Abstract
The diet composition and prey selection of sprat (Sprattus sprattus) was studied in the Hardangerfjord, western Norway during four cruises in spring and autumn of 2009 and 2010. Feeding activity, indicated by stomach fullness, varied with sprat size, season and time of day. Small-sized sprat were found to feed both in spring and autumn, while the larger individuals had lower feeding activity late in the season. Diurnal variation in stomach fullness was observed in the autumn but not in spring. Sprat fed on a broad diversity of prey types, including copepods, phytoplankton and meroplankton. Multivariate statistical analysis suggested that the dietary composition of sprat differed significantly between years, seasons and among areas within the fjord. During spring, phytoplankton and invertebrate eggs dominated the diet, while copepods were most important in the autumn. Positive selection was found for Microsetella norvegica and euphausiid eggs, which were the most frequently occurring prey in stomachs, followed by Oithona and Acartia spp. Interannual variability in zooplankton abundance in the fjord, related to physical processes, may have a large impact on the feeding conditions for fjord populations of sprat.
Introduction
The European sprat (Sprattus sprattus Linnaeus, 1758) is a small pelagic and schooling clupeid fish, distributed in shelf waters of the Northeast Atlantic, including the Mediterranean, Black Sea, North Sea and Baltic Sea (Limborg et al. Citation2009). Sprat is an ecologically important pelagic fish species, acting as a link between plankton and production on higher trophic levels. Sprat feed on zooplankton (Möllmann et al. Citation2004; Ellis & Nash Citation1997), and have the ability to control their food sources through selective feeding (Arrhenius Citation1996; Möllmann & Köster Citation1999). Sprat is also important as prey for top predators, e.g. cod (Gadus morhua) and harbour porpoise (Phocoena phocoena) (Daan et al. Citation1990; Santos & Pierce Citation2003). The species is also of importance in commercial fisheries in the Baltic Sea, the North Sea – Skagerrak, and in fjords of southern and western Norway (Torstensen & Gjøsæter Citation1995; ICES Citation2011). The commercial fishery in Norwegian fjords has experienced large fluctuations, with low catches since the early 2000s (ICES Citation2011). Local spawning takes place in the fjords from January to July (Torstensen Citation1984), but the stock is also believed to be recruited by drift of eggs and larvae from adjacent fjords or coastal areas. Recent population studies on sprat (Glover et al. Citation2011) have demonstrated limited connectivity between Norwegian fjord and North Sea populations, indicating that Norwegian fjord-sprat can be considered as reproductively isolated populations, separated from both the North Sea and Baltic sprat populations. However, the potential link between fjord sprat and sprat in the Skagerrak (off southeast Norway) has not been studied and remains unresolved.
The availability of suitable food is usually considered to be a key factor in determining the recruitment and growth of fish. Although there are a large number of articles related to the feeding ecology of sprat in the Baltic (Möllmann et al. Citation2004; Casini et al. Citation2004; Bernreuther Citation2007) and the North Sea (De Silva Citation1973; Last Citation1987; Voss et al. Citation2009), very little is available on Norwegian coastal and fjord populations.
Fjords are semi-enclosed estuarine ecosystems where the sills act as barriers to the free interchange of deep oceanic water and deep basin water of the fjords (Falkenhaug et al. Citation1995, Citation1997). Fjords and estuaries may act as nursery areas for juvenile fish (Thiel et al. Citation1996; Landaeta et al. Citation2011a) and as a refuge for pelagic fish to avoid predation (Kaartvedt et al. Citation2009). The zooplankton composition in fjords is determined by a combination of advective exchange with the coastal waters and local production within the fjord (Salvanes et al. Citation1995). As a consequence, the abundance and composition of available prey for planktivorous fish may vary within the fjord system.
This study aims to explore trophic patterns of fjord populations of sprat. The seasonal and size-dependent diet of sprat was studied in Hardangerfjord, western Norway in order to investigate whether feeding habits of sprat varied between seasons and within the fjord area.
Material and methods
Study area
The Hardangerfjord () is 170–180 km long with a maximum depth of 850 m and is separated from the ocean by a 150-m deep sill, situated outside the fjord mouth. Further inwards, the fjord consists of a number of basins and fjord branches separated by secondary sills. The sampling was conducted onboard the RV Håkon Mosby during cruises in spring (April) and autumn (October/November) in 2009 and 2010 (). The sampling sites were located in four different areas of the fjord (): outer fjord branches, intermediate area, inner area and the innermost branches. This division follows the distribution patterns of main water masses and is similar to divisions used by previous studies performed in Hardangerfjord (Jorde & Klavestad Citation1963; Brattegaard Citation1966).
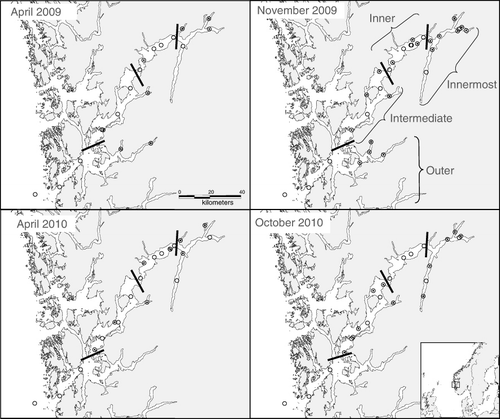
Table I Summary of sampling dates, number of stations and gear. Each ‘sprat station’ includes one trawl haul and two net tows (WP2 180 µm and Juday 90 µm).
Zooplankton and environmental data
Seawater temperature and salinity were measured at all trawl stations with a SeaBird Electronics SBE911 CTD profiler fitted with a water bottle rosette. In addition to the trawl stations, CTD casts were made at predefined stations situated along an axis extending from the fjord mouth to the fjord head (). Seawater samples for the measurements of chlorophyll a were collected from eight depths between the surface and 100 m and filtered through GF/F glass fibre filters. The filters were frozen for later chlorophyll a analysis.
Zooplankton sampling took place at each trawl station, immediately after the fish trawling, using a Juday net (90 µm, 36 cm diameter) and a WP2 net (180 µm, 56 cm diameter). The plankton nets were towed vertically from 100 m to the surface at a speed of 0.5 m s−1. Zooplankton samples were split into two halves. One half of the sample was preserved in 4% borax buffered formaldehyde–seawater solution for identification and enumeration. The other half was fractionated into size-classes for biomass estimation: 90–180 and >180 µm (Juday 90 µm net) and 180–1000, 1000–2000 and>2000 µm (WP2) and dried to constant weight at 60°C. The total size-fractionated biomass estimates were obtained by combining the 90–180 µm fraction from the Juday net with all three fractions from the WP2 net. Estimates of the numerical abundance of prey in the water column were obtained only from the 90 µm net.
Fish collection and stomach analysis
The vertical and horizontal distribution of sprat during cruises was acoustically recorded by using calibrated echosounders (Simrad EK60). Sprat were sampled with two types of pelagic trawls (): the Firkløver trawl (26×26 m opening) and the ‘Harstad trawl’ (18×18 m: Nedreaas & Smedstad Citation1987), with 22-mm mesh size (stretched) in the cod-ends. Both trawls were used with an 8 mm inner lining in the cod-ends, except in April 2010. The trawls were equipped with floats and towed within the upper 50 m at ~2 knots. Time of sampling was classified as daytime, dusk, night or dawn using sunrise and sunset times. Dusk and dawn samples were defined as those that were taken 1 h before to 1 h after sunset and sunrise, respectively. A random sample of 20–25 sprats was taken from each trawl catch for stomach analysis. The total length of each individual was measured to the nearest 0.5 cm and wet weight to the nearest 1 g. To describe size-dependent differences in diet, fishes were categorized into three size-groups:<8, 8–10 and>10 cm. Immediately after the length and weight measurements, stomachs were dissected out and frozen separately in individual plastic bags. In the laboratory, stomach contents were analysed under a stereo-microscope. The identification of prey to species level was highly dependent on the state of digestion of the contents. Prey fragments were identified to the lowest taxonomic level possible and enumerated. The dry weight of each prey category was obtained by drying at 60°C for about 24 h. Phytoplankton and unidentified stomach contents were not enumerated, but quantified as the bulk dry weight. For larger organisms, the drying period was prolonged until a constant weight was obtained. In the current study, the mean weights of the stomach contents were assessed based on all stomachs, including the empty ones.
The average amount of each prey category per fish was calculated as ‘Partial stomach Fullness Index’ (PFI) according to a slightly modified version of Lilly & Fleming (Citation1981):
If all stomach content is identified, the average ‘Total Fullness Index’ (TFI) will be equal to the sum of all PFI:
Statistical analysis and selectivity
Differences in TFI between fjord areas (), predator size (<8, 8–10 and>10 cm) and time of day (day, dusk, night, dawn) were tested for each cruise, using a one-way analysis of variance (ANOVA).
Variation in diet composition of sprat was explored using non-metric multidimensional scaling (MDS) and hierarchical cluster analysis, using PRIMER software v 6.1.6 (Clarke & Gorley Citation2006). Analyses were conducted on resemblance matrices constructed using Bray–Curtis similarities on fourth root-transformed PFI. For each trawl sample, individuals were grouped together by size-class. Groups were eliminated from the analysis when the number of fish was less than 5 in the sample, which resulted in 67 ‘dietary groups’. The mean PFI of each prey category was calculated for each group. This technique helps to overcome the variability caused by the fact that stomachs of individual fish often contain only a few of the total number of prey categories recorded (Platell & Potter Citation2001).
In order to determine whether season, fish size, sampling site and time of day influenced the dietary composition of sprat, an analysis of similarity (ANOSIM, 999 iterations, p<5%) was run to test the null hypotheses that there were no differences between groups of samples as a function of the four explanatory factors. In order to assess the appropriate similarity level for assemblage discrimination, similarity profile permutation tests (SIMPROF; 1000 iterations, p<0.05) were run. The similarity level at which the departure statistics exceeded the 5% probability criterion was used to define assemblage groups via cluster analysis.
Analysis of selectivity was based on the numerical abundance of 17 taxa identified in the stomachs. Only fishes containing food were included in the selectivity analysis. Selection of prey types was estimated using a selectivity index described by Chesson (Citation1983):
Results
Hydrography
The Hardangerfjord is seasonally affected by the freshwater run-off from rivers, causing large seasonal variations in the hydrography. The stratification is usually strongest in summer, when a brackish surface layer is present in the entire fjord system. During our investigations (spring and autumn), the freshwater influence was generally low, with average salinities >25‰ during all four cruises (Supplementary Material Table SI). During spring cruises (April 2009 and 2010) the upper 100 m was weakly stratified with a pycnocline at 20–30 m depth. Surface salinities decreased along the fjord axis from>32‰ in the outer fjord area to ~27‰ in the inner fjord branches. During autumn cruises (November 2009 and October 2010), the stratification was stronger with a pycnocline at ~10 m depth. Lower surface salinities and stronger stratification was observed in October 2010 (22.2–29.8‰) than November 2009 (23.0–30.4‰), especially in the fjord branches, where the freshwater influence was higher. Below 50 m, the salinity was more stable along the fjord (35‰). Temperatures in April increased with depths from 4.8°C at the surface to ~9°C below 50 m. In autumn, temperatures in the upper 100 m varied between 7.3 and 13.5°C, with a subsurface maximum at 20–30 m. Higher surface temperatures were observed in October 2010 compared to November 2009. For a more detailed description of the hydrography and circulation patterns of Hardangerfjord we refer to Asplin et al. (Citation2014).
Chlorophyll
A phytoplankton bloom occurred during cruises in April 2009 and 2010, and was dominated by diatoms (based on observations made on board the ship). The chlorophyll a distribution was characterized by subsurface maxima, reaching 8.4 and 6.7 mg m−3 in April 2009 and 2010, respectively (Supplement, Table SI). In April 2009, the highest chlorophyll a values were found in the inner fjord branches. In April 2010, the phytoplankton was more evenly distributed in the fjord, with areas of high concentration both in the intermediate and outer fjord area. In November 2009, chlorophyll a concentrations were low (<1 mg m−3) in the entire fjord system. In October 2010 an autumn bloom, dominated by dinoflagellates, was still prevailing, with chlorophyll a concentrations reaching 3.3 mg m−3 in the outer fjord area.
Zooplankton abundance and composition
The average zooplankton biomass in the uppermost 100 m on trawl stations ranged between 0.5 and 1.9 g m−2 and was dominated by small-sized zooplankton (<1000 µm; ). In the innermost fjord branches, the smallest fraction (90–180 µm) made up>50% of the total biomass. The proportion of large-sized zooplankton (>1000 µm) was higher in the autumn, especially in the outer fjord areas, reflecting the seasonal population growth of copepods. Higher biomass values were found in spring 2010 than in spring 2009.
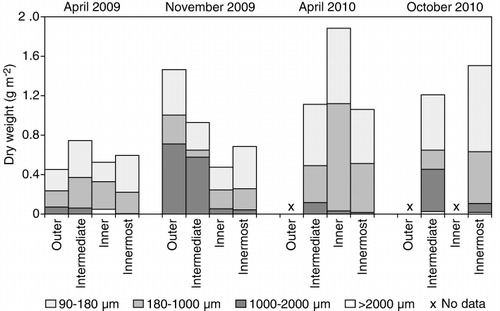
The numerically dominant zooplankton taxa during all seasons were Oithona spp., copepod nauplii and Microsetella norvegica ( and Supplementary Material Table SII). The latter species was especially abundant in the innermost fjord branches, in the autumn. The larger copepods, Calanus spp. (a mixture of C. finmarchicus and C. helgolandicus), were most abundant in the outer areas, especially in April 2009 (). Meroplanktonic larvae (mainly Bryozoa and Echinodermata) and eggs were also seasonally important components in the spring of both years.
Sprat distribution
During all four cruises, sprat schools were mainly found in fjord branches and in the inner areas of the fjord. Sprat schools were acoustically detected in the upper 25 m during night and occasionally down to 150 m depth during day. At trawl stations incorporated in this study, the sprat schools were distributed within the upper 50 m, and thus the zooplankton in the uppermost 100 m of the water column was assumed to represent the major portion of food available for sprat. The mean length of the examined sprat varied from 7.9 to 10.2 cm, with small differences among cruises. However, in April 2010, few fishes were <8 cm, possibly due to the absence of inner lining in the cod-end (). Therefore the small-sized sprat may be underrepresented in April 2010.
Sprat feeding activity
A total of 734 sprat stomachs were analysed, of which 186 were empty (). A higher proportion of empty stomachs was observed in the autumn of 2009 and 2010 (33.4% and 35.8%) than in April (10.1% and 5.6%), respectively, for the two years, indicating lower feeding activity in the autumn. Also, higher TFI was observed in spring than in autumn. The mean Total Fullness Index (TFI) was generally higher in 2009 than in 2010.
Table II Summary of number of stomachs examined (N), mean length, mean weight (wet weight) and Total Fullness Index (TFI) of sprat during cruises in 2009 and 2010.
To assess the influence of fish length on the stomach fullness, data from all sampling sites and all four cruises were combined and allocated to 0.5-cm length-classes. The overall TFI decreased significantly (p<0.05) with increasing sprat length (). The negative relationship between predator size and stomach fullness was most pronounced in the autumn (November 2009, October 2010; A). In April 2009, no significant differences in TFI between size-classes were found (p>0.05, one-way ANOVA, ).
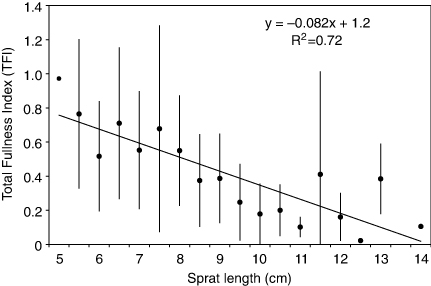
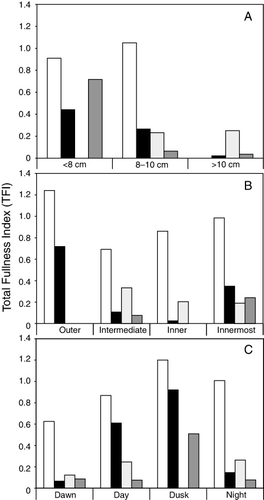
Table III Results from one-way ANOVA, testing for differences in Total Fullness Index (TFI) within fjord areas, time of day, and sprat size. Significant difference (p<0.05) is indicated in bold.
The highest TFI values were found in the outer fjord area (), which was only sampled in 2009. In autumn, feeding activity was low in the intermediate and inner fjord branches, but a slightly elevated TFI was observed in the innermost area. The geographical variation in TFI was most pronounced in the autumn, and significant differences in TFI between fjord areas were found for all size-classes in November 2009 (p<0.05, ).
The diurnal variation in TFI was most pronounced during autumn (). Highest TFI values were observed during dusk and lowest values during dawn. The differences in TFI between different periods of the day (day, dusk, night, dawn) was significant for all size-classes in November 2009 (p<0.05), but not in the spring ().
Diet composition and selection
A broad diversity of prey types was found in the sprat stomachs (). Copepods and eggs were the numerically dominating prey items. The smaller harpacticoid copepod, Microsetella norvegica, was the most frequently occurring prey, followed by euphausiid eggs, Oithona and Acartia spp. In addition, phytoplankton was also commonly observed in the stomachs during spring cruises, and made up 2–89% of the stomach content by dry weight. The larger copepods, such as Metridia spp. (including M. lucens and M. longa) and Calanus spp. (including C. finmarchicus and C. helgolandicus), were less frequent in the sprat diet. The group ‘Other’ includes a high number of taxa, each contributing less than 1% of the total number, e.g. meroplanktonic larvae, invertebrate eggs, cnidarians, phytoplankton (diatoms) and appendicularians.
Table IV Summary of prey items encountered in sprat stomachs. Total number of the prey items found in the stomachs (p), proportion of prey item p of total prey (% p), total number of fishes (N) feeding on the prey type p, proportions of fishes (% N) feeding on prey item p.
The PFI, based on prey dry weights, demonstrated clear seasonal and spatial variation in the diet of sprat (). Phytoplankton, euphausiid eggs and other eggs were the dominating components in the diet in spring, especially April 2009. In contrast, in the autumn (November 2009 and October 2010), copepods were the more important prey (). Eggs were an important constituant in the diet of sprat in the intermediate and inner fjord areas, while copepods and phytoplankton were the dominant components of the diet in the outer fjord branches (). Small differences in diet composition were found between the three size-groups of sprat (). Phytoplankton was mainly found in sprat 8–10 cm long and the highest proportion of ‘small copepods’ was observed in sprat<8 cm.
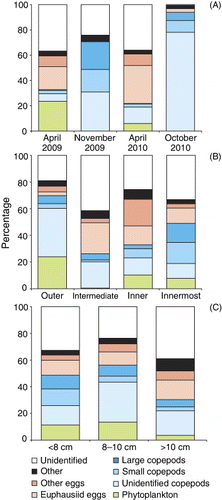
The observed seasonal and spatial variations in diet composition were further supported by the multivariate analysis (MDS). A two-dimensional spatial plot of ordination (MDS, stress=0.18, ) suggested that fishes tended to cluster according to season (month/year) and fjord area. This was confirmed by the ANOSIM test, which revealed that of the four factors investigated, sampling period (month/year) was the most important (global R=0.261, p<0.01%) followed by fjord area (R=0.151, p<0.01%). The ANOSIM test revealed no significant differences in diet between groups defined by fish size-class or time of day.
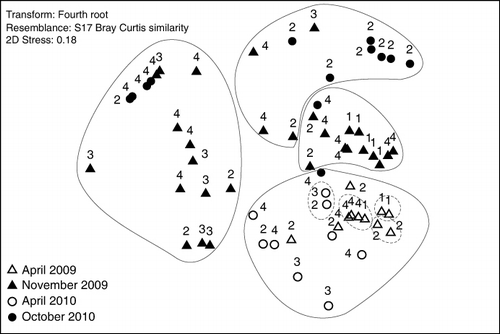
A similarity profile test (SIMPROF) of cluster analysis identified four main clusters at a similarity level of 42.4% (π=3.25, p<0.1). One of these clusters (including April 2009 and 2010) showed significant clustering into four smaller subclusters by 64.4% similarity (SIMPROF, π=1.9, p<2.9; ). The autumn clusters generally had a lower number of prey taxa, and a lower TFI, compared to the spring clusters. A larger spatial variation in the diet was found in April 2009 than in 2010, indicated by the higher number of clusters.
Sprat generally showed low selectivity for most of the prey taxa (). Strong selectivity was found for M. norvegica during all sampling periods. Euphausiid eggs were selected in spring of both years, while unidentified invertebrate eggs were only selected in April 2010. Calanus spp. and Acartia sp. were only selected in April and November 2009. Positive selection was also found for Temora sp. in April 2009. Copepod nauplii were abundant in the zooplankton samples, but were rare in the stomachs, indicating less selectivity for these.
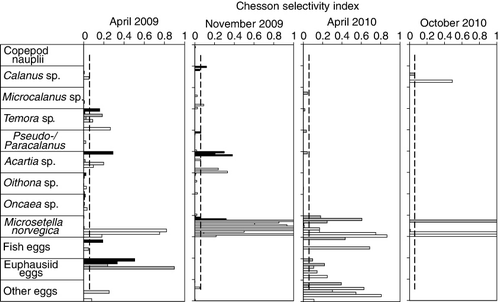
Discussion
Feeding activity
The overall stomach fullness decreased with predator size, which may be related to the higher energy demands relative to body size in smaller individuals. However, this trend was most pronounced in the autumn. All size-classes of sprat were found to feed in both spring and autumn, but a seasonal pattern in feeding activity was apparent. In spring, large-sized sprat had slightly higher TFI than smaller individuals. Reproduction has been reported to influence feeding in fish, and the metabolic demand is typically high immediately after spawning (Pedersen & Hislop Citation2001), resulting in enhanced food consumption in mature sprat during spring. In the autumn, sprat>10 cm had low feeding activity, indicated by low TFI (), and high proportions of empty stomachs in November 2009 and October 2010 (). However, small sprat (<8 cm) had relatively high TFI in October 2009, indicating that the smaller individuals continue to feed late in the season. A similar size-dependent seasonal pattern has been observed in other areas. Small individuals were found to feed through the winter in Oslofjorden (Brun Citation2007) and in the Baltic (Möllmann et al. Citation2004). In the North Sea, sprat>9 cm ceased feeding from July to February, while small individuals continued to feed through the winter (Last Citation1987; De Silva Citation1973; Szypula et al. Citation1997; Casini et al. Citation2004). These observations suggest that small sprat need more energy to survive and prioritize feeding during winter, while for large-sized sprat predator avoidance is the key issue, with reduced focus on feeding activity (Kaartvedt et al. Citation2009). Temperature is known to have a pronounced influence on the stomach evacuation of sprat (Bernreuther et al. Citation2009). Warmer temperatures and higher evacuation rates in the autumn may have resulted in lower stomach fullness. However, the seasonal difference in temperature was small (2–2.5°C), and may only partly explain the observed seasonal differences in stomach fullness.
In the current study, an interannual variation in feeding activity was evident. The mean stomach fullness (TFI) was significantly lower in April 2010 (0.25) than in April 2009 (0.56). This may partly be due to the size composition of sprat, as the mean fish size was smaller in April 2009 and TFI is expected to decrease with size. However, when comparing the TFI within one size group (8–10 cm), feeding activity was still significantly lower in April 2010 than in 2009. No differences in the number of empty stomachs were found between April 2009 and 2010, indicating that the lower TFI observed in 2010 is due to a lower feeding activity overall, and not due to a lower portion of individuals feeding. No clear differences were found in zooplankton biomass (g m−2) between the two periods (; Supplement, Table SII). On the contrary, the highest abundances of several taxa were observed in April 2010. This indicates that feeding activity may be affected by factors other than food availability alone, e.g. risk of predation or competition for food (Maes & Ollevier Citation2002; Möllman et al. Citation2004). More information on the distribution and abundances of piscivores and planktivores, e.g. herring (Clupea harengus), in the fjord is needed in order to explain the observed interannual variations in feeding activity.
Sprat is generally considered a visual predator and the main feeding period is expected during daylight hours. The highest TFI was found in fishes caught during dusk (1 h after sunset) and the lowest during dawn (1 h prior to sunrise), which is in accordance with previous studies reporting main feeding activity just before sunset (Voss et al. Citation2003). Our results suggest that sprat feed both day and night in spring, related to higher energy demands during spawning and growth. In the autumn, sprat need less energy and feeding occurs mainly during daylight hours. The more pronounced diurnal variation in TFI in the autumn may also be due to the seasonal differences in day length. More gut contents may remain throughout the shorter night in spring (8.5 h between dusk and dawn) than in autumn (15–16 h), most likely leading to a more pronounced diurnal variation in stomach fullness.
Diet composition
Copepods were found to be among the most important prey of sprat in Hardangerfjord. This is in agreement with previous studies on sprat from the North Sea (De Silva Citation1973; Last Citation1987), Oslofjorden (Brun Citation2007; Kaartvedt et al. Citation2009) and the Baltic (Coombs et al. Citation1992; Rudstam et al. Citation1992, Citation1994; Arrhenius Citation1996; Casini et al. Citation2004; Möllmann et al. Citation2004; Bernreuther Citation2007). Similar to studies from the North Sea, fjord sprat had a diverse diet including copepods, cladocerans, appendicularians, eggs and meroplanktonic larvae. To our knowledge, this is the first report on phytoplankton being found in the diet of non-larval sprat. Phytoplankton was recorded in 36% and 8% of all the analysed stomachs in April 2009 and April 2010, respectively. The amount of phytoplankton in the sprat stomachs varied considerably, and made up 2–89% of the total stomach contents by weight. The phytoplankton was usually in a newly ingested state, with single cells still visible. From this we conclude that ingestion of phytoplankton by sprat was not just incidental and that phytoplankton in the stomachs did not originate from stomach contents of digested animals. It has been shown that near-shore sardines typically consumed more phytoplankton than offshore sardines (Emmett et al. Citation2005), which may also be the case for near-shore sprat.
The presence of phytoplankton in the stomachs indicates that sprat is able to filter-feed in addition to selective particulate feeding. Sprat has previously been considered as a strictly zooplanktivorous particulate feeder that usually does not filter-feed (Bernreuther Citation2007), although microplankton (including diatoms) have been found to be important food for first-feeding sprat larvae (Dickmann et al. Citation2007). In contrast, herring is known as a facultative filter-feeder and applies this feeding mode in order to maximize the energy intake (Gibson & Ezzi Citation1992). Different feeding modes in clupeids have been observed in other estuaries as well, and may be attributed to turbidity (Maes & Ollevier Citation2002). Turbidity reduces the efficiency of visual feeding while it promotes encounter rate. Herring adapt to high turbidity levels by switching from particulate feeding to filter feeding (Gibson & Ezzi Citation1992). Our results suggest that this feeding mode is also utilized by sprat. The prey size retention efficiency of filter-feeding planktivores is largely determined by the length, shape and distance between gill rakers. Studies on the retention efficiency of sprat were not within the scope of this study. However, Karlén (Citation2011) showed that at a given length, sprat has a smaller distance between its gill rakers than herring, indicating that sprat is able to filter-feed on small-sized plankton.
Phytoplankton was more common in the fish stomachs in April 2009 (36% of all stomachs analysed), than in April 2010 (8%). The density of copepods at the trawling stations was generally lower in April 2009 than in April 2010. This would be in line with the theory of relative profitability, which states that if large organisms are in short supply, it is energetically favourable for fishes to switch to the filter-feeding mode, given that smaller prey are available in sufficient quantities (Gibson & Ezzi Citation1992). The presence of phytoplankton in the diet of sprat might indicate a low availability of larger copepods, forcing the fish to filter-feed also on smaller prey.
The numerically most important food species for sprat in the Hardangerfjord was the planktonic harpacticoid copepod, Microsetella norvegica. The high proportion of this small sized copepod (0.5–0.7 mm) in the diet contradicts results from the Baltic, where older copepodites of Pseudocalanus acuspes, Temora longicornis and Acartia spp. (1–1.5 mm) were the most important prey (Casini et al. Citation2004; Möllmann et al. Citation2004). Microsetella norvegica was present in the Hardangerfjord during all cruises, with highest abundances in the inner fjord branches in the autumn. This widely distributed species often represents one of the numerically dominant copepods in coastal waters in late summer and autumn, and has previously been reported as an important prey for fish larvae in temperate waters (Arthur Citation1976; Young & Davis Citation1992; Turner Citation2004; Morote et al. Citation2010). There is, however, little knowledge on the basic biology of the genus (Uye et al. Citation2002; Hjorth & Dahllöf Citation2008), as the traditional use of coarse-mesh samplers usually underestimates smaller copepods. It is thought to have three to four generations between March and September in boreal waters and persists during the cold period as overwintering adults throughout the water column. This suggests that M. norvegica may play an important role in the pelagic food web especially in the late season, when the larger copepod species such as Calanus spp. have migrated to deeper waters for overwintering.
In the spring of both years, eggs dominated the diet, implying again that sprat mainly feeds on small-sized prey. Among the eggs observed in this study, euphausiid eggs (0.5–0.7 mm) were by far the most abundant, but eggs of copepods, benthic invertebrates and fish were also encountered in the stomachs. It cannot be ruled out that some of the copepod eggs may have been consumed by feeding on egg-carrying females, e.g. Microsetella sp. and Oithona sp. Eggs of copepods and fishes have previously been found in the diet of sprat (Ticina et al. Citation2000; Voss et al. Citation2009; Plirú et al. Citation2012), and clupeids have been identified as major predators on cod and sprat eggs in the Baltic (Köster & Möllmann Citation2000).
Spatial and interannual variation in diet composition
The temporal and spatial variations observed in the diet composition of sprat were partly reflecting the variations in the zooplankton composition in the net tows. A clear gradient in the abundance and species composition of zooplankton in the upper 100 m was observed along the fjord axis: higher proportions of large-sized zooplankton were found in the outer areas of the fjord (e.g. Calanus spp.), while the inner fjord branches were dominated by small-sized species like Microsetella and Oithona. A similar pattern was found by previous studies in Hardangerfjord (Gundersen Citation1953; Lie Citation1967), indicating a frequent exchange between the fjord and coastal waters (Asplin et al. Citation2014). The along-fjord trend in the zooplankton composition was also reflected in the diet of sprat, but the variability among stations was pronounced. Sprat were mainly distributed and sampled within the fjord branches. These subbranches create a series of micro-basins with differences in hydrography, advection and zooplankton composition, causing large spatial variability in diet.
Selectivity
We found low selectivity estimates for most prey taxa, indicating a non-selective feeding behaviour in sprat. However, for some of the prey taxa there were noticeable differences in the abundance percentage of zooplankton species in the sea and in sprat stomachs, indicating active selection. We have not considered any effect of varying digestion times of the various prey types and therefore, the results on selectivity should be interpreted carefully.
Our results suggest that sprat actively select M. norvegica, Acartia sp., euphausiid eggs, other eggs and, to a lesser extent, Temora longicornis. Activity, size and pigmentation of prey have been shown to influence their susceptibility to predators (O'Brien Citation1979). Dark pigmentation, presence of egg sacs and poor swimming ability probably makes M. norvegica vulnerable for predation by sprat. Previous studies have shown a high selectivity for Temora, which is related to its high swimming activity (Möllmann et al. Citation2004). Oithona is a small, non-pigmented, inactive copepod, which makes this prey less conspicuous for visual feeders. However, despite the low selectivity index found for Oithona spp., this species was the third most encountered prey in the stomachs due to its high abundance in the zooplankton. Contrary to other studies (Casini et al. Citation2004), we found no selection for Pseudo/Paracalanus, and very low selection for Calanus spp. that have been found to be a preferred prey for clupeids (Dalpadado et al. Citation2000). Selectivity estimates could be biased if the zooplankton samples did not reflect the zooplankton abundances where the fish had been feeding. It should be noted that the older copepodite stages of Calanus, Metridia and Pseudocalanus may be distributed deeper in the water column, especially in the autumn. Our study covered the uppermost 100 m of the water column, and the total abundances of these copepods may have been underestimated. This would, however, lead to an overestimation of selectivity. Due to depth integrated sampling of the 0–100 m layer, we were not able to resolve the feeding interactions between predator and prey on smaller vertical scales. Further small-scale investigations with depth-stratified sampling covering the entire water column are needed to better understand the selective feeding behaviour of sprat in Hardangerfjord.
High feeding activity (high TFI) and low zooplankton abundance were observed in April 2009, while the opposite was found in April 2010. This suggests a negative relationship between feeding activity in sprat and their zooplankton prey, indicating a possible top-down relationship. Although debated, there is growing evidence that marine pelagic fish may control zooplankton populations through predation (Gjøsæter et al. Citation2011), especially in periods when predator stock size is high. Top-down control by clupeids has been documented from the Baltic (Möllmann & Köster Citation2004), the North Sea (Fauchald et al. Citation2011) and in estuaries (Maes et al. Citation2005). Further data are needed on sprat consumption rate, zooplankton production rate and advection in order to determine whether the sprat are able to control their prey in Hardangerfjord.
Despite high abundances of zooplankton in the outer fjord areas, sprat schools were usually recorded in the inner fjord branches, indicating that the distribution of sprat is affected by other factors than food availability alone. Sprat tolerates low salinities and low oxygen levels, and has been found to choose its overwintering habitat based on predator avoidance rather than prey availability (Kaarvedt et al. Citation2009). The inner parts of fjords have a turbid surface layer, causing a reduction in visibility. These areas may act as a refuge for small planktivores like sprat from predation by larger visual predators (Giske et al. Citation1994; Landaeta et al. Citation2011b). Suitable conditions for predator avoidance, combined with the presence of high abundance of suitable-sized prey (e.g. eggs, smaller size copepods), may explain the occurrence of the high abundance of sprat in the inner parts of Hardangerfjord.
In conclusion, this study is the first attempt to provide detailed information on the feeding ecology of sprat in Hardangerfjord. We have shown that fjord sprat feeds on a wide variety of prey, and that the diet is dominated by small-sized prey. This study also demonstrates a pronounced seasonal and interannual variation in diet and feeding activity in the fjord system. Interannual variability in zooplankton abundance, related to physical processes, may have a large impact on the feeding conditions for zooplanktivorous fishes in the fjord. Further studies on feeding behaviour and growth should be performed in order to better understand the dynamics and the mechanisms triggering the variability in fjord sprat populations.
Editorial responsibility: Haakon Hop
smar_a_810752_sm1887.pdf
Download PDF (110.7 KB)Acknowledgements
We thank the captains and crews of RV Håkon Mosby for their help in the collection of fish and zooplankton. We are greatly indebted to several colleagues: Else Torstensen, Cecilie Kvamme, Knut Hansen and others who assisted in the field work, Monica Martinussen for analysing zooplankton samples and sprat stomachs and Vidar Wennevik and Karen Gjertsen for drawing figures. Thanks are due to C. Kvamme and three anonymous reviewers for constructive comments on the manuscript. This study was funded by the Ministry of Fisheries and Coastal Affairs and the Norwegian Research Council (Ecological Processes and Impacts Governing the Resilience and Alternations in the Porsangerfjord and the Hardangerfjord, EPIGRAPH, project no. 188955/130).
References
- Arrhenius F. 1996. Diet composition and food selectivity of O-group herring (Clupea harengus L.) and sprat (Sprattus sprattus (L.)) in the northern Baltic Sea. ICES Journal of Marine Science 53:701–12. 10.1006/jmsc.1996.0089
- Arthur DK. 1976. Food and feeding of larvae of 3 fishes occurring in the California current, Sardinops sagax, Engraulis mordax, and Trachurus symmetricus. Fishery Bulletin 74:517–30.
- Asplin L, Johnsen IA, Sandvik AD, Albretsen J, Sundfjord V, Aure J, Boxaspen KK. 2014. Dispersion of salmon lice in the Hardangerfjord. Marine Biology Research 10:216–225.
- Bernreuther M. 2007. Investigations on the Feeding Ecology of Baltic Sea Herring (Clupea harengus L.) and Sprat (Sprattus sprattus L.). PhD Thesis. University of Hamburg, Institute of Hydrobiology and Fisheries Science. 175 pages.
- Bernreuther M, Temming A, Herrmann JP. 2009. Effect of temperature on the gastric evacuation in sprat Sprattus sprattus. Journal of Fish Biology 75:1525–41. 10.1111/j.1095-8649.2009.02353.x
- Brattegaard T. 1966. The natural history of the Hardangerfjorden. 7. Horizontal distribution of the fauna of rocky shores. Sarsia 22:1–54.
- Brun H. 2007. Vertical Distribution and Trophic Interactions of Krill, Sprat and Gadoids in the Inner Oslofjord during Winter. MSc Thesis. University of Oslo. 113 pages.
- Casini M, Cardinale M, Arrhenius F. 2004. Feeding preferences of herring (Clupea harengus) and sprat (Sprattus sprattus) in the southern Baltic Sea. ICES Journal of Marine Science 61:1267–77. 10.1016/j.icesjms.2003.12.011
- Chesson J. 1983. The estimation and analysis of preference and its relationship to foraging models. Ecology 64:1297–304. 10.2307/1937838
- Clarke KR, Gorley RN. 2006. Primer v.6: User Manual/Tutorial. Plymouth: Primer-E. 192 pages.
- Coombs SH, Nichols JH, Conway DVP, Milligan S, Halliday NC. 1992. Food availability for sprat larvae in the Irish Sea. Journal of the Marine Biological Association of the United Kingdom 72:821–34. 10.1017/S0025315400060069
- Daan N, Bromley PJ, Hislop JRG, Nielsen NA. 1990. Ecology of North Sea fish. Netherlands Journal of Sea Research 26:343–86. 10.1016/0077-7579(90)90096-Y
- Dalpadado P, Ellertsen B, Melle W, Dommasnes A. 2000. Food and feeding conditions of Norwegian spring-spawning herring (Clupea harengus) through its feeding migrations. ICES Journal of Marine Science 57:843–57. 10.1006/jmsc.2000.0573
- De Silva SS. 1973. Food and feeding habits of herring Clupea harengus and sprat C. sprattus in inshore waters of the west coast of Scotland. Marine Biology 20:282–90. 10.1007/BF00354272
- Dickmann M, Möllmann C, Voss R. 2007. Feeding ecology of Central Baltic sprat Sprattus sprattus larvae in relation to zooplankton dynamics: Implications for survival. Marine Ecology Progress Series 342:277–89. 10.3354/meps342277
- Ellis T, Nash RDM. 1997. Predation by sprat and herring on pelagic fish eggs in a plaice spawning area in the Irish Sea. Journal of Fish Biology 50:1195–202. 10.1111/j.1095-8649.1997.tb01647.x
- Emmett RL, Brodeur RD, Miller TW, Pool SS, Krutzikowsky GK, Bentley RJ, McCrae J. 2005. Pacific sardine (Sardinops sagax) abundance, distribution and ecological relationships in the Pacific northwest. California Cooperative Oceanic Fisheries Investigation Reports 46:122–43.
- Falkenhaug T, Nordby E, Svendsen H, Tande K. 1995. Impact of advective processes on displacement of zooplankton biomass in a North Norwegian fjord system: A comparison between spring and autumn. In: Skjoldal HR, Hopkins C, Erikstad KE, Leinaas HP, editors. Ecology of Fjords and Coastal Waters. Amsterdam: Elsevier Science, p 195–217.
- Falkenhaug T, Tande K, Timonin A. 1997. Spatio-temporal patterns in the copepod community in Malangen, Northern Norway. Journal of Plankton Research 19:449–68. 10.1093/plankt/19.4.449
- Fauchald P, Skov H, Skern-Mauritzen M, Johns D, Tveraa T. 2011. Wasp-waist interactions in the North Sea ecosystem. PLoS ONE 6(7):1–10. 10.1371/journal.pone.0022729
- Gibson RN, Ezzi IA. 1992. The relative profitability of particulate-feeding and filter-feeding in the herring, Clupea harengus L. Journal of Fish Biology 40:577–90. 10.1111/j.1095-8649.1992.tb02607.x
- Giske J, Aksnes DL, Fiksen Ø. 1994. Visual predators, environmental variables and zooplankton mortality risk. Vie et Milieu 44:1–9.
- Gjøsæter H, Melle W, Bogstad B, Dalpadado P, Holst JC, Huse G, et al. 2011. Er det for lite mat i havet til våre fiskebestander? Havforskningsrapporten 2011. Fisken og Havet 1/2011:100–07. (in Norwegian)
- Glover KA, Skaala O, Limborg M, Kvamme C, Torstensen E. 2011. Microsatellite DNA reveals population genetic differentiation among sprat (Sprattus sprattus) sampled throughout the Northeast Atlantic, including Norwegian fjords. ICES Journal of Marine Science 68:2145–51. 10.1093/icesjms/fsr153
- Gundersen KR. 1953. Zooplankton investigations in some fjords in Western Norway during 1950–1951. Fiskeridirektoratets Skrifter, Serie Havundersøkelser 10:1–54.
- Hjorth M, Dahllof I. 2008. A harpacticoid copepod Microsetella spp. from sub-Arctic coastal waters and its sensitivity towards the polyaromatic hydrocarbon pyrene. Polar Biology 31:1437–43. 10.1007/s00300-008-0483-3
- ICES. 2011. Report of the Herring Assessment Working Group for the Area South of 62 N (HAWG), 16–24 March 2011, ICES Headquarters, Copenhagen. ICES CM 2011/ACOM:06. 749 pages.
- Jorde I, Klavestad N. 1963. The natural history of the Hardangerfjorden. 4. The benthonic algal vegetation. Sarsia 9:1–99.
- Kaartvedt S, Rostad A, Klevjer TA. 2009. Sprat Sprattus sprattus can exploit low oxygen waters for overwintering. Marine Ecology Progress Series 390:237–49. 10.3354/meps08196
- Karlén M. 2011. Are Diets in Herring (Clupea harengus) and Sprat (Sprattus sprattus) Reflected in their Morphology – A Study on Gill Rakers. Degree project Thesis. Department of Systems Ecology, Stockholm University, Stockholm. 12 pages.
- Köster FW, Möllmann C. 2000. Egg cannibalism in Baltic sprat Sprattus sprattus. Marine Ecology Progress Series 196:269–77. 10.3354/meps196269
- Landaeta MF, Contreras J, Bustos CA. 2011a. Chilean fjords: Potential nursery ground of Bathylagichthys parini (Pisces: Osmeriformes: Bathylagidae). Revista de Biologia Marina y Oceanografia 46:67–71. 10.4067/S0718-19572011000100010
- Landaeta MF, López BG, Suárez-Donoso N, Bustos CA, Balbontín F. 2011b. Larval fish distribution, growth and feeding in Patagonian fjords: Potential effects of freshwater discharge. Environmental Biology of Fishes 93:73–87. 10.1007/s10641-011-9891-2
- Last JM. 1987. The food of immature sprat (Sprattus sprattus (L.)) and herring (Clupea harengus L.) in coastal waters of the North Sea. Journal du Conseil 44:73–79. 10.1093/icesjms/44.1.73
- Lie U. 1967. The natural history of the Hardangerfjorden. 8. Quantity and composition of the zooplankton, September 1955–September 1956. Sarsia 30:49–74.
- Lilly GR, Fleming AM. 1981. Size relationships in predation by Atlantic cod, Gadus morhua, on capelin, Mallotus villosus, and sand lance, Ammodytes dubius, in the Newfoundland area. NAFO Scientific Council Studies 1:41–45.
- Limborg MT, Pedersen JS, Hemmer-Hansen J, Tomkiewicz J, Bekkevold D. 2009. Genetic population structure of European sprat Sprattus sprattus: Differentiation across a steep environmental gradient in a small pelagic fish. Marine Ecology Progress Series 379:213–24. 10.3354/meps07889
- Maes J, Ollevier F. 2002. Size structure and feeding dynamics in estuarine clupeoid fish schools: Field evidence for the school trap hypothesis. Aquatic Living Resources 15:211–16. 10.1016/S0990-7440(02)01181-6
- Maes J, Tackx M, Soetaert K. 2005. The predation impact of juvenile herring Clupea harengus and sprat Sprattus sprattus on estuarine zooplankton. Hydrobiologia 540:225–35. 10.1007/s10750-004-7146-3
- Morote E, Olivar MP, Villate F, Uriarte I. 2010. A comparison of anchovy (Engraulis encrasicolus) and sardine (Sardina pilchardus) larvae feeding in the Northwest Mediterranean: Influence of prey availability and ontogeny. ICES Journal of Marine Science 67:897–908. 10.1093/icesjms/fsp302
- Möllmann C, Köster FW. 1999. Food consumption by clupeids in the Central Baltic: evidence for top-down control? ICES Journal of Marine Science 56:100–13. 10.1006/jmsc.1999.0630
- Möllmann C, Kornilovs G, Fetter M, Koster FW. 2004. Feeding ecology of central Baltic Sea herring and sprat. Journal of Fish Biology 65:1563–81. 10.1111/j.0022-1112.2004.00566.x
- Nedreaas K, Smedstad OM. 1987. Abundance and distribution of postlarvae in the 0-group saithe survey in the North saithe survey in the North Sea and the Northeast Arctic in 1986 and 1987. ICES CM 1987/G:31. 27 pages.
- O'Brien WJ. 1979. The predator–prey interaction of planktivorous fish and zooplankton. American Scientist 67:572–81.
- Pedersen J, Hislop JRG. 2001. Seasonal variations in the energy density of fishes in the North Sea. Journal of Fish Biology 59:380–89. 10.1111/j.1095-8649.2001.tb00137.x
- Plirú A, van der Kooij J, Engelhard GH, Fox CJ, Milligan SP, Hunter E. 2012. Sprat feeding behaviour, selective predation, and impact on plaice egg mortality. ICES Journal of Marine Science 69:1019–29. 10.1093/icesjms/fss081
- Platell ME, Potter IC. 2001. Partitioning of food resources amongst 18 abundant benthic carnivorous fish species in marine waters on the lower west coast of Australia. Journal of Experimental Marine Biology and Ecology 261:31–54. 10.1016/S0022-0981(01)00257-X
- Rudstam LG, Hansson S, Johansson S, Larsson U. 1992. Dynamics of planktivory in a coastal area of the northern Baltic Sea. Marine Ecology Progress Series 80:159–73. 10.3354/meps080159
- Rudstam LG, Aneer G, Hilden M. 1994. Top-down control in the pelagic Baltic ecosystem. Dana 10:105–29.
- Salvanes AGV, Aksnes D, Fossa JH, Giske J. 1995. Simulated carrying capacities of fish in Norwegian fjords. Fisheries Oceanography 4:17–32. 10.1111/j.1365-2419.1995.tb00058.x
- Santos MB, Pierce GJ. 2003. The diet of harbour porpoise (Phocoena phocoena) in the northeast Atlantic. Oceanography and Marine Biology 41:355–90.
- Szypula J, Grygiel W, Wyszynski M. 1997. Feeding of Baltic herring and sprat in the period 1986–1996 in relation to their state of biomass. Bulletin of the Sea Fisheries Institute 3:73–83.
- Thiel R, Mehner T, Kopcke B, Kafemann R. 1996. Diet niche relationships among early life stages of fish in German estuaries. Marine and Freshwater Research 47:123–36. 10.1071/MF9960123
- Ticina, V, Vidjak O, Kacic I. 2000. Feeding of adult sprat Sprattus sprattus, during spawning season in the Adriatic Sea. Italian Journal of Zoology 67:307–11. 10.1080/11250000009356329
- Torstensen E. 1984. Sprat spawning in two fjord areas of western Norway in 1982 and 1983. ICES Doc. CM 1984/H:41:1–16.
- Torstensen E, Gjøsæter J. 1995. Occurrence of 0-group sprat (Sprattus sprattus) in the littoral zone along the Norwegian Skagerrak coast 1945–1992, compared with the occurrence of 0-group herring (Clupea harengus). Fisheries Research 21:409–21. 10.1016/0165-7836(94)00297-A
- Turner JT. 2004. The importance of small planktonic copepods and their roles in pelagic marine food webs. Zoological Studies 43:255–66.
- Uye S, Aoto I, Onbe T. 2002. Seasonal population dynamics and production of Microsetella norvegica, a widely distributed but little-studied marine planktonic harpacticoid copepod. Journal of Plankton Research 24:143–53. 10.1093/plankt/24.2.143
- Voss R, Köster FW, Dickmann M. 2003. Comparing the feeding habits of co-occurring sprat (Sprattus sprattus) and cod (Gadus morhua) larvae in the Bornholm Basin, Baltic Sea. Fisheries Research 63:97–111. 10.1016/S0165-7836(02)00282-5
- Voss R, Dickmann M, Schmidt JO. 2009. Feeding ecology of sprat (Sprattus sprattus L.) and sardine (Sardina pilchardus W.) larvae in the German Bight, North Sea. Oceanologia 51:117–38. 10.5697/oc.51-1.117
- Young JW, Davis TLO. 1992. Feeding ecology and interannual variations in diet of larval jack mackerel, Trachurus declivis (Pisces, Carangidae), from coastal waters of eastern Tasmania. Marine Biology 113:11–20. 10.1007/BF00367633