels and levees.
The Aceh River currently flows along the median axis of the delta (). Its course is highly sinuous and the meanders have migrated rapidly during the twentieth Century (refer to the Main Map in Supplementary Material). The sinuosity ratio increased during twentieth century from 1.75 to 1.91 before dropping to 1.36 in 1996 (CitationChapkanski, Brocard, Lavigne, Tricot, et al., 2022) when extensive containment dykes were constructed, cutting several meanders (CitationDiposaptono & Mano, 1998). Wide palaeochannels, already abandoned by the twentieth century, are found to the north-east of the Aceh River, between the Aceh River and the Alue Naga Canal (). They were partly excavated and reused during the construction of the derivation canal. East of the Alue Naga Canal, coast-parallel paleochannels are found between the sand ridges. Their layout is reminiscent of present-day tidal channels. They connect to the Agan River, which collects water along eastern delta plain, at the toe of the volcanoclastic hills, and represents the only fluvial system east of the Aceh River that manages to cross the strand plain and reach the sea. We identified four belts of buried paleochannels and associated levees to the west of the Aceh River (). They form a series of ribbons, parallel to the modern river, that stretch over the entire delta plain. The westernmost belt lies close to the delta west margin and is highly sinuous (sinuosity index of 1.68). The other three belts show similar sinuosity but tend to take a more northerly course in the downstream direction. The boreholes dug in these paleochannels expose similar stratigraphic superpositions (-T1). Weathered silty-clay deposits fill the upper 2 m of the channels. They overlay sands showing a decreasing grain-size upward (-T1). The levees are made of weathered silty-sand (-T1). The surrounding floodplains consist of silty-clay layers that extend ∼3–5 m below the fluvial levees.
4.1.2. Beach ridges and swales
Prominent (ridgelines 10–15 m amsl) sandy beach ridges form a belt that extends from the present-day coastline to 3–6 km inland. They are separated by swales filled by silty sands ( and -T2). Imbrication of beach ridges occur ∼3–4 km inland. The height of the beach ridges and swales decreases seaward (-T2). A more localized, pronounced drop in height occurs ∼ 3 km from the shoreline, were the ridge height decreases to 3–5 m amsl. Seaward, swales then tend to be filled by shelly and clayey sandy tidal flats 0–5 m amsl (-T2).
Long-shore drift along the Aceh delta coast is predominantly to the north-east, owing to the predominance of the waves tracking from the north-west (CitationDiposaptono & Mano, 1998). Sediment drift generates coastal ridges that are concave seaward in the western delta and convex seaward in the eastern delta. Beach ridges between the Aceh River and Alue Naga mouths are truncated as a result of the erosion of a formerly more prominent river mouth which was surrounded by more cuspate shorelines, a trait typical of quickly prograding, wave-dominated deltas (CitationAnthony, 2015). The delta lobe has since been smoothed by the coastal retrogradation (CitationChapkanski, Brocard, Lavigne, Tricot, et al., 2022).
4.2. Submarine delta
The most significant morphological feature of the Aceh River prodelta is an offlap break quasi parallel to the coastline at the transition between a relatively flat topset (∼0.3°) and a steeper foreset (∼2°). North of the contemporary river mouth, the offlap break lies ∼3 km from the coastline, at a depth of ∼26 m. In the eastern delta the slope break is located farther offshore, ∼6 km from the coastline. Concave lobate forms are observed offshore and may represent the remnants of former mouths of the Aceh River.
4.3. HEM results and interpretations
The distribution of resistivity at depth is reported on , and . Low resistivity values (blue) correspond to seawater-saturated sediments of the lower delta. Sand and coarser-grained sediment filled by freshwater are represented by orange to brown pixels. Low to medium resistivity (green pixels) corresponds to silty-clay or/and coarser sediment filled by brackish water (CitationSiemon et al., 2007; CitationSiemon & Steuer, 2011). The general decrease of resistivity values with depths is probably induced by higher groundwater mineralization at depth, or/and by biases introduced by the comparison of point information in the boreholes with volume information in the electromagnetic data.
Figure 7. Resistivity maps at 20, 15 and 10 m below ground level and associated interpretations of resistivity bodies. Texture in boreholes at each specific depth are shown.
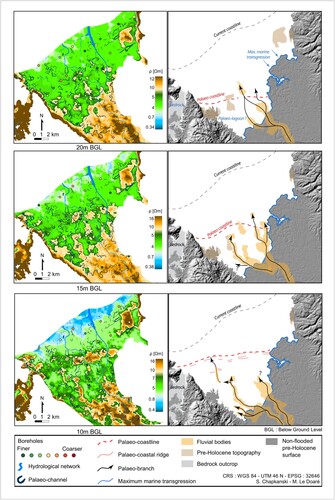
Figure 8. Resistivity maps at 5, 4 and 3 m below ground level and associated interpretations of resistivity bodies. Texture in boreholes at each specific depth is shown.
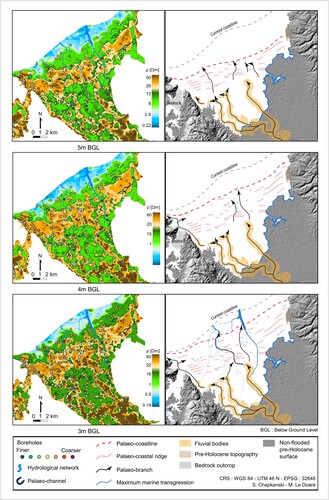
From 20 to 15 m bgl, a high resistivity belt along the valley axis seems to be composed of sand and gravel deposited by the Aceh River in its upper delta. By contrast, in the middle delta, resistive patches are aligned crosswise to the river course and may correspond to the first beach ridges to have formed after the maximum flooding of the Aceh River valley.
From 10 to 5 m bgl, some resistive patches are aligned parallel to the valley margins. They are elongate and display some sinuosity consistent with paleochannels and levees of the Aceh River. Four major SE-NW-striking belts can be clearly seen. In the middle delta, a ∼3 km wide belt of high resistivity lies parallel to the coastline and coincides with the beach ridges of the eastern delta. This belt is composed of sands that evidence the sub-surface continuation of the eastern delta ridges towards the southwest, below urbanized areas of the delta. Compared to eastern delta ridges, western delta ridges appear more discontinuous, and interrupted by the former courses of the Aceh River across the western delta. Sporadically, coast-parallel unidentified ridges are preserved, allowing us to track the beach or chenier ridges across the western delta. Intervening conductive layers are made of former inter-ridge lagoons or tidal flats and are nowadays covered by flat silty-clay floodplains.
Starting at 5–4 m bgl and above, the paleo-channel belts become fragmented, indicating that they were no longer fed by the Aceh River. In the lower delta, lobate, resistive bodies may correspond to former delta lobes beyond the former river mouths.
4.4. Processes and evolution of the Aceh River delta
At the beginning of the Holocene, the sea level of the Andaman Sea (CitationScheffers et al., 2012; CitationTjia, 1996) and Malacca strait (CitationGeyh et al., 1979) was about – 70 m below the current sea level. A marine high stand as high as + ∼ 3 m has been documented during the mid-Holocene ∼5500 years ago; it was followed by a gradual lowering to the current sea level. Based on the landward extent of the delta plain, it appears that this sea-level high stand led to a Holocene marine transgression that flooded the Aceh valley as far as 6 km inland (CitationCulshaw et al., 1979). The resistive sandy bodies buried 20–15 m below ground may belong to a transgressive tract deposited before sea level stabilized, sheltering the flooded valley farther inland from swale, thus allowing the upper valley to be filled by lagoons, swamps, or tidal flats. The Aceh River then covered this area with channel belts, fluvial levees and silty-clay to peaty floodplain deposits, as evidenced by resistivity maps above 10 m bgl. As delta progradation proceeded, the Aceh River expanded over the central part of the upper delta, feeding a series of now-buried channels, which may represent successive courses of the Aceh River or synchronous distributary channels. The courses of the most deeply imaged sand belts match the course of the modern Aceh River and of the abandoned channels still visible in the topography.
The presence of abandoned meander belts in the western part of the delta () implies that the Aceh River has flown for some time across the western delta where ridges are interrupted. The downstream terminations of the meander belts most likely correspond to former mouths of the Aceh River, which occupied the estuary, east from Ulee Lheue where beach ridges show curved shape ( and ). Layouts of the Aceh and Angan River at different time-spans (refer to the Main Map in Supplementary Material), show that, since the end of nineteenth century, both rivers have maintained the same courses across the delta (CitationChapkanski, Brocard, Lavigne, Tricot, et al., 2022). Until the end of twentieth century, the mouth of the Aceh River progressively retreated before migrating westward at the beginning of 21th century, after the destruction of the up-drift ridge.
Coast-parallel beach ridges across a strandplain as large as 7–8 km require a substantial amount of delta progradation since the mid-Holocene. As beach ridges are formed from available sediments along the coast, dispersed by the swash of waves, and that inter-ridge swales lie within the intertidal zone, the height and morphology of these features may reflect sea-level change, as well as the succession of depositional and erosive phases (CitationOtvos, 2000). Low, widely spaced beach ridges form under relatively high sediment influx and rapid vertical aggradation, by contrast to tall, closely spaced ridges, which tend to form under reduced sedimentation rates (CitationTaylor & Stone, 1996). The observed decrease in ridge and swale height seaward may indicate a lowering of sea level (CitationTaylor & Stone, 1996). Therefore, the first cluster (∼5–6 km from the coastline; -T2) of high, wide and imbricate beach ridges may reflect a relatively slow rate of delta progradation. The progressive seaward decrease in the elevation of the intervening swales may track the post-high stands lowering of sea-level since the mid-Holocene. The lower-standing and more widely spaced character of the more recent beach ridge may result from high rate of sedimentation and relatively faster delta progradation. Accelerating delta progradation may also be responsible for the arrangement of the beach ridges located around the mouth of the Aceh River (). Based on the truncated aspect of ridges and their curvature increasing seaward, the cuspate shape and asymmetric character of delta would have been more pronounced in the last visible stage of the delta progradation (). Coastal retrogradation has prevailed at least since the late of nineteenth century (∼100 m of coastal retreat during the century prior to 2004 Indian Ocean Tsunami, and ∼120 m of land loss following the tsunami events; CitationChapkanski, Brocard, Lavigne, Tricot, et al., 2022).
5. Conclusion
Mapping of landforms and buried sediment deposits in the Holocene tract of the Aceh River delta was performed using historical maps, aerial photographs, satellite images, digital elevation models and airborne electromagnetic data. Particular attention was paid to the depiction of fluvial levees, palaeochannels, tidal channels, lagoons, tidal flat, swales and beach ridges such as to provide keys for deciphering the evolution of the fluvial-deltaic system. The morphology of fluvial levees and beach ridges were documented through topographic transects. The airborne electromagnetic data was correlated with extensive in-situ stratigraphic data in order to evaluate the down-depth attenuation of silty-clay and sand layers. The results were used to provide relevant discretization of resistivity maps at specific depth layers. The landform layouts revealed some degree of consistency with the underlying buried sediments structures. The results were compiled to produce a geomorphological map of the Holocene delta of the Aceh River. The results were interpreted, and we proposed a brief discussion concerning the lines of the delta evolution in relation to the sea-level trends. Research involving radiocarbon dates, sedimentological analyses and provenance tracing is currently in progress to establish robust chronological and morpho-sedimentary frame of the Holocene evolution of the Aceh River delta.
Software
Pretreatments, feature recognition and digitizing were conducted using ArcGis Software 10.3 (ESRI, California, USA). Figures and final maps were improved using Illustrator 23.0.3 (Adobe Systems Incorporated, California, USA). Descriptive statistics were conducted using the software IBM SPSS statistics 20.0 (Armonk, NY, USA).
Supplemental Material
Download Zip (25 MB)Acknowledgements
The authors thank Cédric Bataille, Camille Tricot and Axel Heitz-Retamal for the help with the digitizing. We warmly thank Tomy Afrizal, Tarmizi, Fajarul Aulia, Rifqi Irvansyah, Junaidi Saman, Hestia Melani, Nila Kanti and Andreas Surya Adiwinata Suherman for field work logistical and technical assistance as well as Diah Novitasari from the French School for Far East Studies (EFEO) in Jakarta for the collaboration and all the help with the administrative procedure and research permit authorizations. The authors are grateful to the three reviewers (Mike Shand, Bachtiar Wahyu Mutaquin and Colin Woodroffe) for their conscientious review and feedback that helped to improve earlier versions of the manuscript. We express our gratitude to the journal editorial membership for the kind communication and careful editorial handling.
Disclosure statement
No potential conflict of interest was reported by the authors.
Data availability statement
All the data that support the findings of this study are available from the corresponding author upon reasonable request except the HEM data provided by the Federal Institute for Geosciences and Natural Resources (BGR).
Additional information
Funding
References
- Aagaard, T., Hughes, M., Baldock, T., Greenwood, B., Kroon, A., & Power, H. (2012). Sediment transport processes and morphodynamics on a reflective beach under storm and non-storm conditions. Marine Geology, 326–328, 154–165. https://doi.org/10.1016/j.margeo.2012.09.004
- Aiello, A., Canora, F., Pasquariello, G., & Spilotro, G. (2013). Shoreline variations and coastal dynamics: A space–time data analysis of the Jonian littoral, Italy. Estuarine, Coastal and Shelf Science, 129, 124–135. https://doi.org/10.1016/j.ecss.2013.06.012
- Ainsworth, R. B., Vakarelov, B. K., & Nanson, R. A. (2011). Dynamic spatial and temporal prediction of changes in depositional processes on clastic shorelines: Toward improved subsurface uncertainty reduction and management. AAPG Bulletin, 95(2), 267–297. https://doi.org/10.1306/06301010036
- Amorosi, A., & Milli, S. (2001). Late Quaternary depositional architecture of Po and Tevere river deltas (Italy) and worldwide comparison with coeval deltaic successions. Sedimentary Geology, 144(3–4), 357–375. https://doi.org/10.1016/S0037-0738(01)00129-4
- Anthony, E. J. (2015). Wave influence in the construction, shaping and destruction of river deltas: A review. Marine Geology, 361, 53–78. https://doi.org/10.1016/j.margeo.2014.12.004
- Anthony, E. J., Marriner, N., & Morhange, C. (2014). Human influence and the changing geomorphology of Mediterranean deltas and coasts over the last 6000 years: From progradation to destruction phase? Earth-Science Reviews, 139, 336–361. https://doi.org/10.1016/j.earscirev.2014.10.003
- Arjasakusuma, S., Kusuma, S. S., Saringatin, S., Wicaksono, P., Mutaqin, B. W., & Rafif, R. (2021). Shoreline dynamics in East Java Province, Indonesia, from 2000 to 2019 using multi-sensor remote sensing data. Land, 10(2), 100. https://doi.org/10.3390/land10020100
- Bennett, J. D., Bridge, D. M., Cameron, N. R., Djunuddin, A., Ghazali, S. A., Jeffery, D. H., Keats, W., Rock, N. M. S., Thompson, S. J., & Whandoyo, R. (1981). The geology of the Banda Aceh Quadrangle, Sumatra. Geological Research and Development Centre, Bandung. Explanatory note, 19 pp., and geological map, quadrangle 0421, scale 1:250,000.
- Beselly, S. M., van der Wegen, M., Grueters, U., Reyns, J., Dijkstra, J., & Roelvink, D. (2021). Eleven years of mangrove–mudflat dynamics on the mud volcano-induced prograding delta in east Java, Indonesia: Integrating UAV and satellite imagery. Remote Sensing, 13(6), 1084. https://doi.org/10.3390/rs13061084
- Brandolini, F., Reynard, E., & Pelfini, M. (2020). Multi-temporal mapping of the Upper Rhone Valley (Valais, Switzerland): fluvial landscape changes at the end of the Little Ice Age (18th–19th centuries). Journal of Maps, 16(2), 212–221. https://doi.org/10.1080/17445647.2020.1724837
- Caldwell, R. L., Edmonds, D. A., Baumgardner, S., Paola, C., Roy, S., & Nienhuis, J. H. (2019). A global delta dataset and the environmental variables that predict delta formation on marine coastlines. Earth Surface Dynamics, 7(3), 773–787. https://doi.org/10.5194/esurf-7-773-2019
- Chapkanski, S., Brocard, G., Lavigne, F., Meilianda, E., Ismail, N., Darusman, D., & Goiran, J. P. (2022). Fingerprinting sources of beach sands by grain-size, using mid-infrared spectroscopy (MIRS) and portable XRF. Implications for coastal recovery along a tsunami-struck delta coastline. CATENA, 219, 106639. https://doi.org/10.1016/j.catena.2022.106639
- Chapkanski, S., Brocard, G., Lavigne, F., Tricot, C., Meilianda, E., Ismail, N., Majewski, J., Goiran, J., Alfian, D., Daly, P., Horton, B., Switzer, A., Degroot, V., Steuer, A., Siemon, B., Cavero, J., Virmoux, C., & Darusman, D. (2022). Fluvial and coastal landform changes in the Aceh River delta (Northern Sumatra) during the century leading to the 2004 Indian Ocean Tsunami. Earth Surface Processes and Landforms, 47(5), 1127–1146. https://doi.org/10.1002/esp.5292
- Culshaw, M. G., Duncan, S. V., & Sutarto, N. R. (1979). Engineering geological mapping of the Banda Aceh alluvial basin, Northern Sumatra, Indonesia. Bulletin of the International Association of Engineering Geology-Bulletin de l'Association Internationale de Géologie de l'Ingénieur, 19(1), 40–47. https://doi.org/10.1007/BF02600445
- Diposaptono, S., & Mano, A. (1998). Proceedings of civil engineering in the ocean (Vol. 14, pp. 107–112).
- Doocy, S., Rofi, A., Moodie, C., Spring, E., Bradley, S., Burnham, G., & Robinson, C. (2007). Tsunami mortality in Aceh province, Indonesia. Bulletin of the World Health Organization, 85(4), 273–278. https://doi.org/10.2471/BLT.06.033308
- Ericson, J. P., Vörösmarty, C. J., Dingman, S. L., Ward, L. G., & Meybeck, M. (2006). Effective sea-level rise and deltas: Causes of change and human dimension implications. Global and Planetary Change, 50(1–2), 63–82. https://doi.org/10.1016/j.gloplacha.2005.07.004
- Fanget, A. S., Bassetti, M. A., Fontanier, C., Tudryn, A., & Berné, S. (2016). Sedimentary archives of climate and sea-level changes during the Holocene in the Rhône prodelta (NW Mediterranean Sea). Climate of the Past, 12(12), 2161–2179. https://doi.org/10.5194/cp-12-2161-2016
- Farr, J. L., & Djaeni, A.. (1975). A reconnaissance hydrogeological study of the Kreung Aceh Basin North Sumatra.
- Fernández-Blanco, D., Philippon, M., & Von Hagke, C. (2016). Structure and kinematics of the Sumatran fault system in North Sumatra (Indonesia). Tectonophysics, 693, 453–464. https://doi.org/10.1016/j.tecto.2016.04.050
- Genrich, J. F., Bock, Y., McCaffrey, R., Prawirodirdjo, L., Stevens, C. W., Puntodewo, S. S. O., Subarya, S. S. O., & Wdowinski, S. (2000). Distribution of slip at the northern Sumatran fault system. Journal of Geophysical Research: Solid Earth, 105(B12), 28327–28341. https://doi.org/10.1029/2000JB900158
- Geyh, M. A., Streif, H., & Kudrass, H. R. (1979). Sea-level changes during the late Pleistocene and Holocene in the Strait of Malacca. Nature, 278(5703), 441–443. https://doi.org/10.1038/278441a0
- Giacomelli, S., Rossi, V., Amorosi, A., Bruno, L., Campo, B., Ciampalini, A., Civa, A., Hong, W., Sgavetti, M., & de Souza Filho, C. R. (2018). A mid-late Holocene tidally-influenced drainage system revealed by integrated remote sensing, sedimentological and stratigraphic data. Geomorphology, 318, 421–436. https://doi.org/10.1016/j.geomorph.2018.07.004
- Higgins, S. A. (2016). Advances in delta-subsidence research using satellite methods. Hydrogeology Journal, 24(3), 587–600. https://doi.org/10.1007/s10040-015-1330-6
- Huete, A. R. (2004). Remote sensing for environmental monitoring. In J. F. Artiola, I. L. Pepper, & M. L. Brusseau (Eds.), Environmental monitoring and characterization (pp. 183–206). Academic Press.
- Ito, T., Gunawan, E., Kimata, F., Tabei, T., Simons, M., Meilano, I., Augustan, A., Ohta, Y., Nurdin, I., & Sugiyanto, D. (2012). Isolating along-strike variations in the depth extent of shallow creep and fault locking on the northern Great Sumatran Fault. Journal of Geophysical Research: Solid Earth, 117(B6), 1–16. https://doi.org/10.1029/2011JB008940
- Iwaco, B. V.. (1993). Kotamadya Banda Aceh: Planning for water supply development and raw water sources allocation. In Study of water sources allocation for water supply for D.I. Aceh province, prepared in association with COWI consulting engineers and planners AS, PT YODYA KARYA architects & consulting engineers & PT GAMMA EPSILON consulting engineers for government of Indonesia, ministry of public works, directorate general of human settlements, directorate of water supply, Banda Aceh (pp. 93).
- Kelsey, H. M., Engelhart, S. E., Pilarczyk, J. E., Horton, B. P., Rubin, C. M., Daryono, M. R., Ismail, N., Hawkes, A. D., Bernhardt, C. E., & Cahill, N. (2015). Accommodation space, relative sea level, and the archiving of paleo-earthquakes along subduction zones. Geology, 43(8), 675–678. https://doi.org/10.1130/G36706.1
- Korus, J. T., & Fielding, C. R. (2015). Asymmetry in Holocene river deltas: Patterns, controls, and stratigraphic effects. Earth-Science Reviews, 150, 219–242. https://doi.org/10.1016/j.earscirev.2015.07.013
- Kuenzi, W. D., Horst, O. H., & McGEHEE, R. V. (1979). Effect of volcanic activity on fluvial-deltaic sedimentation in a modern arc-trench gap, southwestern Guatemala. Geological Society of America Bulletin, 90(9), 827–838. https://doi.org/10.1130/0016-7606(1979)90<827:EOVAOF>2.0.CO;2
- Latief, H., Puspito, N. T., & Imamura, F. (2000). Tsunami catalog and zones in Indonesia. Journal of Natural Disaster Science, 22(1), 25–43. https://doi.org/10.2328/jnds.22.25
- Levy, J. K., & Gopalakrishnan, C. (2005). Promoting disaster-resilient communities: The Great Sumatra–Andaman earthquake of 26 December 2004 and the resulting Indian Ocean Tsunami. Water Resources Development, 21(4), 543–559. https://doi.org/10.1080/07900620500363297
- Lillesand, T., Kiefer, R. W., & Chipman, J. (2015). Remote sensing and image interpretation (p. 770) John Wiley & Sons.
- Malavoi, J. R., & Bravard, J. P. (2010). Éléments d’hydromorphologie fluviale. Onema, 228.
- Malawani, M. N., Sunarto, S., Handayani, T., & Yoga, A. G. H. (2018). Recent delta evolution based on mollusk shell record on sediment in Delta Wulan, Demak, Indonesia. Geo Media: Majalah Ilmiah dan Informasi Kegeografian, 16(2), 81–87. https://doi.org/10.21831/gm.v16i2.25204
- Mathers, S., & Zalasiewicz, J. (1999). Holocene sedimentary architecture of the Red River delta, Vietnam. Journal of Coastal Research, 15(2), 314–325. https://www.jstor.org/stable/4298944
- May, S. M., Brill, D., Engel, M., Scheffers, A., Pint, A., Opitz, S., Wennrich, V., Squire, P., Kelletat, D., & Brückner, H. (2015). Traces of historical tropical cyclones and tsunamis in the Ashburton Delta (north-west Australia). Sedimentology, 62(6), 1546–1572. https://doi.org/10.1111/sed.12192
- May, S. M., Gelhausen, H., Brill, D., Callow, J. N., Engel, M., Opitz, S., Scheffers, A., Joannes-Boyau, R., Leopold, M., & Brückner, H. (2018). Chenier-type ridges in Giralia Bay (Exmouth gulf, Western Australia)-processes, chronostratigraphy, and significance for recording past tropical cyclones. Marine Geology, 396, 186–204. https://doi.org/10.1016/j.margeo.2017.03.005
- McLeod, M. K., Slavich, P. G., Irhas, Y., Moore, N., Rachman, A., Ali, N., Iskandar, T., Hunt, C., & Caniago, C. (2010). Soil salinity in Aceh after the December 2004 Indian Ocean tsunami. Agricultural Water Management, 97(5), 605–613. https://doi.org/10.1016/j.agwat.2009.10.014
- Meilianda, E., Dohmen-Janssen, C. M., Maathuis, B. H. P., Hulscher, S. J., & Mulder, J. P. M. (2010). Short-term morphological responses and developments of Banda Aceh coast, Sumatra Island, Indonesia after the tsunami on 26 December 2004. Marine Geology, 275(1–4), 96–109. https://doi.org/10.1016/j.margeo.2010.04.012
- Meltzner, A. J., Sieh, K., Chiang, H. W., Shen, C. C., Suwargadi, B. W., Natawidjaja, D. H., Philibosian, B. E., & Galetzka, J. (2010). Coral evidence for earthquake recurrence and an AD 1390–1455 cluster at the south end of the 2004 Aceh–Andaman rupture. Journal of Geophysical Research: Solid Earth, 115(B10), 1–46. https://doi.org/10.1029/2010JB007499
- Monecke, K., Finger, W., Klarer, D., Kongko, W., McAdoo, B. G., Moore, A. L., & Sudrajat, S. U. (2008). A 1,000-year sediment record of tsunami recurrence in northern Sumatra. Nature, 455(7217), 1232–1234. https://doi.org/10.1038/nature07374
- Montagne, D. G. (1963). Het terrassenlandschap van de Atjeh vallei tussen Seulimeum en Indrapuri, bezien vanuit de lucht (No. 3). Kementerian Pertahanan, Djawatan Topografi Angkatan Darat, Instituut Geografi.
- Muis, B. A., Murtilaksono, K., Suratijaya, I. N., & Haridjaja, O. (2016). Analysis potency of water availability and water demand in Krueng Aceh watershed. International Journal of Sciences: Basic and Applied Research, 29(1), 191–201.
- Nanson, R. A., Vakarelov, B. K., Ainsworth, R. B., Williams, F. M., & Price, D. M. (2013). Evolution of a Holocene, mixed-process, forced regressive shoreline: The Mitchell River delta, Queensland, Australia. Marine Geology, 339, 22–43. https://doi.org/10.1016/j.margeo.2013.04.004
- Otvos, E. G. (2000). Beach ridges – definitions and significance. Geomorphology, 32(1-2), 83–108. https://doi.org/10.1016/S0169-555X(99)00075-6
- Paris, R., Wassmer, P., Sartohadi, J., Lavigne, F., Barthomeuf, B., Desgages, E., Grancher, D., Baumert, P., Vautier, F., Brunstein, D., & Gomez, C. (2009). Tsunamis as geomorphic crises: Lessons from the December 26, 2004 tsunami in Lhok Nga, west Banda Aceh (Sumatra, Indonesia). Geomorphology, 104(1–2), 59–72. https://doi.org/10.1016/j.geomorph.2008.05.040
- Ploethner, D., & Siemon, B. (2006). Hydrogeological reconnaissance survey in the Province Nanggroe Aceh Darussalam Northern Sumatra, Indonesia survey area: Banda Aceh/Aceh Besar 2005. Report BGR Project, HELP ACEH, Helicopter Project Aceh C, 1, 1–33.
- Prasetiyawan P., Viora, E., Maramis, E., & Keliat, B. A. (2006). Mental health model of care programmes after the tsunami in Aceh, Indonesia. International Review of Psychiatry, 18(6), 559–562. https://doi.org/10.1080/09540260601039959
- Pre, C. A. G., Horton, B. P., Kelsey, H. M., Rubin, C. M., Hawkes, A. D., Daryono, M. R., Rosenberg, G., & Culver, S. J. (2012). Stratigraphic evidence for an early Holocene earthquake in Aceh, Indonesia. Quaternary Science Reviews, 54, 142–151. https://doi.org/10.1016/j.quascirev.2012.03.011
- Rao, K. N., Saito, Y., Nagakumar, K. C. V., Demudu, G., Rajawat, A. S., Kubo, S., & Li, Z. (2015). Palaeogeography and evolution of the Godavari delta, east coast of India during the Holocene: An example of wave-dominated and fan-delta settings. Palaeogeography, Palaeoclimatology, Palaeoecology, 440, 213–233. https://doi.org/10.1016/j.palaeo.2015.09.006
- Redwood-Campbell, L. J., & Riddez, L. (2006). Post-tsunami medical care: Health problems encountered in the International Committee of the Red Cross Hospital in Banda Aceh, Indonesia. Prehospital and Disaster Medicine, 21(S1), S1–S7. https://doi.org/10.1017/S1049023X00015806
- Rossetti, D. F., Polizel, D., Cohen, S. P., & Pessenda, M. C. L., & R, L. C. (2015). Late Pleistocene–Holocene evolution of the Doce River delta, southeastern Brazil: Implications for the understanding of wave-influenced deltas. Marine Geology, 367, 171–190. https://doi.org/10.1016/j.margeo.2015.05.012
- Rubin, C. M., Horton, B. P., Sieh, K., Pilarczyk, J. E., Daly, P., Ismail, N., & Parnell, A. C. (2017). Highly variable recurrence of tsunamis in the 7,400 years before the 2004 Indian Ocean tsunami. Nature Communications, 8(1), 16019. https://doi.org/10.1038/ncomms16019
- Scawthorn, C., Ono, Y., Iemura, H., Ridha, M., & Purwanto, B. (2006). Performance of lifelines in Banda Aceh, Indonesia, during the December 2004 Great Sumatra earthquake and tsunami. Earthquake Spectra, 22(3_suppl), 511–544. https://doi.org/10.1193/1.2206807
- Scheffers, A., Brill, D., Kelletat, D., Brückner, H., Scheffers, S., & Fox, K. (2012). Holocene sea levels along the Andaman Sea coast of Thailand. The Holocene, 22(10), 1169–1180. https://doi.org/10.1177/0959683612441803
- Septiangga, B., & Mutaqin, B. W. (2021). Spatio-temporal analysis of Wulan Delta in Indonesia: Characteristics, evolution, and controlling factors. Geographia Technica, 16(Special Issue), 43–55. https://doi.org/10.21163/GT_2021.163.04
- Sieh, K., Daly, P., Edwards McKinnon, E., Pilarczyk, J. E., Chiang, H. W., Horton, B., Rubin, C. M., Shen, C. C., Ismail, N., Vane, C. H., & Feener, R. M. (2015). Penultimate predecessors of the 2004 Indian Ocean tsunami in Aceh, Sumatra: Stratigraphic, archeological, and historical evidence. Journal of Geophysical Research: Solid Earth, 120(1), 308–325. https://doi.org/10.1002/2014JB011538
- Siemon, B., Ibs-von Seht, M., & Frank, S. (2020). Airborne electromagnetic and radiometric peat thickness mapping of a bog in Northwest Germany (Ahlen-Falkenberger Moor). Remote Sensing, 12(2), 203. https://doi.org/10.3390/rs12020203
- Siemon, B., Ploethner, D., & Pielawa, J. (2006). Interpretation of electromagnetic data – Helicopter-borne geophysical investigation in the province Nanggroe Aceh Darussalam, Northern Sumatra, Indonesia. Vol. B-1, Survey area Banda Aceh/Aceh Besar 2005, Helicopter Project Aceh (HELP ACEH). BGR Report, Archive-No. 0126196 B-1, Hannover, 114 p.
- Siemon, B., Röttger, B., & Rehli, H.-J. (2006). Technical report on the helicopter-borne geophysical investigation in the province Nanggroe Aceh Darussalam, Northern Sumatra, Indonesia. Vol. A-1, Survey area Banda Aceh/Aceh Besar 2005, Helicopter Project Aceh (HELP ACEH). BGR Report, Archive-No. 0126196 A-1, Hannover, 41 p.
- Siemon, B., & Steuer, A. (2011). Airborne geophysical investigation of groundwater resources in Northern Sumatra after the Tsunami of 2004. INTECH Open Access Publisher.
- Siemon, B., Steuer, A., Meyer, U., & Rehli, H. J. (2007). HELP ACEH – A post-tsunami helicopter-borne groundwater project along the coasts of Aceh, northern Sumatra. Near Surface Geophysics, 5(4), 231–240. https://doi.org/10.3997/1873-0604.2007005
- Stanley, D. J., & Warne, A. G. (1994). Worldwide initiation of Holocene marine deltas by deceleration of sea-level rise. Science, 265(5169), 228–231. https://doi.org/10.1126/science.265.5169.228
- Storms, J. E., Hoogendoorn, R. M., Dam, R. A., Hoitink, A. J. F., & Kroonenberg, S. B. (2005). Late-holocene evolution of the Mahakam delta, East Kalimantan, Indonesia. Sedimentary Geology, 180(3–4), 149–166. https://doi.org/10.1016/j.sedgeo.2005.08.003
- Syvitski, J. P., Cohen, S., Kettner, A. J., & Brakenridge, G. R. (2014). How important and different are tropical rivers? – An overview. Geomorphology, 227, 5–17. https://doi.org/10.1016/j.geomorph.2014.02.029
- Syvitski, J. P., Kettner, A. J., Correggiari, A., & Nelson, B. W. (2005). Distributary channels and their impact on sediment dispersal. Marine Geology, 222–223, 75–94. https://doi.org/10.1016/j.margeo.2005.06.030
- Tabei, T., Kimata, F., Ito, T., Gunawan, E., Tsutsumi, H., Ohta, Y., Yamashina, T., Soeda, Y., Ismail, N., Nurdin, I., Sugiyanto, D., & Meilano, I. (2015). Geodetic and geomorphic evaluations of earthquake generation potential of the northern Sumatran fault, Indonesia. In M. Hashimoto (Ed.), International symposium on geodesy for earthquake and natural hazards (GENAH) (pp. 21–28). Springer.
- Tarragoni, C., Bellotti, P., Davoli, L., Petronio, B. M., & Pietroletti, M. (2011). Historical and recent environmental changes of the Ombrone Delta (southern Italy). Journal of Coastal Research, 61(10061), 344–352. https://doi.org/10.2112/SI61-001.36
- Taylor, M., & Stone, G. W. (1996). Beach-ridges: A review. Journal of Coastal Research, 12(3), 612–621. https://www.jstor.org/stable/4298509
- Tjia, H. D. (1996). Sea-level changes in the tectonically stable Malay-Thai Peninsula. Quaternary International, 31, 95–101. https://doi.org/10.1016/1040-6182(95)00025-E
- Umitsu, M., Tanavud, C., & Patanakanog, B. (2007). Effects of landforms on tsunami flow in the plains of Banda Aceh, Indonesia, and Nam Khem, Thailand. Marine Geology, 242(1-3), 141–153. https://doi.org/10.1016/j.margeo.2006.10.030
- Vakarelov, B. K., & Ainsworth, R. B. (2013). A hierarchical approach to architectural classification in marginal-marine systems: Bridging the gap between sedimentology and sequence stratigraphy. AAPG Bulletin, 97(7), 1121–1161. https://doi.org/10.1306/11011212024
- Wassmer, P. C., Gomez, C. A., Iskandasyah, T. Y. W. M., Lavigne, F., & Sartohadi, J. (2015). Contribution of Anisotropy of Magnetic Susceptibility (AMS) to reconstruct flooding characteristics of a 4220 BP tsunami from a thick unconsolidated structureless deposit (Banda Aceh, Sumatra). Frontiers in Earth Science, 3, 1–40. https://doi.org/10.3389/feart.2015.00040