Abstract
The insects overwhelm all other organisms of the world in species numbers and diversity. However, there are relatively few insects that inhabit the sea. Most of them are confined to the intertidal zone, with only five species of sea skaters, genus Halobates, having been successful in colonizing the open ocean. We discuss the ecology of both coastal and pelagic Halobates and the closely related genus Asclepios, focusing on their distribution ranges and adaptive strategies to marine environments accompanied by brief discussions of their biology. Updated information on the known localities for three species of Asclepios and some 40 coastal species of Halobates are presented. Many species live in vulnerable coastal habitats exposed to environmental pollution and coastal development. As a case study we trace the historical changes in populations of three Japanese sea skaters, Asclepios shiranui, Halobates matsumurai and H. japonicus, all designated as endangered species. For oceanic Halobates, we present an updated distribution map along with global current systems and sea-surface temperatures, examine interactions between distribution ranges and physical factors at the air–sea interface and discuss spatio-temporal variations in populations of each species. Finally, we infer the life history strategy of oceanic Halobates through theoretical considerations.
Published in collaboration with the University of Bergen and the Institute of Marine Research, Norway, and the Marine Biological Laboratory, University of Copenhagen, Denmark
Introduction
The insects arose during the Silurian–Ordovician period more than 420 Mya (Grimaldi Citation2009). They evolved over the next 200–300 Mya and succeeded in occupying all conceivable niches in the terrestrial world, surpassing all other organisms in species numbers and diversities. However, relatively few insects have been successful in colonizing the marine environment. Out of more than one million insect species known, only a few thousand belonging to about 20 orders are considered to be marine (Cheng Citation1976a, Citation2009; Cheng & Frank Citation1993). Most of them remained within the intertidal zone. Only five species of sea skaters, in the genus Halobates Eschscholtz, 1822, have completely left the shore and advanced into the open ocean (Cheng 1974, 1985). The genus Halobates is a member of the Gerridae (Hemiptera: Heteroptera) and contains 46 described species that spend their entire lives in a two-dimensional world at the sea–air interface.
Why there are so few insects in the sea and how only five species of Halobates were able to establish themselves in the high seas are intriguing questions that have aroused many discussions and speculations (e.g. Cheng Citation1976b; Andersen Citation1991; Ruxton & Humphries Citation2008). Throughout their evolutionary history the insects have evolved special adaptations for primarily an aerial or terrestrial existence, e.g. wings for long-distance dispersal and complete metamorphoses enabling larvae and adults to exploit different habitats thereby further extending available niches. In order to return to the sea they have to contend with various physical (turbulence, tidal movements, hydrostatic pressure change, depth), physiological (respiration, osmotic regulation) and biological (reproduction, food availability, competition, predation) factors (Cheng Citation1976b; Ward Citation1992). Although various species have been able to overcome each of these obstacles, insects are still poorly represented in the marine environment. It is likely that insects, which evolved more recently, were unable to invade the oceanic realm where crustaceans had so successfully established themselves in the Cambrian some 100 Mya earlier (Cheng Citation1976b). It is interesting to note that pelagic Halobates are the only invertebrates that live entirely at the ocean surface, the only niche left for the insects to invade (Cheng Citation1985).
In this review, we discuss the ecology of Halobates and the closely related genus Asclepios Distant, 1915. We give special reference to their distributions and adaptive strategies to their marine habitats. We will treat their biology rather briefly, as this has been covered extensively in several previous reviews (e.g. Cheng 1973a, 1985; Andersen & Cheng Citation2004).
Materials and methods
We examined the distributions of all coastal and oceanic Halobates species. For coastal species, we compiled known localities for each species and categorized them according to their distribution patterns. For oceanic species we produced an updated map integrated with relevant surface physical factors which may control their distribution ranges. We also evaluated the biology of coastal Halobates species in the context of a changing marine environment in which they are increasingly exposed to coastal development and environmental pollution. In this context we made special reference to three endangered Japanese sea skaters by tracing changes in their population and distribution for a period of several decades as examples of stresses affecting coastal species. Finally, we analysed the life-history strategies of oceanic Halobates species based on theoretical considerations.
Coastal sea skaters
Overview
The Gerridae, to which Halobates and Asclepios belong, are one of the largest families of semi-aquatic Heteroptera with over 700 described species, most of which live in freshwater habitats. At least 80 species belonging to 10 genera are known to live in various brackish or marine habitats (Cheng et al. Citation2012). A large body of information about Halobates and Asclepios is available in the literature, but hardly anything is known about the biology of any other species in the remaining eight genera.
Three species of Asclepios and some 40 species of Halobates are found in coastal areas (Andersen & Cheng Citation2004). Over 90% of the Halobates species live around tropical or subtropical island shores, four are found in temperate coastal waters in Japan, Korea and Australia, and two occur in freshwater rivers in Australia (Andersen & Weir 2004). Two subgenera of coastal Halobates can be recognized, Hilliella China, 1957 and Halobates, based on minor morphological differences (Andersen & Weir Citation1994). However, we will treat all the species as Halobates in this review.
Biology and life history
Most coastal Halobates and Asclepios known are found in habitats associated with mangrove forests or emergent coastal vegetation, as they depend largely on insects that fall onto the sea surface for food. They catch and feed on floating insects with their piercing and sucking mouthparts. Cannibalism is not uncommon and adults have often been observed to feed on younger nymphs (Andersen & Cheng Citation2004). They are preyed upon by seabirds and occasionally by marine turtles or surface feeding fish (Cheng et al. Citation2010).
Hitherto, no attempt has been successful in rearing any sea skaters from eggs to adults in the laboratory. However, through short-term laboratory studies and size measurements of field samples we found that their life histories include the egg stage, five nymphal stages and the adult stage (Cheng & Maxfield Citation1980; Cheng Citation1982; Ikawa et al. unpublished data), common to the majority of semi-aquatic Gerridae studied (Cheng Citation1966). Sea skaters are wingless throughout their life cycle. Nymphs and adults are morphologically alike. Sexes are distinguishable from the fifth nymphal instar for the majority of species (Cheng Citation1973a), but from the fourth instar for Halobates japonicus Esaki, Citation1924 (Ikawa et al. Citation2008).
Year-round studies on life cycle were carried out more recently on Halobates matsumurai Esaki, Citation1924 (A) and Asclepios shiranui (Esaki, Citation1924) in Japan (Ikawa et al. Citation2012). They have two or three generations a year and overwinter in the egg stage. In temperate regions of Japan where winter sea-surface temperature (SST) falls below 15°C, diapause would be a prerequisite. Many temperate gerrids overwinter as adults (Spence & Andersen 1992), but egg diapause is known for at least two freshwater gerrids, Metrobates hesperius Uhler, 1871 (Taylor Citation2009) and Metrocoris histrio White, 1883 (Ban et al. Citation1988). Coastal Halobates that live in tropical or subtropical waters probably breed all year round (Andersen & Cheng Citation2004).
Figure 1. Halobates matsumurai. A: Adult male. Scale = 2 mm. (Photo by A. Chiba.) B: Developing egg showing thick egg shell with red eye-spots and black egg-burster barely showing through. Scale = 1 mm. (Photo by T. Ikawa.) C: Egg shell and egg-burster left behind after hatching. (Photo by T. Ikawa.)
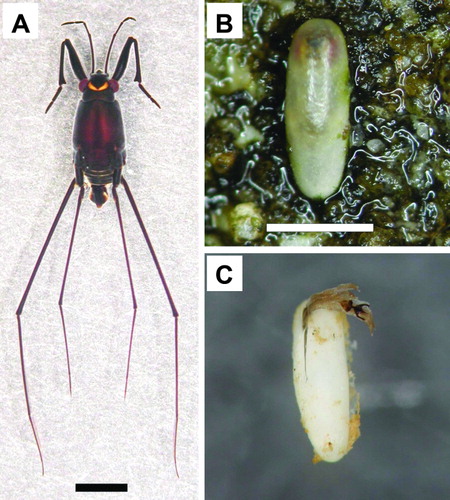
In contrast to oceanic species which lay eggs on flotsam, coastal Halobates are known to lay eggs only on solid or attached substrates (Foster & Treherne Citation1986). The eggs of H. matsumurai are laid on sandstones along the shore (B,C), mostly above mean seawater level (Ikawa et al. Citation2012). Sandstones on the shore might be the only permanent substrate available in the natural habitat of H. matsumurai where the area around the low water mark is mostly sandy. The eggs laid on the sandstones are exposed to solar radiation, wind and storms, and also to predation. As a consequence, the hard eggshell of H. matsumurai might have evolved as a special adaptation. Submature eggs in the oviducts are soft, but mature eggs are entirely covered by hard shells (B,C; Ikawa et al. Citation2012). On the other hand, Halobates fijiensis Herring, 1958, the only other coastal Halobates for which oviposition in the field was known, laid its eggs mainly on sublittoral turtle grass or green algae (Foster & Treherne Citation1986). Oviposition occurred only during days of extreme low spring tide when these plants were exposed to the air, thus the eggs would remain submerged and protected from overheating and desiccation, and also from wave action.
Life along the seashore
Adults and nymphs of coastal sea skaters live their entire lives along the shore. They generally remain under overhanging vegetation during mid-day in order to avoid damaging effects from solar radiation (Cheng et al. Citation1978). During cool autumn days in temperate Japan, they have been observed to skate in the sun (Yone et al. Citation2004). Adults and older nymphs may occasionally venture into open waters when the sea is calm while younger nymphs kept close to the shore (Cheng Citation1985).
One of the distinctive behavioural features of coastal sea skaters is the formation of aggregations in which they spend much of their time (Birch et al. Citation1979). Each aggregation usually consists of similar-sized individuals, presumably to avoid cannibalism (Ikawa Citation2009). Aggregations of the following species have been observed to maintain constant positions against currents and/or winds: Halobates robustus Barber, 1925 (Birch et al. Citation1979), Halobates fijiensis (Foster & Treherne Citation1986), Halobates japonicus (Ikawa et al. Citation2006, Citation2007a) and Halobates mariannarum Esaki, 1937 (Ikawa Citation2009) by using environmental cues such as edge of the mangrove (H. robustus) or shadows of vegetation on the sea surface (H. japonicus). These insects are constantly exposed to local currents, winds or tidal movements. Ebb tides, offshore winds or waves could wash them out to open waters where they cannot survive while onshore winds or shoreward flows may dash them against the bank or leave them stranded on the beach. Thus they need to adjust their positions constantly against changing physical forces. The size, position and composition of aggregations are known to vary according to the tidal cycle. During ebb tide aggregations were maintained close to the mangrove or to the shore and only a small number of individuals were seen in the open water. As the tide rose, aggregations became sparse and less dense, and a considerable number of individuals could be seen in the open water (Birch et al. Citation1979). These behaviours suggest that it is essential for coastal sea skaters to remain close to the shore especially during ebb tide when chances of being washed out to sea are greater.
Habitat preference
Most coastal species appear to prefer seashores fringed with mangrove or other overhanging vegetation. A survey on the endemic Halobates robustus in the Galapagos Islands has found that it occurred around four major islands: Santa Cruz, Santa Maria, Fernandina and Isabella. Individuals were present in large numbers along both sandy and rocky shores fringed with mangroves but were completely absent from shores without any rocky outcrops or mangroves (Birch et al. Citation1979). Another survey carried out on Halobates alluaudi Bergroth, 1893 in the Seychelles found them to be present on Mahé, Praslin, La Digue and the small islet Curieuse, but absent from Cousin and the small islets of Sainte Anne and Cerf. They were found, sometimes in large flotillas consisting of both adults and nymphs, only when mangroves or overhanging trees were present, and were totally absent from islands with only fringing rocks (Cheng Citation1991). Halobates matsumurai and Asclepios shiranui differed from other coastal species by living in temperate regions in Japan where there are no mangroves. Surveys carried out around two islands in Japan showed that they occurred only in narrow, protected coves with overhanging vegetation but not along sandy shores exposed directly to the open sea (Ikawa Citation2010).
Several studies suggest differences in habitat preference among coastal species. In the coasts of New Guinea and adjacent islands, several species of coastal Halobates appeared to have different habitat preference (Polhemus & Polhemus Citation2006). Polhemus (Citation1990) observed segregation of habitat among three coastal species in Aldabra Atoll. Halobates poseidon Herring, Citation1961 was mostly confined to the mangroves and never beyond 500 m off shore, H. alluaudi typically occurred inside the reef crest and almost never beyond 1000 m offshore, while Halobates flaviventris Eschscholtz, 1822 was seen mostly beyond the fore reef 500–1000 m offshore. Halobates flaviventris is more tolerant to exposed habitats than most coastal species (Cheng 1981; Polhemus Citation1990). Moreover, H. flaviventris, Halobates princeps White, 1883 and Halobates maculatus Schadow, 1922 have been collected in neuston samples at locations that are more than 150 km from shore (Cheng & Holdway Citation1983; Cheng et al. Citation1990). It is perhaps unsurprising, as this would be the necessary means for populations to disperse among islands, within archipelago or an ocean basin. In fact, this would be how pelagic Halobates species arose from nearshore ancestors as suggested by DNA analysis (Andersen et al. Citation2000). However, we are unaware whether any of the extant coastal species is able to survive and reproduce in the open ocean. Further studies on physiology, adaptation and molecular analyses would be necessary before we can determine whether any specimens caught at the high seas are able to adapt to life on the open ocean and on their way to becoming new species.
Distribution
Asclepios is clearly an Old World genus, known only from East and Southeast Asia and nowhere else: the type species Asclepios annandalei is the most widely distributed species and occurs in India, Malaysia, Singapore, Sri Lanka and Thailand; Asclepios apicalis (Esaki, Citation1924) is found in Taiwan and Vietnam; and Asclepios shiranui is known from Japan, China and Korea.
The distribution of coastal Halobates is more complex. More than 70% of the known coastal Halobates species were recorded from the Indo-Pacific Australasian region, which is probably their centre of diversity. Only two species, H. hawaiiensis and H. robustus, were recorded from the Pacific Ocean east of the International date line and seven were recorded west of the Indian subcontinent (H. alluaudi; H. formidabilis Distant, 1910; H. flaviventris; H hayanus White, 1883; H. melleus Linnavuori, 1971; H. poseidon; H. tethys Herring, Citation1961). No coastal Halobates species is known from any islands in the Atlantic Ocean, although there is an abundance of suitable habitat. Instead, we find species of Rhagovelia Mayer, 1865 (Trochopus Carpenter, 1898), a member of the Veliidae, in such habitats. Some Halobates species are known only from their type localities, some are endemic to islands or island groups, while others are widely distributed and found in locations hundreds of kilometres apart. We do not have sufficient data at present to determine whether some species are truly endemic or restricted to certain islands or island groups. As and when more collections become available, their ranges may be extended. However, based on available data in the literature and various collection records we can make the following generalizations for coastal Halobates:
Endemic
Species that are possibly endemic and known only from specific islands or discrete oceanic island groups (.1; A). Most notable in this category is Halobates robustus, which is endemic to the Galapagos Islands (Birch et al. Citation1979). The remaining species are all known either from the type locality and a few additional locations nearby, or within an island group. Dispersal of these species is likely controlled by local currents, which may serve to restrict their distributions. It is possible that some of the species may have a wider distribution as more collections become available, as evidenced by H. alluaudi. This species was described from the Seychelles and was thought to be endemic to the island group (Cheng Citation1991). However, specimens were later collected during a survey of the remote Aldabra and Cosmoledo atolls, extending its range in the Indian Ocean (Polhemus & Polhemus Citation1991).
Figure 2. A: Distributions of endemic species; Halobates acherontis, H. browni, H. bryani, H. fijiensis, H. kelleni, H. panope, H. robinsoni, H. salotae; * for ease of map plotting H. hawaiiensis with widespread distribution is included in this map while the endemic H. robustus to the Galapagos Is. and H. tethys to Mauritius are not included. B: Distributions of transoceanic species; H. flaviventris, H. hayanus.
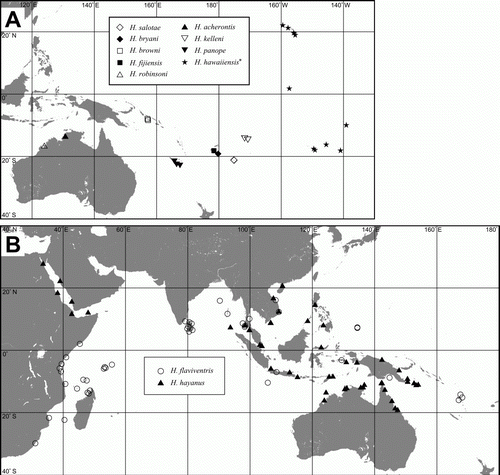
Table I. Known distributions of coastal Halobates species in the Indo-Pacific (no coastal species known in the Atlantic) (QLD, Queensland; NSW, New South Wales; NT, Northern Territories; WA, Western Australia).
Regional
Species distributed along continental shores or among islands within an archipelago (.2; A,B). Within this group are several species that are distributed between Australia and Papua New Guinea: Halobates darwini Herring, Citation1961, Halobates herringi Polhemus & Cheng, 1982 and Halobates mjobergi Hale, 1925. Two Australian species, Halobates zephyrus Herring, Citation1961 and Halobates whiteleggi Skuse, 1981, are known only from New South Wales and Queensland, while Halobate elephanta Andersen & Foster, 1992 and Halobate galatea Herring, Citation1961 are found around the coasts of the Indian subcontinent. The former has also been collected from the Andaman Sea. Halobates nereis Herring, Citation1961 is found in the West Caroline Islands.
Widespread
Species distributed within one ocean basin (.3; A,B). Most of the species in this group are found in the Pacific Ocean, only three occur in the Indian Ocean (Halobates alluaudi, H. formidabilis and H. poseidon).
Species with trans-oceanic distribution ( .4; B)
Only two species, Halobates flaviventris and H. hayanus, belong to this group. They both occur in the Pacific as well as the Indian Oceans. Halobates flaviventris probably has the widest range among coastal sea skaters. The original description of this species was cast in doubt and the type specimens have not been located. It was redescribed from specimens collected from Palau by Herring (Citation1961) and is now known from New Hebrides in the western South Pacific through Indonesia to the East African coast in the Indian Ocean (Andersen & Cheng Citation2004).
Endangered Japanese species
Most sea skaters live in coastal areas and many are now exposed to increasing development and pollution. Unfortunately, we are unable to trace population changes associated with habitat alterations due to lack of long-term studies. The only exceptions are three species in Japan on which we have considerable collection records by professional and amateur entomologists over the years. Furthermore, Japan is the only country in the world that has designated any sea skater as an endangered species.
Halobates matsumurai, Halobates japonicus and Asclepios shiranui are the only known coastal sea skaters in Japan. They were all described by Esaki (Citation1924) and were found along the western or southwestern coasts of Japan in the early days. Following rapid economic growth and industrialization after World War II (~1952–1972) much of their habitats were lost and few specimens were seen in the wild. As a result, A. shiranui was designated as an endangered species in the first Red Data Book released by the Japanese government in 1991 (Environment Agency Citation1991). All three species were later designated as endangered by the Japanese government and/or several local governments (Association of Wildlife Research & EnVision Citation2007; Ministry of the Environment Citation2007).
Asclepios shiranui was found in waterways of salt farms, estuarine waters and small coves along the westernmost coast of Honshu and the north coast of Kyushu (A) until the 1950s (Miyamoto Citation1961; Hayashi & Miyamoto Citation2003). Then, its populations began to decline. It became rare and was thought to be extinct. It was rediscovered in the north coast of Kyushu in 1996 (Hayashi & Miyamoto Citation1997) but was totally extinct in Honshu. Its present distribution is limited to northern Kyushu (A).
Figure 5. Historical range (blue) and presently known locations (red dots) of Japanese sea skaters. A: Asclepios shiranui. B: Halobates matsumurai. C: Halobates japonicus.
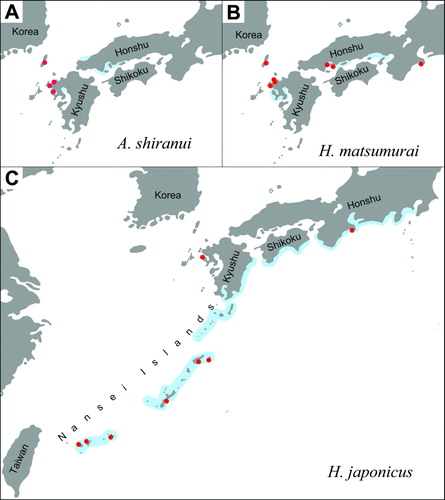
Until the 1950s, H. matsumurai was found in inner bays along the coast of northern Kyushu and western Honshu (Esaki Citation1924; Miyamoto Citation1961; B). It was rarely seen later and thought to be extinct. It was rediscovered recently in several locations in Kyushu and Honshu (Miyamoto & Hayashi Citation1996; Hayashi & Miyamoto Citation2003).
Japan's post-war high economic growth began in the 1950s. The nationwide rapid industrialization and large-scale coastal development resulted in seawater pollution which was recognized as the main cause of population decline for many species of marine animals and plants, including A. shiranui and H. matsumurai. Most salt farms along the coast had been abolished due to changes in salt manufacturing processes. It is suspected that the loss or deterioration of waterways of salt farms could also be responsible for the lack of available habitats for A. shiranui (Hayashi & Miyamoto Citation2003).
In the north coast of Kyushu, A. shiranui and H. matsumurai are often found in the same habitats (Kawachino Citation2001; Hayashi & Miyamoto Citation2003). The locations where they occur in different regions share strikingly similar geographic features. They are both found in bays or along jagged coasts of island-studded seas. Even in such areas, they occur only in deep inner coves or bays and not in shores directly exposed to the open sea (Ikawa Citation2010).
Halobates japonicus is designated as endangered by two local governments (Association of Wildlife Research & EnVision Citation2007). Changes in its distribution range are rather ambiguous compared to the other two species and little is known about its habitat preference. It was found along the Pacific coast of Honshu, Shikoku and Kyushu, and in the Nansei Islands (Esaki Citation1924; Miyamoto Citation1961; C). There were no collections from any locations along the Pacific coast for decades but a few records exist for the Nansei Islands after 1960 (Takara & Azuma Citation1972; Hayashi & Miyamoto Citation2005), probably because these remote islands were not as affected by post-war large-scale industrialization as mainland Japan. Recently, large populations of this species were found in a mangrove-fringed bay in Ishigaki, a remote subtropical island where a series of studies have been carried out (e.g. Ikawa et al. Citation2008). In Okinawa, the much-populated main island of the Nansei Islands, H. japonicus seemed rather rare (M. Moriguchi, personal communication). A small population was observed recently around mangroves in the mouth of Oura River, which is also one of the few remaining habitats in Japan for several endangered species such as dugong, blue coral, etc. (T. Ikawa, personal observation).
Most of the present habitats of the three Japanese sea skaters are in areas conserved by the government as National Parks, Quasi-National Parks, or Important Wetland 500. Starting in the 1930s, the Japanese government designated National Parks or Quasi-National Parks which must have served to preserve habitats for these insects from coastal developments and pollution. ‘Important Wetland 500’ was designated in 2001 in response to the resolution of the RAMSAR Convention. One wetland in Kyushu was included in ‘Important Wetland 500’ because the endangered A. shiranui and H. matsumurai occur there.
Among the three species, the habitat of A. shiranui is most restricted, being found only in the northern coast of Kyushu (A). Halobates matsumurai is found along the coast of northern Kyushu and western Honshu while the range of H. japonicus extends for some 1500 km from the Pacific coast of Honshu to the Nansei Islands. Obviously, they can survive only in quiet waters in protected coves, inner bays or jagged shores without coastal development or pollution (Ikawa et al. Citation2006; Ikawa Citation2010). Their present distributions are quite restricted compared to that in the 1950s or earlier.
Sea skaters and vulnerable marine environments
Field studies carried out on many tropical islands over the last 30 years (Caroline and Marshall Islands, Cook, Enewetak, Heron, Fiji, Guam, Hawaii, Hong Kong, Magnetic, Moorea, Singapore, Solomons, Tonga, etc.) have confirmed that survival of the majority of coastal Halobates species is heavily dependent upon the existence of mangroves or emergent vegetation. Although most of the locations have not been revisited, among those that were visited more recently a considerable decrease or total absence of sea-skater populations were noted (L. Cheng, unpublished). According to the World Mangrove Atlas (Spalding et al. Citation1997), mangrove habitats are largely confined between 30°N or 30°S of the equator with notable extensions in Bermuda (32°20′N), Japan (31°22′N), Australia (38°45′S), New Zealand (38°05′S) and the east coast of South Africa (32°59′S). Destruction of mangrove habitats for industrial or agricultural development has accelerated in many parts of the world in recent years. Although a number of mangrove habitats have been set aside as protected areas, destruction continues in many developing countries where most of the mangrove areas are located. This would be detrimental to the survival of coastal sea skaters. It is essential for us to understand their biology and ecology in order to develop conservation measures to preserve natural habitats for the survival of these unique marine insects before it is too late.
Oceanic species
Overview
Five species of ocean skaters, Halobates micans Eschscholtz, 1822, Halobates sericeus Eschscholtz, 1822, Halobates germanus White, 1883, Halobates sobrinus White, 1883 and Halobates splendens Witlaczil, 1886, occupy vast areas of tropical and subtropical oceans (Herring Citation1961; Scheltema Citation1968; Cheng Citation1989; Andersen & Cheng Citation2004; A). Morphological and molecular phylogenetic studies showed that the oceanic way of life has evolved at least twice from coastal life (Andersen Citation1991; Andersen et al. Citation2000; Damgaard et al. Citation2000). Although pelagic Halobates share a number of features in common with coastal species, e.g. being completely wingless and confined to the sea–air interface, going through five nymphal instars before reaching the adult stage and probably reproducing throughout the year (Cheng Citation1973b; Spence & Andersen Citation1994), they differ from coastal species in several major features which are specifically adapted for their pelagic existence. The most important is undoubtedly their ability to prevent UV damage. Living in the open ocean where no shade can be found, they are exposed to solar radiation throughout the day. Pelagic Halobates has a UV-absorbent layer in their cuticle which excludes practically all UV in the biologically damaging wavelengths between 260 and 320 nm (Cheng et al. Citation1978). Their cuticles were found to contain substantial amounts of mycosporin-like amino acids which protect them from UV damage (D. Karentz & L. Cheng, unpublished data).
Figure 6. A: Distributions of oceanic Halobates, showing negative sampling stations (white dots) as well as positive ones (H. micans, green dots; H. sericeus, blue dots; H. germanus, red dots; H. sobrinus, orange dots; H. splendens, purple dots). B: Generalized global current system superimposed on annual mean sea-surface temperature. Abbreviations are used for Agulhas Retroflection (Agulhas Ret.), Equatorial Counter Current (Eqt. Cnt. C.), Indonesia Throughflow (ITF), North Equatorial Counter Current (N. Eqt. Cnt. C.), North Equatorial Current (N. Eqt. C.), South China Sea Throughflow (SCST), South Equatorial Current (S. Eqt. C.).
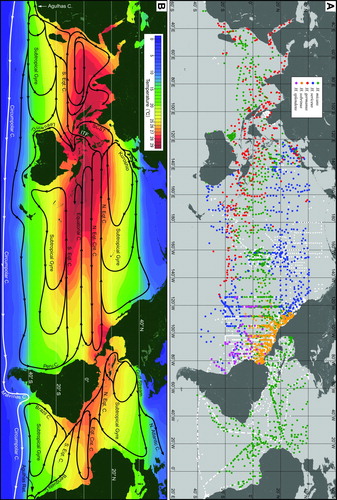
They also differ from coastal species in their feeding habits. Their main prey are small zooplankton organisms trapped at the sea surface, but they may also feed on terrestrial or migratory insects that fall on the sea (Cheng & Birch Citation1977), whereas coastal species depend entirely on terrestrial insects for food. Unlike coastal species, they lay eggs on floating materials (Cheng Citation1973a) such as sea shells, bird feathers, tar lumps, nylon ropes, bits of plastics, etc. (B,C), because solid, attached substrates are unavailable in the ocean. It is interesting to note that the freshwater gerrid Metrobates hesperius has been observed to lay eggs on floating leaves which may serve as a means of dispersal (Taylor Citation2009).
Figure 7. A: Mating pair of Halobates micans. (Photo by M. Sasaki) B: Eggs of oceanic Halobates on seabird feather. Scale = 2 mm. (Photo by S. Koyachi) C: Eggs of oceanic Halobates on nylon rope. Scale = 2 mm. (Photo by S. Koyachi)
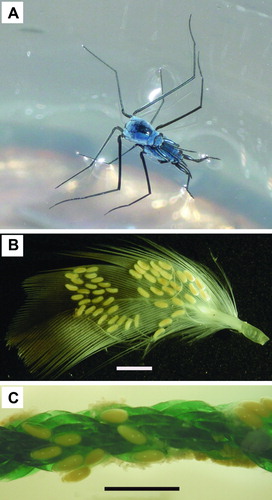
Compared to coastal species, bodies of nymphs and adults of oceanic Halobates are more rounded and the legs are shorter, presumably an adaptation for living on the ocean surface where winds and waves are frequently encountered. Their bodies are covered by a layer of microtrichia which traps air and prevents them from drowning (Cheng Citation1973c). Hairs on their mid-femur and tibia are also longer and much denser, presumably making them more efficient for long-distance skating on the rough seas (Miyamoto & Senta Citation1960).
Distribution ranges and oceanic pathways
Ocean skaters are found roughly between latitudes 40°N and 40°S of all three major oceans (A), an area larger than the total land area of the world. Thus, they have the widest distribution range among the Insecta. In this section we examine how the range of each species may be influenced by physical factors such as SST, ocean circulation and prevailing winds, and how far and where Halobates could skate with or against such forces. A newly constructed map (A) is presented and potential oceanic pathways for their long-range dispersal are discussed.
Although ocean skaters are known to occupy tremendously wide areas of the major oceans, certain regions are seldom surveyed or even visited by oceanographic cruises. Thus, there is always some uncertainty in determining limits of their distribution ranges. To recognize the true distribution ranges, we need to have negative as well as positive surface tow records covering the entire World Ocean, which is, of course, impossible. We were able to obtain considerable data sets from all available sources for the central and eastern North Pacific, but data are largely lacking from many other regions, especially the central South Pacific and central South Indian Oceans. In our map we have included both positive and negative tow records from all available data sets, whereas only positive tows were used for compiling previous distribution maps (Cheng Citation1974, 1989; Andersen & Cheng Citation2004).
Distribution of Halobates micans
Halobates micans (A) is the only cosmopolitan species living in all three major oceans and is also the only species found in the Atlantic Ocean. It occupies the warm equatorial region, but has different latitudinal ranges in each ocean basin. In the North Atlantic it has been found at ca. 40°N, the highest latitude recorded. However, this may not be its northern limit, because we do not have data beyond 40°N (A). The Gulf Stream which branches at about 38°30′N and 44°W (Mann Citation1967) could possibly carry individuals further north in its northward branch, the North Atlantic Current (B). Ring-like eddies shed from the Gulf Stream (Saunders Citation1971) could also facilitate further dispersal of H. micans northwards in warm core rings or southwards into the Sargasso Sea in cold core rings. Thus, the Gulf Stream, combined with other surface currents would be able to transport H. micans within the North Atlantic Subtropical Gyre.
Within the equatorial Atlantic H. micans could be dispersed westward by the Equatorial Current and the trade winds or eastward by the Equatorial Counter Current. However, there is a conspicuous gap off the northeastern coast of Brazil (ca. 0–10°N and 30–60°W) where it is absent, as evidenced by many negative tows (A). This region is characterized by frequently observed patches of low-salinity surface waters (Hu et al. Citation2004) resulting from freshwater discharged from the Amazon and Orinoco Rivers which have the largest rate of discharge in the World (Berner & Berner Citation1987).
In the South Atlantic, especially south of 20°S, our data are insufficient for determining conclusive boundaries. It is likely that H. micans extends to ca. 40°S only along the South American coast. This extension could be attributed to the west boundary Brazil Current that flows along the coast of Brazil (B). Cool water carried along the coast of Argentina by the Malvinas Current northward until it reaches the Brazil Current could act as a barrier for dispersal of H. micans further south.
In the central and eastern South Atlantic H. micans is probably limited to ca. 20°S. In fact, none was caught in 68 tows along a cruise track from Rio de la Plata (38°S) to Cape Town (34°S) (Cheng & Shultz-Baldes Citation1981). The SST in the eastern South Atlantic is lower compared to the western South Atlantic (B), owing to the Benguela Current which carries cold water around the Cape of Good Hope and the west African coast. Combined with strong northward winds (Hellerman & Rosenstein Citation1983; Josey et al. Citation2002) it would be difficult for H. micans to penetrate southward. Thus, in the South Atlantic it is confined mainly within the South Equatorial and Equatorial Counter Currents with an extension along the Brazil Current.
In the Pacific Ocean H. micans is largely confined within a zone between latitudes 15°N and 15°S, bounded by the North and South Equatorial and the Equatorial Counter Currents (A). This is much narrower than that in the Atlantic. We do not think SST alone is responsible for this narrower distribution as there were no notable differences between the two areas (B). Its absence is likely due to the presence of another species, H. sericeus, in higher latitudes. Within this zone, the North and South Equatorial and Equatorial Counter Currents as well as trade winds could facilitate trans-Pacific migration of H. micans. Beyond this zone its range extends northwards to ca. 25°N along the North American coast, and to ca. 30°N along the Japanese coast. The latter was attributed to the strong Kuroshio Current that flows northeastward from the Philippines.
In the Indian Ocean our data are limited, especially from the South Indian Ocean and the Arabian Sea. Halobates micans is mainly found between 8°N and 10°S, the narrowest zone among three oceans. SST is unlikely to form any barrier because it is quite high in most parts of the Indian Ocean (B). The Agulhas Current could be responsible for the southern range extension of H. micans along the African coast and the Leuween Current for its presence along the Australian coast (A).
It should be noted that around the Malay Archipelago, collections of H. micans are quite limited compared to Halobates germanus. In the Sulu, Celebes and Java Seas and the Bay of Thailand only H. germanus has been collected (A). In the Banda Sea both species were caught but most of the specimens were H. germanus (Cheng et al. Citation1990).
Distribution of Halobates germanus
Halobates germanus occurs in the Indian and the western and central Pacific Oceans (A). In the Indian Ocean, it is found in the Arabian Sea, the Bay of Bengal, and Central and South Indian Ocean as far as 10°S. The southern extension along the African coast could be attributed to the Agulhas Current, as is also the case for Halobates micans. It also occurs in the Red Sea, the Gulfs of Aden, Oman and the Andaman Sea where H. micans has never or rarely been caught. In the Pacific Ocean, H. germanus is found off the coasts of Melanesia, Micronesia and Polynesia but not the Hawaiian Islands. It is absent east of 125°W as evidenced by numerous negative data. This longitude is also the easternmost location of Polynesia, where strong westward winds blow (Hellerman & Rosenstein Citation1983; Josey et al. Citation2002). Strong winds and lack of island coasts might prevent H. germanus from invading the eastern Pacific. In contrast to other species it appears to prefer areas close to islands or margins of continents. It was also frequently collected in many areas of marginal seas and straits in the Malay Archipelago, suggesting its stronger affinity to coastal environments. Moreover, several adults were collected, skating among aggregations of the coastal Halobates japonicus in an inner bay of Nansei Islands (Ikawa et al. Citation2008).
Distribution of Halobates sericeus
Halobates sericeus is only found in the Pacific Ocean where it has an amphi-tropical distribution with populations living north (10–40°N) and south (10–40°S) of the equatorial zone occupied by H. micans (A). As a considerably wide area (20–40°S and 120–170°W) in the South Pacific has rarely been surveyed, we could not determine whether it is really absent in this area. It appears to live within the subtropical gyres in the North and South Pacific Ocean and can thus be expected to circulate widely with currents in the gyres.
Distributions of Halobates sobrinus and Halobates splendens
Halobates sobrinus and H. splendens have relatively narrow distribution ranges confined to the eastern tropical Pacific Ocean (A). Halobates sobrinus occupies an area between ca. 5°S–30°N and ca. 120–80°W off the coast of Mexico and Central America within the Intertropical Convergence Zone where the water is quite warm (B) and the wind stress is very low (Hellerman & Rosenstein Citation1983; Josey et al. Citation2002).
Halobates splendens occurs off the coast of Central and South America, between ca. 30°S–10°N and ca. 120–70°W (A). Off the Peru coast, SST and salinity are lower during upwelling. This species must have adapted to this annual fluctuations in temperature and salinity. There are no distinct recursive winds or currents in this region (B; Hellerman & Rosenstein Citation1983; Josey et al. Citation2002). Consequently, H. splendens is continuously exposed to northwestward winds and currents. This might account for its low density compared to the other three species (H. micans, H. sericeus and H. sobrinus) which can be found in the same area (Cheng & Shulenberger Citation1980; ).
Table II. Average densities of Halobates in various oceanic regions.
Trans-oceanic and intra-oceanic migration pathways
There exist three possible trans-oceanic pathways that would allow gene flow among populations of Halobates micans and/or of Halobates germanus. First, H. micans could be transported by the Agulhas Current from the Indian Ocean to the South Atlantic Ocean. This current flows southward along the African coast and turns to the east from the tip of South Africa, forming the Agulhas Retroflection (B). Rings shed from the latter are injected into the South Atlantic Ocean (Gordon Citation2003) and may carry H. micans with them. Second, the poleward Leeuwin Current (Cresswell & Golding Citation1980) which flows along the west coast of Australia and continues along its south coast to the Tasman Sea (Ridgway & Condie Citation2004) could be a carrier from the Indian Ocean to the Pacific Ocean (B). Halobates micans collected off the west Australian coast down to ca. 35°S might suggest this possibility. Third, H. micans and H. germanus could migrate from the western Pacific Ocean via the Indonesia Throughflow (Lukas et al. Citation1996) or the South China Sea Throughflow (Qu et al. Citation2005) to the Indian Ocean (B). In fact, H. germanus is frequently found in many areas of marginal seas and straits in the Malay Archipelago (A). On the other hand, collection records suggest rather limited migration of H. micans from the western Pacific into the Indian Ocean (A). There seems to be no direct pathway between the Atlantic and the Pacific Oceans because two cold currents, the Peru and the Malvinas that flow northwards along the western and eastern South America, would prevent them from migrating southward (B). In addition, the Drake Passage would be too cold and too rough for Halobates to skate through.
In spite of the existence of potential trans-oceanic pathways, results of DNA analysis suggest limited gene flow among populations of H. micans or H. germanus from different oceans (Andersen et al. Citation2000). Transit through the three potential trans-oceanic pathways would not have been frequent enough to achieve complete mixing of different populations. However, these pathways could certainly carry ocean skaters to some extent, allowing gene flow and preventing allopatric speciation.
We do not know to what extent the northern and southern populations of H. sericeus are able to cross the equatorial zone occupied by H. micans. Results from molecular analyses suggest very limited gene flow between the two populations (Andersen 2000; Leo et al. Citation2012). However, specimens collected close to the equator in the central and eastern Pacific Ocean (about 165–145°W, 130°W, 110°W, 80°W in A) suggest that it could penetrate into the habitat of H. micans. In fact, along the northern and southern ranges of H. sericeus, prevailing trade winds are known to converge towards the equator, thus able to carry the insects across. Cheng (Citation1997) suggested that trans-equatorial migration was possible during seasonal weakening of zonal currents. Therefore, if migration is limited between northern and southern populations, there must exist some other factors to keep the two populations apart. Higher SST in the equatorial zone may not be a barrier because H. sericeus appears to have a high temperature tolerance (Ikawa et al. Citation2002). Lower salinity might be a possibility because in general H. sericeus is found in saltier waters in subtropical gyres and has been found to shift its distribution towards the equator following the salinity front (Ikawa et al. Citation2002). Biological competition with H. micans is another possibility (Cheng Citation1985).
Do distribution ranges overlap?
The distribution map (A) presented here is compiled from various collection records accumulated over many years. We found large areas of overlap in the following three regions: (1) eastern tropical Pacific Ocean (4 species; Halobates micans, H. sericeus, H. sobrinus and H. splendens); (2) western Pacific Ocean (ca. 10–35°N) between the westernmost area of the North Equatorial Current and the Kuroshio (3 species; H. micans, H. sericeus and H. germanus); (3) Indian Ocean between ca. 10°S and 10°N (2 species; H. micans and H. germanus).
In the eastern tropical Pacific Cheng & Shulenberger (Citation1980) showed that even though two or more species were occasionally collected in the same net, usually only one species dominated numerically. Thus, areas of high density of each species did not overlap. In the westernmost areas of the North Pacific Equatorial Current, results of three cruises conducted in different years revealed that H. micans was predominant in two cruises. However, it was almost completely replaced by H. sericeus during the third cruise (Ikawa et al. Citation2002). The southward shift of salinity front related to the El Nino event (Kimura et al. Citation2001) might have been responsible for transporting H. sericeus southwards with saltier water moving from the north. In the Indian Ocean, distribution overlap would also be uncommon. During the circumnavigational cruise of ‘Operation Drake’, no tow contained both species. Halobates germanus was caught only in the Gulf of Aden and the Red Sea while H. micans occurred exclusively in the open stretch of the ocean (Cheng & Holdway Citation1983). In the eastern South Indian Ocean, a total of 1190 individuals sampled in 221 tows contained no H. germanus but were all H. micans (Ikawa et al. Citation2007b). Seasonal variations of winds and currents in the Indian Ocean might cause seasonal shifts in the distribution of Halobates (Ikawa et al. Citation2007b), generating apparent overlaps in the map.
Co-occurrence and spatio-temporal variations
So far, the only region where two or more species are known to co-occur in comparable densities is along the Kuroshio Current between the southeastern East China Sea and the southern Pacific coast of Japan (Ikawa et al. Citation2004). In the North Pacific, Halobates micans and H. germanus are usually found south of the habitat zone of H. sericeus (A). The Kuroshio originates east of the Philippines and flows across the habitats of both H. micans and H. germanus, possibly transporting them to the north. Eddies generated by frontal disturbances of the Kuroshio (Kimura et al. Citation1997) may provide heterogeneous environments for these species to coexist in a narrow area (1000–6000 m2) that can be covered by a single net tow. However, H. micans and H. germanus may not be carried totally passively within the Kuroshio. Each species appears to have its own habitat preference. Higher numbers of H. micans were found along the Pacific coast of Japan where the main stream of the Kuroshio flows while H. germanus was more abundant within the branch of the Kuroshio in the East China Sea. This difference could be caused by the difference in volume transport between the main stream of the Kuroshio and its branch. Halobates micans appeared to be more closely associated with warm waters of the Kuroshio than H. germanus.
Temporal changes in species composition in the East China Sea were possibly due to different temperature preferences among the three species (Ikawa et al. Citation2004). In the East China Sea, when the SST was lowest (23.2°C), the most common insects caught were H. sericeus. When SST was intermediate (27.6°C), H. sericeus was predominant followed by H. germanus while H. micans was most abundant followed by H. germanus when SST was highest (28.9°C). Halobates micans appeared to be abundant in this area and off the Japanese coast only during the summer when SST was quite high (T. Ikawa, unpublished data), suggesting that it is least tolerant to low SST.
Most ocean skaters are caught in warm waters where SST is above 20°C; a few collected in cooler waters have been considered strays (Andersen & Cheng Citation2004). In fact, the distribution of H. micans was found to be limited by the 21°C winter isotherm in the Atlantic Ocean (Scheltema Citation1968). They were rarely caught off the south coast of Japan during winter when SST falls below 20°C except in the main stream of the Kuroshio (Miyamoto & Senta Citation1960; T. Ikawa, unpublished data).
Oceanic Halobates were thought to reproduce all year round (Cheng Citation1973a; Spence & Andersen Citation1994). Most females sampled off the Japanese coast in autumn were gravid. Many eggs were also found on floating materials and some were obviously ready to hatch (T. Ikawa, unpublished data). Their numbers decreased toward winter and we do not know where the females or eggs went. In May, population began to recover, H. sericeus being the earliest to be caught in any numbers (T. Ikawa, unpublished data). Thus, the abundance of Halobates appears to fluctuate seasonally not only in this region but probably also in other areas where SST falls below 20°C in winter. The 20°C isotherm shifts annually ca. 1000 km along the meridian (see .4 in Open University Citation1989). We can therefore expect the northern or southern limits of H. sericeus in the Pacific or of H. micans in the Atlantic to shift seasonally about 1000 km northwards in boreal summer and southwards in boreal winter.
Theoretical aspects of population structure and life-history strategy
Physical forces, such as winds or currents, must play decisive roles in determining the population structure and life history of ocean skaters that live exclusively at the air–sea interface. Hitherto, the only theoretical study on the influence of any physical factor on Halobates was that by Ikawa et al. (Citation1998). They considered oceanic diffusion as an integrated effect of all physical forces. In the following three sections, we delve further into the effects of oceanic diffusion on ocean skaters, discuss theoretical inferences with results from DNA analysis (Andersen et al. Citation2000), and illuminate their unique adaptive strategy to live at the ocean surface.
Oceanic diffusion operating on a patch of ocean skaters
The term diffusion usually refers to molecular diffusion. Oceanic diffusion, however, is quite different from molecular diffusion (Okubo Citation1980). First, molecular diffusion is microscopic, caused by thermal movements of molecules. Oceanic diffusion, on the other hand, is caused by turbulence that consists of various-sized eddies. The worldwide prevailing winds and tide-generating forces give energy to large-scale eddies, and this energy is transferred to smaller eddies. Because these eddies carry rather high energy, oceanic diffusion is much more powerful than molecular diffusion. Second, while diffusivity, i.e. diffusion coefficient, is constant in molecular diffusion, it increases with the scale of the phenomenon in oceanic diffusion because more numbers of larger eddies are involved with the increase of the scale. This means oceanic diffusion operates more powerfully as the scale of phenomena increases. Therefore, oceanic diffusion must greatly influence ocean skaters that have wide distribution ranges across the ocean.
Based on the empirical data, Okubo (Citation1971, Citation1974) constructed two kinds of oceanic diffusion diagrams, i.e. horizontal variance versus diffusion time and the diffusivity versus the scale of diffusion. This is formalized as (Ikawa et al. Citation1998)
where σ 2 (cm2) is the horizontal variance of a diffused dye patch and t (s) is the time after its release on the ocean (Okubo Citation1971). Equivalently, the diffusivity K a (cm2 s−1) is given as
We set 1.5σ as the radius of a Halobates patch, because 67.5% of the population is included within this radius, provided the distribution is two-dimensional Gaussian. Then, our calculations show that oceanic diffusion could increase the radius of a Halobates patch as much as 3500 km in 120 days (Ikawa et al. Citation1998). This distance is equivalent to about one-fourth of the maximum distribution range of Halobates micans in the Pacific Ocean. Starting from the egg stage, ocean skaters could live for more than 4 months (Cheng Citation1985; L. Cheng, unpublished data). Then, individuals could probably be carried across the Pacific Ocean within the time required for several reproductive cycles. It also means a high rate of gene flow is possible within a major ocean basin where there are no geographical barriers. However, gene flow might be restricted between major oceans where continents or island chains could act as physical barriers for dispersal. These predictions were supported by the analyses of mitochondrial DNA sequences of oceanic Halobates (Andersen et al. Citation2000). Haplotype lineages of H. micans from Pacific, Atlantic and Indian Oceans were significantly different from one another. Similarly, limited gene flow was suggested between H. germanus populations from Pacific and Indian Oceans. Thus, gene flow among populations of different ocean basins is limited. However, there is no correlation between nucleotide divergence and geographical distance of H. micans or H. germanus within each ocean basin.
Mutual encounter due to random movements and oceanic diffusion
How do ocean skaters find conspecifics or mates in the vast ocean once they are scattered by storms and winds? Halobates are often observed to form dense aggregations (Cheng Citation1985). Their local distribution patterns analysed from sampling data are not uniform but clumped (Cheng & Shulenberger Citation1980; Ikawa et al. Citation2002). However, little is known about the mechanism of aggregation or mating of sea skaters. They may find conspecifics by chemicals as demonstrated in a coastal veliid Rhagovelia (Trochopus) (Cheng & Roussis Citation1998) and suggested in the coastal Halobates hawaiiensis (Tsoukatou et al. Citation2001), or by detecting surface ripples as shown in a freshwater Gerris Fabricius, 1794 (Gerridae) (Wilcox Citation1979). Ignoring these aggregative factors, conservative estimates of mutual encounter rates can be obtained by two different ways, i.e. by active random movements or by passive oceanic diffusion (Ikawa et al. Citation1998).
For estimating encounter rate due to random movements, the following conservative values are assumed: speed of motion = 0.1 m s−1 (Cheng Citation1985); time for activity day−1=8 h (L. Cheng, unpublished data); distance that a skater can scan visually = 0.1 m (L. Cheng, unpublished data). Then, encounter day−1 (f r) due to random movements when the population density ρ, is
On the other hand, if ocean skaters are carried only by oceanic diffusion, the frequency of mutual encounter f o (times day−1) is obtained as
The most interesting aspect with this calculation is the dependency of mutual encounter rate on population density ρ, especially when ρ becomes very low. By oceanic diffusion, mutual encounter rate is proportional to one-third power of population density ρ. Consequently, a decrease in encounter rate with a decrease in ρ is not as steep as a linear decrease expected in the case of random movements. As is shown in , the estimated densities of Halobates in various regions of the ocean fluctuated from 8.2×10 km−2 to 2.1×105 km−2. When the density is 10 km−2, 103 km−2 or 105 km−2, we obtained encounter rates of f r=6×10−3, 0.6 and 60, and f o=5, 24 and 111, respectively. These calculations suggest that by oceanic diffusion alone an individual may be able to find a mate even after it has been carried away for a long distance into regions where densities are very low. Thus oceanic diffusion could be responsible for bringing individuals living far apart closer and result in high rates of gene flow within an ocean basin. These calculations do not contradict the results of DNA analyses but suggest that populations of Halobates in a given ocean basin are not substructured. There remain possibilities that more extensive and precise DNA and morphological analyses might reveal limited gene flow in an ocean basin. Then ocean skaters would presumably require some means of maintaining their local aggregations against oceanic diffusion.
As mentioned in previous sections, densities and distributions of ocean skaters could undergo drastic fluctuations annually and seasonally. It would be vital for them to develop means to re-aggregate or to find mates under low densities. Oceanic diffusion which acts to carry Halobates apart could also enhance encounters among dispersed populations. Riding on oceanic diffusion would be one of their tactics to survive at the ocean surface.
Adaptive significance of distribution range
One of the most notable features of distribution pattern of oceanic Halobates is their wide extension without distinct discontinuities (A). We examine the adaptive significance of such vast distribution areas and infer life-history strategies that enabled ocean skaters to survive on the high seas by using the mathematical model constructed by Skellam (Citation1951) and Kierstead & Slobodkin (1952).
All organisms have thresholds of tolerances for environmental conditions surrounding them. For an aquatic species with restricted physiological tolerances, areas of suitable water could be limited and oceanic turbulence could act to carry individuals away into unsuitable areas. Therefore, to keep a stable distribution range, there should be a balance between intrinsic growth rate and loss due to diffusion into unsuitable areas. The mathematical expression for this is
where ρ ( x, y, t) is population density as a function of space and time, r is growth rate (instantaneous rate of population increase), K is diffusivity and Ω is the region suitable for Halobates to reproduce.
The steady-state patch size for zonal and circular patch is given by
where L is the width of the zonal patch and R is the radius of the circular patch, respectively. Under the influence of oceanic diffusion, diffusivity K in equation (Equation6) should depend on the scale of phenomena, i.e. L or R. Thus, instead of K, we use a diffusivity K a from equation (Equation2). Then, expected growth rate is given by (Ikawa et al. Citation1998),
where r z is growth rate for zonal and r z for circular patch, respectively.
The distributions of Halobates micans and H. sericeus can be treated as zonal patches since their eastern and western boundaries are continental coasts and they are only able to expand towards the north and south. On the other hand, the distributions of H. sobrinus, H. splendens and H. germanus in the Pacific Ocean appear to form circular patches (Ikawa et al. Citation1998; A). In A,B are shown relationships between r z and L, and between r c and R, and habitat ranges of Halobates species as estimated in A. Because growth rate is proportional to –2/3 power of habitat size, it increases drastically with the decrease in patch size, i.e. much higher growth rates would be required to keep smaller patches stable.
Figure 8. Relationship between estimated growth rate and patch size of oceanic Halobates spp. for A: Zonal patches, B: Circular patches.
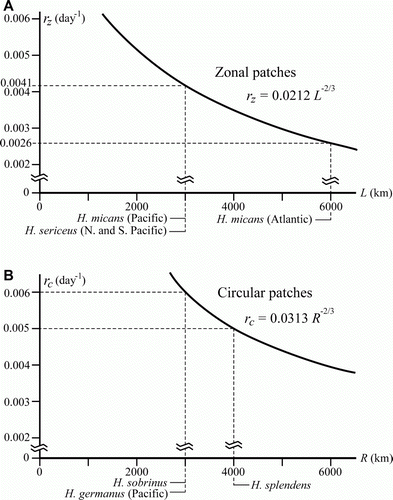
The estimated growth rates of Halobates are from 0.0026 day−1 (H. micans in the Atlantic Ocean) to 0.0060 day−1 (H. sobrinus and H. germanus). These values are rather low by comparison with 0.33 day−1 for the spotted alfalfa aphid Therioaphis maculata Buckton, 1899 (Ito Citation1975), 0.12 day−1 for the flower beetle Tribolium confusum Jacquelin Du Val, 1863 and 0.014 day−1 for the spider beetle Ptinus fur Linnaeus, 1758 (Pianka Citation1978). However, it would be appropriate to assume low growth rates for Halobates considering their relatively long life span (at least 4 months) and long oviposition period (more than 1 month) (Cheng Citation1985; L. Cheng, unpublished data). The calculations by equation (Equation7) show that low values of r can be realized only when the patch size is a few thousand kilometers in width or diameter. For Halobates, it is improbable that small-sized stable patches of less than 1.0 km in diameter could exist, because the corresponding growth rates would have to be more than 0.85 day−1, an unrealistically high value for these insects.
Turbulence is thought to be one of the major factors for excluding most insects from the open ocean (Ward Citation1992). One plausible strategy to successfully survive in rough environments is to reproduce quickly, with high growth rates, early maturity and short life span. Then, once ocean skaters find a suitable habitat, they might be able to aggregate, mate and reproduce quickly. However, the mathematical model and field observations infer a quite different life-history strategy. Ocean skaters have rather low growth rates and long life spans. This life-history strategy could have been selected due to their extremely harsh and unpredictable environment. On the ocean surface, strong winds and storms might occur too frequently to allow them to form a stable local aggregation and to complete even one generation. With high growth rate and short life span, the possibilities would be low for ocean skates to survive and reassemble in a local patch after they are scattered by storms. However, a long life span and long oviposition period would give them better chances to find conspecifics, food and suitable habitats to regroup and reproduce even after they are dispersed over long distances. Although they have been observed to form local aggregations, such flotillas would be unstable and destroyed frequently by storms and winds. Riding on oceanic turbulence, ocean skaters would repeatedly disperse, regroup and reproduce during their long life span, making their whole distribution range as one stable patch, i.e. as a freely exchanging gene pool. This prediction is supported by the results of DNA analyses (Andersen et al. Citation2000). Thus, to occupy vast regions in all three major oceans is their vital strategy for surviving in the high seas.
Concluding remarks – strategy shift from the shore to the ocean
The life of coastal sea skaters is characterized by a strong affinity to the shore where they depend on terrestrial insects falling on the sea surface for food, rocks or plants for egg-laying, and overhanging vegetation for shade, etc. Away from the shore, they cannot survive. Their life is thus inseparable from the shore and they must always move against tidal currents, winds or waves that act to carry them away. The life of pelagic skaters is, by contrast, totally different. They spend their entire lives on the ocean surface where water and air are moving unceasingly in all directions. Their life-history strategy is not to avoid oceanic turbulence but to skate with it as long as they live. During their long life span they can find mates even after they have been dispersed far away from their place of birth, treating the vast tropical ocean basin as one gene pool. To extend their distribution range fully over the broad expanse of the water would be their optimal strategy to successfully colonize the open ocean. DNA analysis suggests that the oceanic way of life evolved twice independently in Halobates; their coastal ancestors that were washed away from the shore managed somehow to adapt to life on the open ocean (Andersen et al. Citation2000). It is fascinating trying to understand how the drastic shift in life-history strategy from total dependence on the shore to the surf-riding life could have been realized.
Editorial responsibility: Tom Fenchel
Acknowledgements
This article was written during the turmoil and tragedy of the earthquake/tsunami that hit eastern Japan (where TI and HO live) on 11 March 2011. We express heartfelt thanks to Dr F. Uiblein for his understanding and to overseas as well domestic friends and colleagues for extending concerns and encouragements. We are indebted to many colleagues who have collected samples for us over the years and for providing us with sampling data. There are too many to mention individually. In particular, we wish to thank Dr A. D. Tran for providing unpublished collection data of coastal species; Mr A. Kawakubo, Mr Y. Nozoe and other members of Saikai Pearl Sea Resort for participating in field studies of Japanese sea skaters; TI's students for assistance in studies of both coastal and oceanic species; A. Townsend for help in accessing historical station data at SIO; and Drs R. L. Pitman and M. Schulz-Baldes for supplying original cruise data; S. Hoshizaki for insightful discussions; and two anonymous reviewers for their valuable comments. We wish to dedicate this article to the memory of three departed colleagues: Dr R. A. Lewin who supported us with his profound academic knowledge and as our dearest friend all through his life; Dr A. Okubo who inspired us with his great insight in mathematical biology and physical oceanography; and Dr M. Tsuchiya who led us to the fascinating world of interplay between physical and biological phenomena.
Land mask (3′ grid) in Figures and has been derived from land cover image provided by GSI, CEReS, Collaborating Organizations (http://www.iscgm.org/gmd/download/sample_image_dl.html). Global sea-surface temperature data (1°grid) in B have been adopted from World Ocean Atlas 2009, NOAA National Oceanographic Data Center (http://www.nodc.noaa.gov/OC5/WOA09/woa09data.html).
Notes
Published in collaboration with the University of Bergen and the Institute of Marine Research, Norway, and the Marine Biological Laboratory, University of Copenhagen, Denmark
References
- Andersen , NM. 1991 . Marine insects: Genital morphology, phylogeny and evolution of sea skaters, genus Halobates (Hemiptera: Gerridae) . Zoological Journal of the Linnean Society , 103 : 21 – 60 .
- Andersen , NM and Cheng , L. 2004 . The marine insect Halobates (Heteroptera: Gerridae): Biology, adaptations, distribution and phylogeny . Oceanography and Marine Biology, An Annual Review , 42 : 119 – 80 .
- Andersen , NM , Cheng , L , Damgaard , J and Sperling , FAH. 2000 . Mitochondrial DNA sequence variation and phylogeography of oceanic insects (Hemiptera: Gerridae: Halobates spp.) . Marine Biology , 136 : 421 – 30 .
- Andersen , NM and Weir , TA. 1994 . The sea skaters, genus Halobates Eschscholtz (Hemiptera: Gerridae), of Australia: Taxonomy, phylogeny and zoogeography . Invertebrate Taxonomy , 8 : 861 – 909 .
- Association of Wildlife Research, EnVision 2007 . Search System of Japanese Red Data . http://www.jpnrdb.com/index.html and http://www.jpnrdb.com/search.php?mode=map&q=07150360721 (accessed 26 March 2012). (in Japanese)
- Ban , Y , Shigeaki , S and Ishikawa , M. 1988 . Life history of the water strider Metrocoris histrio B. White (Hemiptera: Gerridae) in Aichi Prefecture, Japan . Verhandlungen ders Internationalen Vereinigung für Limnologie , 23 : 2145 – 51 .
- Berner , EK and Berner , RA. 1987 . Global Water Cycle: Geochemistry and Environment , 397 Englewood Cliffs , NJ : Prentice-Hall. .
- Birch , MC , Cheng , L and Treherne , JE. 1979 . Distribution and environmental synchronization of the marine insect, Halobates robustus, in the Galápagos Islands . Proceedings of the Royal Society of London , Series B ( 206 ) : 33 – 52 .
- Cheng , L. 1966 . Studies on the biology of the Gerridae (Hem., Heteroptera) II . Entomologists’ Monthly Magazine , 102 : 273 – 82 .
- Cheng , L. 1973a . Halobates . Oceanography and Marine Biology, An Annual Review , 11 : 223 – 35 .
- Cheng , L. 1973b . The ocean-strider Halobates (Heteroptera: Gerridae) in the Atlantic Ocean . Oceanology , 13 : 564 – 70 .
- Cheng , L. 1973c . Marine and freshwater skaters: Differences in surface fine structures . Nature , 242 ( 5393 ) : 132 – 33 .
- Cheng , L. 1974 . Notes on the ecology of the oceanic insect Halobates . Marine Fishery Review , 36 : 1 – 7 .
- Cheng L 1976a . Marine Insects . Amsterdam : North-Holland . 581 .
- Cheng , L. 1976b . “ Insects in marine environments ” . In Marine Insects , Edited by: Cheng , L . 1 – 4 . Amsterdam : North-Holland .
- Cheng , L. 1982 . Halobates from Micronesia, with notes on keeping H. mariannarum alive in the laboratory . Micronesica , 17 : 97 – 106 .
- Cheng , L. 1985 . Biology of Halobates (Heteroptera: Gerridae) . Annual Review of Entomology , 31 : 111 – 35 .
- Cheng , L. 1989 . “ Factors limiting the distribution of Halobates species ” . In Reproduction, Genetics and Distribution of Marine Organisms , Edited by: Ryland , JS and Tyler , PA . 357 – 62 . Fredensborg : Olsen and Olsen .
- Cheng , L. 1991 . The endemic sea-skater Halobates alluaudi Bergroth, 1893 (Hemiptera: Gerridae) in the Seychelles . Raffles Bulletin of Zoology , 39 : 161 – 68 .
- Cheng , L. 1997 . Disjunct distributions of Halobates (Hemiptera: Gerridae) in the Pacific Ocean . Pacific Science , 51 : 134 – 42 .
- Cheng , L. 2009 . “ Marine insects ” . In Encyclopedia of Insects , 2nd edition , Edited by: Resh , VH and Carde , RT . 600 – 04 . San Diego , CA : Academic Press .
- Cheng , L , Baars , MA and Oosterhuis , SS. 1990 . Halobates in the Banda Sea (Indonesia): Monsoonal differences in abundance and species composition . Bulletin of Marine Science , 47 : 421 – 30 .
- Cheng , L and Birch , MC. 1977 . Terrestrial insects at sea . Journal of Marine Biological Association, UK , 57 : 995 – 97 .
- Cheng , L , Damgaard , J and Garrouste , R. 2012 . The sea-skater Halobates (Heteroptera: Gerridae) – Probable cause for extinction in the Mediterranean and potential for re-colonization following climate change . Aquatic Insects , 34 ( Suppl 1 ) : 47 – 58 .
- Cheng , L , Douek , M and Goring , DAI. 1978 . UV absorption by gerrid cuticles . Limnology and Oceanography , 23 : 554 – 61 .
- Cheng , L and Frank , JH. 1993 . Marine insects and their reproduction . Oceanography and Marine Biology, An Annual Review , 31 : 479 – 506 .
- Cheng , L and Holdway , P. 1983 . Halobates collected during the circumnavigational expedition Operation Drake . Zoological Journal of Linnean Society , 78 : 187 – 98 .
- Cheng , L and Maxfield , L. 1980 . Nymphs of two sea-skaters, Halobates robustus and H. micans (Heteroptera: Gerridae) . Systematic Entomology , 5 : 43 – 47 .
- Cheng , L and Roussis , V. 1998 . Sex attractant in the marine insect Trochopus plumbeus (Heteroptera: Veliidae): A preliminary report . Marine Ecology Progress Series , 170 : 283 – 86 .
- Cheng , L and Shulenberger , E. 1980 . Distribution and abundance of Halobates species (Insecta: Heteroptera) in the eastern tropical Pacific . Fishery Bulletin , 78 : 579 – 91 .
- Cheng , L and Schulz-Baldes , M. 1981 . Frequency and population composition of Halobates micans (Heteroptera: Gerridae) from the central and South Atlantic Ocean . Meteor Forschungsergebnisse, Reihe D – Biologie , 33 : 17 – 21 .
- Cheng , L , Spear , L and Ainley , DG. 2010 . Trophic importance of marine insects (Heteroptera: Gerridae, Halobates spp.) to eastern tropical Pacific seabirds . Marine Ornithology , 38 : 91 – 95 .
- Cheng , L and Wormuth , J. 1992 . Are there separate populations of Halobates in the Gulf of Mexico? . Bulletin of Marine Science , 50 : 307 – 19 .
- Cresswell , GR and Golding , JT. 1980 . Observations of a south-flowing current in the southeastern Indian Ocean . Deep-Sea Research , 27 : 449 – 66 .
- Damgaard , J , Andersen , NM , Cheng , L and Sperling , FAH. 2000 . Phylogeny of sea skaters, Halobates Eschscholtz (Hemiptera, Geridae), based on mtDNA sequence and morphology . Zoological Journal of the Linnean Society , 130 : 511 – 26 .
- Esaki , T. 1924 . On the genus Halobates from Japanese and Formsan coasts (Hemiptara: Gerrida) . Psyche , 31 : 112 – 19 .
- Environment Agency . 1991 . The Threatened Species of Japan – Invertebrates . Tokyo : Japan Wildlife Research Center . 271 . (in Japanese)
- Foster , WA and Treherne , JE. 1986 . The ecology and behaviour of a marine insect, Halobates fijiensis (Hemiptera: Gerridae) . Zoological Journal of the Linnean Society , 86 : 391 – 412 .
- Gordon , A. 2003 . The brawniest retroflection . Nature , 421 : 904 – 05 .
- Grimaldi , D. 2009 . “ Fossil record ” . In Encyclopedia of Insects , 2nd edition , Edited by: Resh , VH and Carde , RT . 396 – 403 . San Diego , CA : Academic Press .
- Hayashi , M and Miyamoto , S. 1997 . Rediscovery of Asclepios shiranui (Heteroptera, Gerridae) from northern Kyushu, Japan . Japanese Journal of Entomology , 65 : 438 – 39 .
- Hayashi M , Miyamoto S 2003 . Distribution and habitat of Asclepios shiranui, with distributional notes on some other coastal marine skaters (Heteroptara: Gerridae and Veliidae) in northern Kyushu, Japan . Rostria 51 : 1 – 20 . (in Japanese with English summary)
- Hayashi M , Miyamoto S 2005 . Hemiptera . In : Kawai T , Tanida K Aquatic Insects of Japan: Manual with Keys and Illustrations . Kanagawa : Tokai University Press , 291 – 378 . (in Japanese)
- Hellerman , S and Rosenstein , M. 1983 . Normal monthly wind stress over the world ocean with error estimates . Journal of Physical Oceanography , 13 : 1093 – 104 .
- Herring , JL. 1961 . The genus Halobates (Hemiptera: Gerridae) . Pacific Insects , 3 : 223 – 305 .
- Hu , C , Montgomery , ET , Schmitt , RW and Muller-Karger , FE. 2004 . The dispersal of the Amazon and Orinoco River water in the tropical Atlantic and Caribbean Sea: Observation from space and S-PALACE floats . Deep Sea Research II , 51 : 1151 – 71 .
- Ikawa , T. 2009 . Preliminary study of age structure of the sea skater Halobates mariannarum Esaki (Gerridae: Hemiptera) . Journal of Morioka University , 27 : 27 – 33 .
- Ikawa , T. 2010 . Survey of Kuroshima and Takashima, two major islands of Kujukushima as habitats of the threatened marine insects, Halobates matsumurai Esaki and Asclepios shiranui Esaki (Hemiptara: Gerridae) . Journal of Morioka University , 27 : 27 – 33 .
- Ikawa T , Nozoe Y , Yamashita N , Nishimura N , Ohnoki S , Yusa K , et al. 2012 . Life histories of two sympatric endangered species of marine insects Halobates matsumurai and Asclepios shiranui (Hemiptera: Gerridae) . Psyche 261071. 7 pages .
- Ikawa , T , Okabe , H , Hoshizaki , S , Kamikado , T and Cheng , L. 2004 . Distribution of the oceanic insects Halobates (Hemiptera: Gerridae) off the south coast of Japan . Entomological Science , 7 : 350 – 57 .
- Ikawa , T , Okabe , H , Hoshizaki , S , Suzuki , Y , Fuchi , T and Cheng , L. 2002 . Species composition and distribution of ocean skaters Halobates (Hemiptera: Gerridae) in the western Pacific Ocean . Entomological Science , 5 : 1 – 6 .
- Ikawa , T , Okubo , A , Okabe , H and Cheng , L. 1998 . Oceanic diffusion and the pelagic insects Halobates spp. (Gerridae: Hemiptera) . Marine Biology , 131 : 195 – 201 .
- Ikawa T , Okabe H , Aoki M , Watanabe M 2007a . A field experiment of motion picture analysis of aggregations of the sea skater Halobates japonicus Esaki (Hemiptera: Gerridae) . Journal of Morioka University 24 : 49 – 58 . (in Japanese with English summary)
- Ikawa , T , Onodera , S , Okabe , H , Hosizaki , S and Cheng , L. 2007b . Occurrence and density of Halobates micans (Hemiptera: Gerridae) in the eastern South Indian Ocean . Entomological Science , 10 : 213 – 15 .
- Ikawa T , Susa K , Watanabe M 2006 . Preliminary study of aggregations and reproductive organs of the sea skater Halobates japonicus Esaki (Hemiptera: Gerridae) . Journal of Morioka University 23 : 103 – 08 . (in Japanese with English summary)
- Ikawa T , Watanabe M , Aoki M , Okabe H 2008 . Consecutive daily sampling of aggregations of the sea skater Halobates japonicus Esaki (Hemiptera: Gerridae) . Journal of Morioka University 25 : 31 – 37 . (in Japanese with English summary)
- Ito Y 1975 . Animal Ecology I . Tokyo : Kokinshoin . 226 . (in Japanese)
- Josey , SA , Kent , EC and Taylor , PK. 2002 . Wind stress forcing of the ocean in the SOC climatology: Comparisons with the NCEP–NCAR, ECMWF, UWM/COADS, and Hellerman and Rosenstein datasets . Journal of Physical Oceanography , 32 : 1993 – 2019 .
- Kawachino Y 2001 . New localities of Asclepios shiranui and Halobates matsumurai in Nagasaki Prefecture . Transaction of Nagasaki Biological Society 53 : 26 . (in Japanese)
- Kierstead , H and Slobodkin , LB. 1953 . The size of water masses containing plankton bloom . Journal of Marine Research , 12 : 141 – 47 .
- Kimura , S , Inoeu , T and Sugimoto , T. 2001 . Fluctuation in distribution of low-salinity water in the North Equatorial Current and its effect on the larval transport of the Japanese eel . Fisheries Oceanography , 10 : 51 – 60 .
- Kimura , S , Kasai , A , Nakata , H , Sugimoto , T , Simpson , J and Cheok , JVS. 1997 . Biological productivity of meso-scale eddies caused by frontal disturbances in the Kuroshio . ICES Journal of Marine Science , 54 : 179 – 92 .
- Leo , SST , Cheng , L and Sperling , FAH. 2012 . Genetically separate populations of the ocean-skater Halobates sericeus (Heteroptera: Gerridae) have been maintained since the late Pleistocene . Biological Journal of the Linnean Society , 105 : 797 – 805 .
- Lukas , R , Yamagata , T and McCreary , JPJ. 1996 . Pacific low-latitude western boundary currents and the Indonesian throughflow . Journal of Geophysical Research , 101 : 12209 – 16 .
- Mann , CR. 1967 . The termination of the Gulf Stream and the beginning of the North Atlantic Current . Deep-Sea Research , 14 : 337 – 59 .
- Ministry of the Environment . 2007 . The revised red list of the insects of Japan . 12 pages retrieved from http://www.env.go.jp/press/press.php?serial=8648 (accessed 26 March 2012). (in Japanese)
- Miyamoto S 1961 . Gerridae. Insecta Japonica. Series 1, Part 3 . Tokyo : Hokuryukan . 2 + 40 pages. (in Japanese)
- Miyamoto , S and Hayashi , M. 1996 . Discovery of Halobates matsumurai (Heteroptera: Gerridae) from Tsushima Is., Kyushu . Japanese Journal of Entomology , 64 : 110
- Miyamoto S , Senta T 1960 . Distribution, marine condition and other biological notes of marine waterstriders, Halobates spp., in the south-western sea area of Kyushu and western area of Japan Sea . Sieboldia 2 : 171 – 86 . (in Japanese with English summary)
- Okubo , A. 1971 . Oceanic diffusion diagrams . Deep Sea Research , 18 : 789 – 802 .
- Okubo , A. 1974 . Some speculations on oceanic diffusion diagrams . Rapports et Proces-Verbaux des Reunions, Conseil International pour l'Exploration de la Mer , 167 : 77 – 85 .
- Okubo , A. 1980 . Diffusion and Ecological Problems: Mathematical Models , 254 Berlin : Springer-Verlag .
- Open University . 1989 . Ocean Circulation . Oxford : Pergamon Press . 238 .
- Pianka , ER. 1978 . Evolutionary Ecology , 2nd edition , 397 New York , NY : Harper and Row. .
- Polhemus , DA. 1990 . Heteroptera of Aldabra Atoll and nearby islands, western Indian Ocean, Part 1. Marine Hetertoptera (Insecta): Gerridae, Veliidae, Hermatobatidae, Saldidae and Omaniidae, with notes on ecology and insular zoogeography . Atoll Research Bulletin , 345 : 1 – 16 .
- Polhemus , DA and Polhemus , JT. 1991 . Distributional data and new synonymy for species of Halobates Eschscholtz (Heteroptera: Gerridae) occurring on Aldabra and nearby Atolls, Western Indian Ocean . Journal of the New York Entomological Society , 99 : 217 – 23 .
- Polhemus , DA and Polhemus , JT. 2006 . The marine Heteroptera (Insect) of eastern New Guinea and adjacent archipelagoes . Denesia , 19 : 927 – 82 .
- Qu T , Du Y , Meyers G , Ishida A , Wang D 2005 . Connecting the tropical Pacific with Indian Ocean through South China Sea . Geophysical Research Letters 32 : L24609 . 4 .
- Ridgway KR , Condie SA 2004 . The 5500-km-long boundary flow off western and southern Australia . Journal of Geophysical Research 109 : C04017 . 18 .
- Ruxton , GD and Humphries , S. 2008 . Can ecological and evolutionary arguments solve the riddle of the missing marine insects? . Marine Ecology , 29 : 72 – 75 .
- Saunders , PM. 1971 . Anticyclonic eddies formed from shoreward meanders of the Gulf Stream . Deep-Sea Research , 18 : 1207 – 19 .
- Scheltema , RS. 1968 . Ocean insects . Oceanus , 14 : 8 – 12 .
- Skellam , JG. 1951 . Random dispersal in theoretical populations . Biometrika , 38 : 196 – 218 .
- Spalding M , Blasco F , Field C 1997 . World Mangrove Atlas . Okinawa , , Japan : The International Society for Mangrove Ecosystem . 178 .
- Spence , JR and Andersen , NM. 1994 . Biology of water striders: Interactions between systematics and ecology . Annual Review of Entomology , 39 : 101 – 28 .
- Stoner , AW and Humphris , SE. 1985 . Abundance and distribution of Halobates micans (Insecta: Gerridae) in the northwest Atlantic . Deep-Sea Research , 32 : 733 – 39 .
- Takara T , Azuma S 1972 . Fauna of Iriomote Island, Ryukyus. II. List of the insects (2) Order Hemiptera (Heteroptera) . The Science Bulletin of the Faculty of Agriculture, University of the Ryukyus 19 : 99 – 121 . (in Japanese)
- Taylor SJ 2009 . Concurrent phenologies of three semiaquatic bugs (Heteroptera: Gerridae, Veliidae) on a small river in central Illinois, USA . Psyche 2009 : 562471 . 5 .
- Tsoukatou , M , Cheng , L , Vagias , C and Roussis , V. 2001 . Chemical composition and behavioural responses of the marine insect Halobates hawaiiensis (Heteroptera: Gerridae) . Zeitschrift für Naturforschung , 56 : 597 – 602 .
- Ward , JV. 1992 . Aquatic Insect Ecology. 1. Biology and Habitat , 438 New York , NY : John Wiley and Sons .
- Wilcox , RS. 1979 . Sex discrimination in Gerris remigis: Role of a surface wave signal . Science , 20 : 1325 – 27 .
- Yone F , Matsuo T , Kawakubo A , Saijo F , Mori Y 2004 . Distribution of coastal sea skaters in southern Kujukusima . Transaction of Nagasaki Biological Society 57 : 1 – 9 . (in Japanese)