Abstract
The boreal bivalve Modiolus modiolus is common subtidally where it aggregates to form extensive, long-lived, biogenic habitats with a diverse associated flora and, especially, fauna. Despite this ecological importance, M. modiolus has not been described in terms of its functional morphology and overall biology. Modiolus modiolus is a typical epibenthic, suspension-feeding mytilid, albeit with anatomical modifications adapting it to a partially buried, gregarious lifestyle in a stable environment experiencing medium–high energy levels. The juvenile shell is covered partly in byssal setae secreted by the byssal gland and foot complex and becomes covered in sand grains held in place by a mucoid cement secreted by the dorsal mantle. The camouflaged shell at this vulnerable time probably serves as an anti-predator device. Individuals grow to maximum shell lengths of ∼60–213 mm, depending on depth and locality. With age (≥ 20–45 years), shells often become deformed, particularly posteriorly and around the byssal gape, thereby increasing reproductive capacity (gonadal volume) without increasing somatic growth. Information on the biology, reproductive strategy and life history traits of M. modiolus are reviewed. These field- and laboratory-derived data provide us with essential information to aid future research into the protection and conservation of this ecologically important biogenic habitat. This is because, today, dredging and fishery activities using bottom-towed gear have seriously damaged several M. modiolus habitats with deleterious impacts on ecosystem functioning. Post-impact recovery times are slow and dependent on both local and mega-population distributions.
Introduction
The large, conspicuous shell of Modiolus modiolus (Linnaeus, 1758) has been reported on widely from northern boreal waters, focusing on: (1) growth and reproduction; (2) potential fishery resource; and (3) the ecological importance of its biogenic aggregations that function as habitats for a highly diverse associated fauna. Surprisingly, its functional morphology has not been fully described, nor has the biology of this widespread species been reviewed.
The Mytilidae is one of the most diverse bivalve families in terms of habitats, diet and reproductive strategies. Surprisingly few species have been studied, however, in terms of their biology and functional morphology. Ecologically, the most important mytilids constitute the taxa that aggregate epibenthically and, thereby, create biogenic habitats that are often inhabited by a rich diversity of other biota. The majority of such marine, habitat-forming mytilids are assigned to one of three major subfamilies. They are the Mytilinae Rafinesque, 1815, Modiolinae G. Termier & H. Termier, Citation1950 and Bathymodiolinae Kenk & B. R. Wilson, 1985 (Carter et al. Citation2011) with the intertidal Mytilus edulis Linnaeus, 1758, the subtidal M. modiolus, and the deep-sea hydrothermal vent Bathymodiolus thermophilus Kenk & B. R. Wilson, 1985, as the type species, respectively. All three species reproduce by means of planktotrophic larvae (Bayne Citation1964; de Schweinitz & Lutz Citation1976; Lutz et al. Citation1980), enabling recruitment to and occupancy of patchily distributed habitats.
Mytilus edulis is probably among the most studied bivalves, not least because of its economic and ecological importance (Seed Citation1976, Citation1996; Suchanek Citation1985; Seed & Suchanek Citation1992; Seed et al. Citation2000), but there is also a thorough account of the anatomy of the adult by White (Citation1937). The morphology of B. thermophilus has been described by Kenk & Wilson (Citation1985) and the ecological importance of aggregations of this bivalve for its associated fauna has been documented by Van Dover & Trask (Citation2000) and Van Dover (Citation2002). Only limited anatomical information is available on M. modiolus and other modiolines (Soot-Ryen Citation1955) and mytilid systematics is still based largely on adult shell morphology (Soot-Ryen Citation1969; Carter et al. Citation2011). The functional morphology of M. modiolus has never been described, despite the fact that such a study would seem to be a prerequisite to defining the genus and subfamily sensu stricto and sensu lato, respectively, and to understanding the biology of this important, marine habitat-forming, biodiversity resource.
The potential importance of M. modiolus as a marine fishery resource accounts for some studies of shell growth (Lilleskare Citation1905; Williamson Citation1906; Wiborg Citation1946; Strand & Vølstad Citation1997), while the majority of others address habitat adaptations (Lande et al. Citation1973; Brown et al. Citation1976; Strömgren Citation1976; Brown & Seed Citation1977; Seed & Brown Citation1978; Anwar et al. Citation1990; Brown Citation1990) and/or reproduction (Nordgaard, Citation1901; Williamson Citation1906; Seed & Brown Citation1975, Citation1977; Comely Citation1978, 1981; Brown Citation1984; Jasim & Brand Citation1989) and predation (Nielsen Citation1975; Roberts Citation1975; Elner & Campbell Citation1987). The ecological importance of the far-flung populations of putative M. modiolus as a habitat for a diverse associated biota has been reported upon from both North Atlantic and Northeast Pacific coastal shelf areas (e.g. Petersen Citation1918; Spärck Citation1929; Shelford Citation1935; Einarsson Citation1941; Wiborg Citation1946; Thorson Citation1950; Roberts Citation1975; Noble et al. Citation1976; Comely Citation1978; Logan et al. Citation1984; Sebens Citation1985; Witman Citation1985, Citation1987; Ojeda & Dearborn Citation1989; Brown Citation1990; Göransson & Karlsson Citation1998; Mair et al. Citation2000; Rees et al. Citation2008; Sanderson et al. Citation2008; Ragnarsson & Burgos Citation2012). The inclusion of M. modiolus in the European Conservation network NATURA 2000 as a biogenic reef habitat (Connor et al. Citation2004) and the OSPAR List of threatened and/or declining species and habitats (OSPAR Citation2008; Rees Citation2009) have increased the reporting upon it from European waters. Conversely, because of bottom trawling for other fishery resources, several M. modiolus habitats have been impacted adversely (Magorrian & Service Citation1998; Veale et al. Citation2000; Kenchington et al. Citation2005; Lambert et al. Citation2011; Strain et al. Citation2012), possibly in coincidence with increasing sea temperatures (Kirby et al. Citation2008).
Because they differ with respect to habitat, sources of nutrition and life history traits, it is anticipated that the functional morphologies of representatives of the three mytilid subfamilies reflect such distinctions, as has been demonstrated for some mytilids (Jeffreys Citation1863; Bernard Citation1898; List Citation1902; White Citation1937; Soot-Ryen Citation1955; Stanley Citation1970, Citation1972; Morton Citation1977a, Citation1977b; Lutz & Hidu Citation1979; Ockelmann Citation1983, Citation1995; Fuller & Lutz Citation1989; Carter Citation1990a, Citation1990b; Kafanov & Drozdov Citation1998; Coan et al. Citation2000; Healy et al. Citation2000; Ockelmann & Dinesen Citation2009, Citation2011; Genio et al. Citation2012). Mytilid systematics (Thiele Citation1934; Soot-Ryen Citation1969; Carter Citation1990a; Morton Citation1992; Huber Citation2010; Carter et al. Citation2011) and taxonomic classification (e.g. Abbott Citation1974; Tebble Citation1976; Bernard et al. Citation1993; Higo et al. Citation1999; Coan et al. Citation2000) are largely based on adult shell morphologies. Increasing numbers of molecular studies have, however, identified phylogenetic clades and, recently, focused on deep-sea mytilids using M. modiolus as a closely related taxon (Distel Citation2000; Chichvarkin Citation2002; Samadi et al. Citation2007; Lorion et al. Citation2009, 2010; Ritt et al. Citation2012). Comprehensive information on anatomy and genetics are required, some would argue urgently, as a basis for a first phylogenetic revision of the diverse Mytilidae as understood by Soot-Ryen (Citation1969), Bernard et al. (Citation1993) and Carter et al. (Citation2011), including representatives of the three subfamilies (Modiolinae, Mytilinae and Bathymodiolinae) herein under examination, with more paraphyletic taxa (Carter et al. Citation2011).
The aims of this paper were to: (1) describe the functional morphology and anatomy of M. modiolus; (2) review existing information on biological and life history traits; (3) compare and discuss field-derived information in relation to experimentally derived data; (4) relate these studies to the ecological importance of the species within (5) the broader framework of the success of the habitat-forming Mytilidae.
Taxonomy
Family Mytilidae Rafinesque, 1815
Subfamily Modiolinae Termier & Termier, Citation1950
Modiolinae Termier & Termier, Citation1950: 81, 85. Type genus Modiolus Lamarck, 1799.
Genus Modiolus Lamarck, 1799
Type species: Mytilus modiolus Linnaeus, 1758. Recent.
Modiolus modiolus (Linnaeus, 1758)
Description
Life history comprising four shell stages, including two larval prodissoconchs (I and II), an early post-metamorphic nepioconch and the dissoconch of the juvenile and adult individuals. Dissoconch equivalve, inequilateral, margins smooth, posterior margin enlarged, rounded, anterior margin less rounded, projecting slightly beyond the umbones, antero and postero-dorsal margin steep, ventral margin almost straight except for a byssal notch, which becomes more pronounced in older individuals. Maximum shell lengths of 65–210 mm, depending on locality. Dissoconch comprises three shell layers: an outer finely prismatic calcite layer and middle and inner aragonitic nacreous layers, the latter with prismatic bands. Internal shell whitish with blue radiating rays. Shell deformations sometimes seen in older individuals. Periostracum thin and light brown in young individuals, thicker and darkish brown and partly abraded in larger individuals. Juveniles with a light grey glue pasted over the postero-dorsal regions. Byssal setae present on the posterior end in both juveniles and adults. Dissoconch lacks teeth anterior to the ligament. Secondary ligament opisthodetic, parivincular, located between resilifers, formed separately from the primary ligament and comprising a darker brown outer and posterior inner ligament layers.
Anterior adductor muscles narrow, dorso-ventrally elongated, posterior adductor large, circular. The byssal and posterior pedal retractor muscles penetrate the visceral mass. Foot posterior with large, stalked byssus. Exhalant siphon situated posterior to the posterior adductor muscle and comprising a low unadorned, cone. Inhalant aperture long, not separated from the pedal gape. Siphon and aperture pigmented dark brown. Ctenidia made up of two demibranchs of equal dorso-ventral height, the outer slightly longer than the inner, one cephalic eyespot located underneath the ctenidial axis of both ctenidia at the extreme anterior end of the outer demibranchs. Stomach of Type III (Purchon Citation1957), style sac and midgut conjoined, hindgut simple, rectum traverses the ventricle of the heart to terminate in an anus on the posterior margin of the posterior adductor muscle. Gonads in visceral mass, but not in mantle; dioecious.
Distribution
Lower intertidal to ∼200 m (Comely Citation1978), although individuals may be found at greater depths (Dinesen & Ockelmann Citation2005). Lives byssally attached, either epibenthic or semi-infaunal on and in a variety of substrata ranging from fine mud with shells and gravel to bedrock. Geographical distribution hitherto considered circum-boreal (Jeffreys Citation1863; Jensen Citation1912; Wiborg Citation1946; Dodge Citation1952; Soot-Ryen Citation1955; Roberts Citation1975, Tebble Citation1976; Coan et al. Citation2000). However, a recent molecular study has identified different haplotype networks between Northeast Pacific and Atlantic individuals of putative Modiolus modiolus, suggesting that Pacific individuals may represent a hitherto unrecognized taxon (Halanych et al. Citation2013).
Etymology
Modiolus. Latin, diminutive of modius: measuring vessel, a measure of grain of ∼8.75 litre (Hansson Citation1998).
Remarks
Modiolidae G. Termier & H. Termier, Citation1950 was recently declared a nomen protectum under the ICZN Article 23 and Jolyidae Bourguignat, 1877 was declared a nomen oblitum (Bouchet et al. Citation2010). Under ICZN Opinion 325, Modiolus Lamarck, 1799 was declared a nomen conservandum pro Volsella Scopoli, 1777. Junior synonyms of Modiolus include Modiola Lamarck, 1801 and Eumodiolus Ihering, 1900. Gray (Citation1847) designated Mytilus modiolus Linnaeus, 1758 as the type species of Modiolus Lamarck, 1799 (Jukes-Brown Citation1905; Dodge Citation1952; Soot-Ryen Citation1955, Citation1969; Vokes Citation1980; Coan et al. Citation2000). The Mediterranean is the type locality, but the species has not been reported from there subsequently and the type material is missing (Soot-Ryen Citation1955). For further descriptive details and references to the related literature, see the section on functional morphology below.
Halanych et al. (Citation2013) showed that individuals of Modiolus modiolus from the Northeast Pacific represented a different haplotype network with no haplotypes in common with Atlantic individuals – possibly indicating that putative M. modiolus in the former locatity constitutes a different taxon. These authors further argue that if this is so, a Pacific origin for the two sister species would be most likely, with subsequent dispersal to and speciation in the North Atlantic. Reid (Citation1990) argues that the fossil record indicates species of Littorina (Gastropoda) reached the North Atlantic by an Arctic route following the opening of the Bering Strait in the Upper Pliocene (∼2.6–3.6 Mya) and then speciated. Vermeij (Citation1991) argues the same for other molluscan species. It is not inconceivable, therefore, that the Pacific ancestor of M. modiolus did the same via this trans-Arctic interchange (Vermeij Citation1991). This hypothesis, however, requires further examination.
Functional morphology
Specimens examined and methods applied
Living individuals of Modiolus modiolus were collected from: (1) the East Faroese Shelf, Northeast Atlantic Ocean at depths of between 65 and 90 m and fixed in 4% borax-saturated formaldehyde in seawater and transferred to 80% ethanol (6 specimens, ZMUC-BIV-463); (2) Knæhagen Reef, Øresund, Denmark, at depths of between 28 and 30 m and preserved in 80% ethanol (4 specimens, ZMUC-BIV-464) and (3) the Limfjord, Denmark, at depths of between 10 and 15 m and preserved in 70% ethanol (1 specimen, ZMUC-BIV-465). Beached shell material was collected from Ålbæk Bay, Kattegat, Denmark (10 shells, 3 valves, ZMUC-BIV-466). The above individuals formed the basis for anatomical studies of M. modiolus and have been lodged in the collections of the Natural History Museum, Copenhagen (see accession numbers in brackets above). Two individuals identified as M. modiolus from Haiyangdao Island, Yellow Sea, China, provided as a gift by the late Professor Liu Ruiyu (First Institute of Oceanology, Qingdao, China) have been examined for a comparison with adult individuals of M. modiolus sensu stricto from the Northeast Atlantic and adjacent seas. The Chinese specimens discussed herein have been deposited in the collections of the Natural History Museum, London, with the accession number NHM: 20120253. Two juvenile individuals of M. modiolus of ∼13 mm shell length collected from dredged gravel, at between 11 and 35 m depth, from near Tjärnö, Sweden (58°52.424′N, 11°06.178′E, September 2010) by J.D. Taylor and E.A. Glover. One of these was serially sectioned transversely at 6 µm and, following routine histological procedures, stained in Ehrlich's haematoxylin and eosin. Studies of this individual have complemented the anatomical observations upon adults and the other juvenile but, more specifically, were invaluable in interpreting microstructures and the facilitation of shell camouflage.
Two shells of Bathymodiolus thermophilus from the collection of the Zoological Museum of the University of Copenhagen, Denmark (ZMUC-BIV-467) and shells of Mytilus galloprovincialis Lamarck, 1819 collected from the Mewsbrook Groyne, Rustington, West Sussex, UK (site details in Morton Citation2011) were also studied.
All figures were hand-drawn, digitized and labelled based on the following material: , juvenile M. modiolus; , , , , , , adult M. modiolus (ZMUC-BIV-463, 464 and 464); , adult M. modiolus ZMUC-BIV-465; , , juvenile M. modiolus (transverse sections); : A, adult M. modiolus (ZMUC-BIV-461, 462 and 463), B, adult M. galloprovincialis; and C, adult B. thermophilus (ZMUC-BIV-467).
Abbreviations used in the figures
Numbers in superscript used in the abbreviations refer to the terminology introduced by Purchon (Citation1957) on bivalve stomach classification; a, anus; aa, anterior adductor muscle (or scar); abr, anterior byssal retractor muscle (or scar); ana, anterior aorta; ast, anterior shell thickening; au, auricle; bg, basiphilic gland; bgr, byssal groove; bs, byssal smear; by, byssus; bgr, byssus gland; bys, byssal setae; bys(lc), byssal setae (lenticular centre); bys(oc), byssal setae (outer coat); c, sorting caecum; ca, ctenidial axis; ce, cephalic eyespot; cp-vg-conn, cerebro-pleural–visceral ganglia connective; crt, ciliary rejection tract; cs, crystalline style; csmg, conjoined style sac and midgut; dd, digestive diverticula; ddd1, duct to the digestive diverticula1; ddd2, duct to the digestive diverticula2; ddd3, duct to the digestive diverticula3; ddd4, duct to the digestive diverticula4; dh, dorsal hood; eg, eosinophilic gland; es, exhalant siphon; f, foot; fis, fused inhalant siphon; g, gonad; gs, gastric shield; h, heart; hg, hindgut; ia, inhalant aperture; id, inner demibranch; ill, inner ligament layer; ilp, inner labial palp; imf, inner mantle fold; ipl, inner periostracal layer; iss, inter-siphonal septum; k, kidney; l, ligament; lp, labial palp; m, mouth; ma, mantle; mat, major typhlosole; mg, midgut; mit, minor typhlosole; mm, mantle margin; mmf, middle mantle fold; mpl, middle periostracal layer; m-pc, mantle–labial palp connective; o, oesophagus; od, outer demibranch; og, oral groove; olm, outer lip of mouth; olp, outer labial palp; omf, outer mantle fold; opl, outer periostracal layer; p, periostracum; pa, posterior adductor muscle (or scar); palid, point of attachment of the ascending lamella of the inner demibranch to the visceral mass; palod, point of attachment of the ascending lamella of the outer demibranch to the mantle; pam, point of attachment of the mantle dorsally to the shell; pan, posterior adductor muscle nerve; pbr(1), component 1 of the posterior byssal retractor muscle (or scar); pbr(2), component 2 of the posterior byssal retractor muscle (or scar); pbr(3), component 3 of the posterior byssal retractor muscle (or scar); pe, pericardium; peg, pericardial gland; pg, periostracal groove; pga, pedal gape; pl, pallial line; pn, pallial nerve; pol, posterior outer ligament layer; ppr, posterior pedal retractor muscle (or scar); prm, pallial retractor muscle (or scar); ps, pallial secretion; r, rectum; rc, right ctenidium; re, resilifer; r-ga, renal-gonadal aperture; sa, sorting area; sa2, sorting area2; sa3, sorting area3; sf, swollen filament tip; sg, sand grains; sn, siphonal nerve; spc, supra-pericardial cavity; st, stomach; u, umbo; v, ventricle; vg, visceral ganglion; vg-conn, visceral ganglia connective; vm, visceral mass; vm-pc, visceral mass–labial palp connective.
The juvenile shell
A juvenile individual (∼13 mm shell length) of Modiolus modiolus is illustrated in . The most noticeable feature of such a small dissoconch shell is the presence of byssal setae (bys) located predominantly posteriorly on the outer surface of the periostracum that covers the shell. Another feature is that there is a light grey glue pasted over, especially, the postero-dorsal regions of the shell and to which sand grains (sg) attach. This has been termed a byssal smear (bs), although, as will be described, the origin of such a glue might reside within either the dorsal mantle or the foot. The byssal setae are illustrated in greater detail in from the anterior (B) and posterior (C) regions of the juvenile shell. The former are longer and thinner, whereas the latter are shorter, stouter and more curved.
The adult shell
The mineralogy and microstructure of the adult shell of Modiolus modiolus have been described as calcite and aragonite and to comprise three layers, that is, an outer layer of finely prismatic calcite, a middle layer of nacreous aragonite and an inner layer, also of nacreous aragonite but with prismatic bands (Taylor et al. Citation1969, Carter Citation1990b), which does not challenge the notion of M. modiolus being closely related to the Bathymodiolinae (Genio et al. Citation2012), as some molecular results suggest (Samadi et al. Citation2007).
An adult, generally equivalve, dissoconch shell of M. modiolus is illustrated from the right side in and is distinctively inequilateral. That is, the posterior margin is enlarged and rounded whereas the anterior shell margin is less rounded and projects only slightly anteriorly beyond the umbones. The periostracum is light brown in young individuals but much thicker and darker (and often abraded) in older ones. The antero- and postero-dorsal shell margins are steep (the former slightly curved) and meet at a mid-dorsal apex. The ventral margin is almost straight except for a slight byssal notch that in some individuals, as will be seen, becomes much more pronounced with age. The shell is characterized by an external pattern of commarginal growth lines. Seen from the dorsal aspect (), the external, opisthodetic ligament (l) extends posteriorly from the umbones (u) for not quite half the length of the shell. Ventrally (), there is no significant byssal gape. From the anterior aspect (), the shell is pointed dorsally but laterally swollen ventrally, with the ligament (l) arising from the umbones (u) located approximately half way along the dorso-ventral axis of the shell. The shell is also widest (, x–y) at this level. From the posterior aspect (), the margins of the valves are united everywhere with no marginal crenulations.
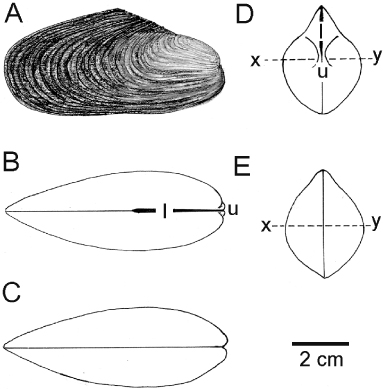
Four deformed shell valves of M. modiolus are illustrated in . A and B are left and C and D are right shell valves of individuals that show varying degrees of ventral and posterior deformation. Most notably, the ventral regions of them all have deformed and exaggerated byssal gapes and the two left valves show postero-dorsal deformations. illustrates an adult M. modiolus shell showing how growth has been stunted posteriorly. This has resulted in the growth lines being produced at slower and slower rates so that the valves are forced ever wider apart with age, creating a flattened posterior shell margin.
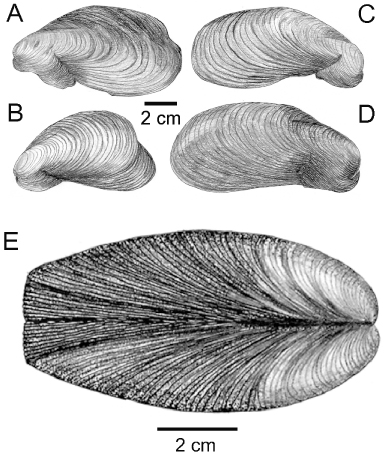
Internally, the shell of M. modiolus () is nacreous and whitish blue. The long opisthodetic, parivincular ligament is located between resilifers and comprises a darker posterior outer ligament layer (pol) and an inner ligament layer (ill) (Yonge Citation1978). This secondary ligament is formed separately from the primary ligament, as in all mytilids (Ockelmann Citation1983). Each valve margin is smooth. The umbones (u) are subterminal and set above a round anterior valve margin.
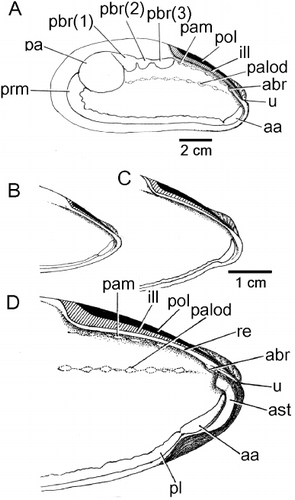
The muscle scars of M. modiolus comprise a large posterior adductor muscle (pa) and an anteriorly adjoining byssal retractor muscle scar that is divided into three major components (pbr(1), pbr(2), pbr(3)). Anterior to this is the location of the posterior pedal retractor muscle, but this cannot be identified from its scar. There is also an anterior byssal retractor muscle (abr), but the scar of this is also difficult to distinguish, as it is located under the resilifer. The pallial line is generally wide, especially posteriorly (prm). There is an anterior adductor muscle scar (aa) that is located antero-ventrally. Extending anteriorly from the posterior adductor muscle scar is a line of small scars that denote where the ascending lamella of the outer demibranch is attached to the mantle/shell (palod). Above this, underneath the resilifer and ligament, is another line of attachment scars for the mantle (pam).
provides more detailed illustrations of the internal anterior areas of the left shell valves of individuals of (B) ∼40 mm, (C) ∼75 mm and (D) ∼110 mm shell lengths. They show how this region of the shell of M. modiolus becomes progressively more thickened (ast), especially under the umbones (u) and around the anterior adductor muscle (aa). The latter is connected thickly with the pallial line (pl). Partially hidden by the hinge plate is the scar of the anterior byssal retractor muscle (abr).
Representatives of the Mytiloidea are highly variable in terms of shell form and this is particularly true of M. modiolus individuals where, with growth and age, ventral shell distortions become more frequent and evident. Such distortions appear to be induced by individuals remaining attached to substrata of limited extent, such as the shells of conspecifics, stones and kelp holdfasts. The change in posterior shell growth, from length to increasing width in larger M. modiolus individuals, as described herein, is also seen in some larger Mytilus edulis individuals and was thought to result from growth limitations due to crowding (Seed Citation1976).
The mantle
The mantle margins of Modiolus modiolus are dark brown and united only between the inhalant aperture and exhalant siphon. In transverse section (), the ventral left mantle margin posteriorly comprises the usual three folds: inner (imf), middle (mmf) and outer (omf). The latter two are small and between them is the long periostracal groove (pg), from the base of which arises the periostracum (p). The inner mantle fold is much larger, swollen and contains the pallial nerve (pn). It also contains much of the pallial retractor muscle (prm). Where it joins the general mantle epithelium internally is a ciliated rejection tract (crt) that is responsible for transporting unwanted filtered particles posteriorly where they are rejected from the mantle cavity via the inhalant aperture as pseudofaeces, as in all mytilids, for example Limnoperna fortunei (Dunker, 1857) (Morton Citation1973).
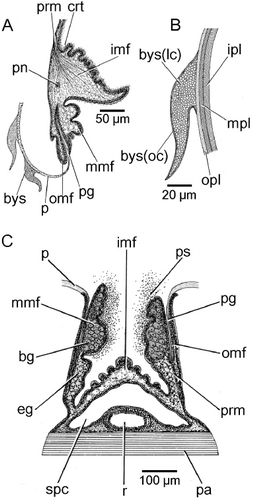
Sections through the mantle margin of M. modiolus in this region show that two byssal setae (bys) are attached to the periostracum (). Byssal setae are most likely produced and deposited by the foot and the byssal glands, for example in Mytilus and Modiolus, and are diagnostic (Ockelmann Citation1983). A more detailed illustration of a single byssal seta is provided in . Each seta is shown to comprise an outer coat (bys(oc)) enclosing a lenticular centre (bys(lc)), which also attaches it to the outer layer and surface of the periostracum. Such a lenticular structure is possibly homologous with the ‘plaque foam’ described and illustrated for the byssus of Mytilus edulis (Silverman & Roberto Citation2007: figure 4). The periostracum itself comprises three layers: a shiny yellow outer layer (opl), a middle conchiolin layer (mpl) that stains little and an inner layer that stains light red in Ehrlich's haematoxylin and eosin. Such a three-layered structure is typical of the Mytilidae, for example M. edulis (Beedham Citation1958).
is an illustration of a transverse section through the dorsal mantle margin of M. modiolus at a point above the posterior adductor muscle (pa) and rectum (r). The inner component of the mantle – i.e. the inner folds (imf), here united – enclose the supra-pericardial cavity (spc), also illustrated in . , however, also shows the separated middle (mmf) and outer mantle folds (omf), both enclosing, as ventrally (), the periostracum (p). This is secreted in the periostracal groove (pg), arising from the base of which is the pallial retractor muscle (prm). In this location, the middle mantle folds are packed with subepithelial gland cells, which are eosinophilic basally (eg) but become more basiphilic (bg) distally. These glands in the middle mantle fold can only pass their secretions (ps) to the outside of the animal. Copious amounts of this secretion are to be seen in this area of the serial sections and could, it is suggested, represent the glue that sticks sand grains to the shell (see , sg), thereby effecting camouflage. This mucous origin thus differs from that of the byssal smear on the shell of early post-metamorphosed individuals, although the foot may aid in wiping the mucoid adhesive over the shell and in depositing debris onto these protecting secretions (Ockelmann Citation1983).
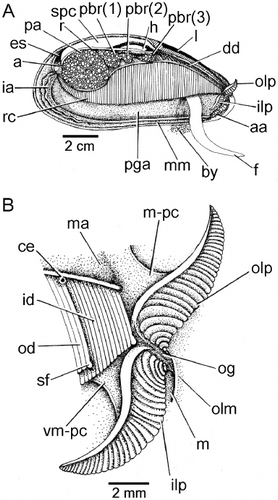
Mantle cavity
The exhalant siphon of Modiolus modiolus (, es) is dark brown and comprises a low, unadorned, cone. It is situated posterior to the posterior adductor muscle. The inhalant aperture (ia) is similarly pigmented, long, not separated from the pedal gape (pga) by pallial fusions, and is marginally apapillate everywhere.
The inhalant aperture and exhalant siphon comprise the left and right inner mantle folds that are separated from each other by fused left and right middle mantle folds. The mantle cavity is in this way divided into two chambers, supra-branchial and infra-branchial, by the paired ctenidia (rc). These are located under the posterior adductor (pa) and posterior byssal retractor muscles (pbr 1–3) and terminate posteriorly at the union of the exhalant siphon and inhalant aperture and anteriorly in a pair of labial palps – inner and outer (ilp, olp) – situated above the anterior adductor muscle (aa). Inside the exhalant siphon can be seen the anus (a) at the terminus of the rectum (r), which penetrates the ventricle of the heart (h) located above the posterior two components (pbr(1) and (pbr(2)) of the posterior byssal retractor muscles. There is a long foot (f) and a stout, stalked byssus (by). Dorsally, the dark digestive diverticulae (dd) lie underneath the ligament (l).
The ctenidia of M. modiolus are attached to the visceral mass at the ctenidial axis and are made up of two demibranchs – inner (id) and outer (od) – both of approximately equal dorso-ventral height, although the latter is slightly longer. The anterior terminus of the right ctenidium and the labial palps are illustrated in . The outer demibranch (od) is anteriorly foreshortened as in all mytilids so that only the anterior-most tips of the filaments of the inner demibranch (id) are inserted between the labial palps (ilp, olp). The terminal filaments of both demibranchs are swollen ventrally (sf), again as is typical of mytilids, for example, Limnoperna fortunei (Morton Citation1973). This is ctenidial–labial palp junction type 1 (Stasek Citation1963), the ctenidia having a ciliation of type B(I) (Atkins Citation1937). The labial palps are small and in an individual of 40 mm shell length, were ∼8 mm long and each comprised ∼32 sorting ridges. Between them is the oral groove (og) that leads to the mouth (m) hidden by the outer lip of the mouth (olm). The inner edges of the inner and outer labial palps are attached at a point approximately half way along their lengths to the visceral mass (vm-pc) and mantle (m-pc), respectively, by tissue connections. Finally, in a juvenile individual of ∼13 mm shell length, a single cephalic eyespot (ce) (Morton Citation2008) or ctenidial ocellus (Carter et al. Citation2012) is located underneath the ctenidial axis of both ctenidia at the extreme anterior end of the outer demibranch.
Visceral mass
The structure of the visceral mass of Modiolus modiolus is illustrated in as seen from the right side after removal of the right mantle lobe and ctenidium. The visceral mass is posteriorly swollen and contains the gonad (g). Antero-dorsally, the visceral mass contains the stomach surrounded by the digestive diverticula (dd). The byssal (abr, pbr) and posterior pedal (ppr) retractor muscles also penetrate the visceral mass, which ventrally is extended into a foot (f) that posteriorly produces a large, stalked byssus (by). Behind the visceral mass can be seen the left ctenidium (id, od). The heart (h) is, as already described, located above the anterior-most components of the posterior byssal retractor muscle (pbr(1) and pbr(2)).
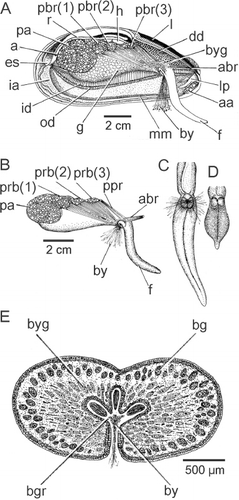
The byssal and pedal musculature of M. modiolus is seen from the right side in . The posterior byssal retractor (pbr) is illustrated in connecting up intra-pedally with the byssus (by). The paired posterior pedal retractor (ppr) and the anterior byssal retractor (abr) muscles are tiny in comparison. The foot (f) is long and thin and is illustrated from the posterior aspect in extended and retracted conditions in and , respectively. The foot is also illustrated in transverse section in . There is a distinct byssal groove (bgr), which is trefoil-shaped in transverse section and contains the byssus (by). The byssus is secreted by a byssal gland that comprises eosinophilic cells some 20 µm in length and arranged around the byssal groove. This was termed the ‘white’ or ‘collagen’ gland in the foot of Mytilus edulis by Brown (Citation1952) and Pujol (Citation1967). There is, however, a second gland that is arrayed subepithelially beneath the outer surface of the foot and comprises elongate, basiphilic, cells some 25 µm in length. These cells, the ‘purple’ gland of Brown (Citation1952), probably secrete mucus, although Pujol (Citation1967) thought it might be ‘enzymatic’ and responsible for tanning the collagen produced by the ‘white’ gland.
The intestine and stomach
The organs of the pericardium and the course of the intestine in Modiolus modiolus are seen from above in . The stomach (st) is located anteriorly and from its posterior margin arises the conjoined style sac and midgut (css). This passes under the heart and between the paired byssal retractor muscles (pbr(1)–(3)). Here, the midgut (mg) separates from the style sac and passes anteriorly on the left side of the body and then passes posterior to the oesophagus (o) and paired anterior byssal retractor muscles (abr) to the left side of the body and becomes the hindgut (hg). It now penetrates the ventricle (v) of the heart, with left and right auricles (au), as the rectum (r), which extends posteriorly again between the paired anterior byssal retractor muscles, under the paired kidneys (k) over the posterior adductor (pa) where it terminates on the posterior face of this muscle in an anus (a).
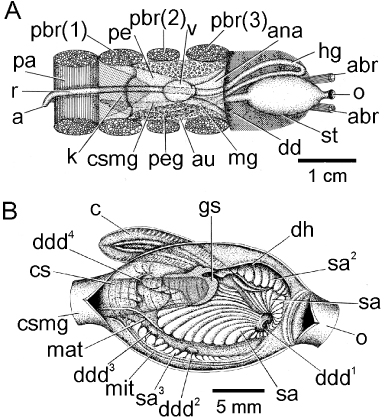
The stomach of M. modiolus () was described first by Nelson (Citation1918), who illustrated the ciliary currents of the interior (figure 12) and the food sorting caecum (figure 15). He also illustrated the structure of the small gastric shield (figure 11). Graham (Citation1949) undertook the first detailed and comparative study of the molluscan stomach, describing the internal architecture of that of Mytilus edulis (fig. 4). Purchon (Citation1957) only briefly added to Graham's account of the M. edulis stomach and defined it as belonging to his subclass Polysyringia, characterized by many openings into it from the digestive diverticula. The Polysyringia was further divided into three orders, with M. edulis and M. modiolus belonging to the Gastrotriteia. Reid (Citation1965) redescribed the stomach of M. edulis and described the ciliary currents of the food sorting caecum of M. modiolus (figure 6) noting that it was shorter than that of M. edulis. Hence, the interior architecture of the stomach of M. modiolus has only been described by Nelson (Citation1918) and thus has not had applied to it the nomenclature that the British school of stomach anatomists created.
The stomach of M. modiolus is illustrated from the right side in . The oesophagus (o) opens into it anteriorly and the conjoined style sac and midgut (csmg) depart it posteriorly. From this opening arises the rod-like crystalline style (cs) that rotates against the small gastric shield (gs), as described by Nelson (Citation1918). Beneath the entrance to the oesophagus is a sorting area (sa) that encircles the first major entrance to the digestive diverticula (ddd1) on the antero-ventral floor of the stomach. The floor of the stomach is also characterized by a sorting area (sa) that is fringed on its right side by the major typhlosole (mat) and which, travelling posteriorly, enters the aperture of the conjoined style sac and midgut. The right side of the major typhlosole has a channel which constitutes the intestinal groove, and herein arise two more, small, openings to the digestive diverticula (ddd2 and ddd3). The right side of the stomach has a sorting area identified by Purchon (Citation1957) as sa3. Above this is situated the minor typhlosole (mit), which also enters the aperture of the conjoined style sac and midgut. To the left of the aperture to the conjoined style sac and midgut is a large opening to the digestive diverticula (ddd4). The left side of the stomach is characterized by the entrance to the sorting caecum (c), which is fringed dorsally by an element of the gastric shield. Further anteriorly is the capacious dorsal hood (dh), the entrance to which is a sorting area defined by Purchon (Citation1957) as sa2. The internal architecture of the stomach of M. modiolus, therefore, is characterized by a large number of sorting areas, including a sorting caecum, that allow the species to select which particles will be sent for intracellular digestion in the digestive diverticula following trituration and extracellular digestion by the rotation of the crystalline style against the gastric shield, both of which are enzymatically active.
The pericardium
The organs of the pericardial cavity of Modiolus modiolus are illustrated in from the right side. The course of the rectum through the medial ventricle (v) of the heart and between the posterior byssal retractor (pbr(1)–(3)) and over the posterior adductor (pa) muscles is as described above. Posterior and ventral to the heart are the paired brown kidneys (not illustrated here for clarity). The epithelia of the left and right auricles (au) of the heart contain the light brown pericardial gland (peg). The renal apertures, which are shared with the gonadal apertures (r-ga), open onto the visceral mass to the left and right between the posterior byssal retractor and posterior pedal retractor (ppr) muscles. The ascending lamella of the outer demibranch of the right ctenidium attaches to the mantle/shell (palod) at the junction between the visceral mass and mantle. The ctenidial axis (ca) and the point of union of the ascending lamella of the inner demibranch of the right ctenidium with the visceral mass (palid) are illustrated and it can be seen that the renal/gonadal aperture opens into the supra-branchial chamber between these two points. Modiolus modiolus is dioecious, the large gonads being located in mesosomal lobes in the visceral mass. Unlike in Mytilus edulis (White Citation1937), the gonadal tissues of M. modiolus never invade the mantle, ctenidia, nor the foot. The male gonadal tissue is pale yellow and in females orange–red. Hermaphroditism has been reported for a small fraction (< 8%) of individuals of M. modiolus in some populations (M'Intosh Citation1894; Wiborg Citation1946; Comely Citation1978; Jasim & Brand Citation1989), but not protandry.
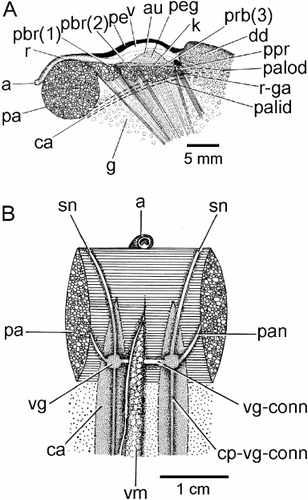
Underneath the posterior adductor muscle (pa) can be seen the visceral ganglia (vg) (). These are connected by the visceral ganglia connective (vg-comm) and each anteriorly and laterally sends nerves to the siphonal apparatus (sn) and the adductor muscle (pan), respectively. These ganglia and their connections (cp-vg-conn) to the cerebral ganglia anteriorly are hidden by the ctenidial axes (ca) located left and right of the visceral mass (vm).
Biology
Environmental adaptations
The Mytiloida have radiated into three major habitats, each occupied by distinctive representatives of three subfamilies: the Mytilinae (Mytilus) in the intertidal, Modiolinae (Modiolus) on the subtidal shelf and the Bathymodiolinae (Bathymodiolus) primarily in the deep sea at hydrothermal vents, cold seeps and organic falls. Phylogenetically, Mytilus forms a clade with Adula (Ockelmann & Dinesen Citation2009). The Mytilinae possibly forms part of one of two major clades within the Mytilidae, with the Modiolinae and Bathymodiolinae attributed to the other (Samadi et al. Citation2007). Studies of the molecular affinities of deep-sea mytilids have suggested that Modiolus modiolus forms a basal node to a monophyletic Bathymodiolinae (Distel Citation2000; Samadi et al. Citation2007; Lorion et al. Citation2009, Citation2010). Shell microstructure features did not challenge this view (Genio et al. Citation2012). Different conclusions (Ritt et al. Citation2012) may have arisen from a misconception that speices of Benthomodiolus Dell, 1987 are classified within the Modiolinae rather than the Bathymodiolinae. Life history traits suggest that the Modiolinae form a sister group basal to the bathymodiolines (Ockelmann & Dinesen Citation2011).
The shells of the three habitat-forming mytilids – that is, M. modiolus (A), Mytilus galloprovincialis (B) and Bathymodiolus thermophilus (C) – all drawn to the same scale, are illustrated from the posterior aspect in and wherein x–y represents the point of greatest shell width. The shell of M. modiolus is dorsally pointed but inflated in the middle, dorso-ventral region at a point approximating the position of the umbones (u). This reflects, as described and discussed above, the variety of endo- and epi-byssate lifestyles adopted by this species both subtidally and occasionally intertidally. In contrast, Mytilus galloprovincialis has a smoothly uniform shell outline but is most inflated towards the ventral region of the shell, above the umbones, which are situated ventrally. This reflects the occupation by this species of the wave-exposed intertidal and a gregarious lifestyle wherein individuals are crowded together basally but have to maintain a long extent of projecting shell to facilitate feeding and respiration. Again in contrast, but approximating a shell form intermediate between the two other habitat-forming mytilids, B. thermophilus has a dorsally pointed shell (like M. modiolus) but is most inflated laterally at a point just above the mid-point of the dorso-ventral axis of the shell and coinciding with the position of the umbones. Ventral to this mid-point, the shell tapers more acutely than in M. modiolus. This shell form too reflects the gregarious, habitat-forming lifestyle of B. thermophilus in the deep sea in that, although it has a shell form reminiscent of M. modiolus, it is more inflated mid-laterally, probably to house the greatly inflated ctenidia that contain chemosymbiotic bacteria (Kenk & Wilson Citation1985).
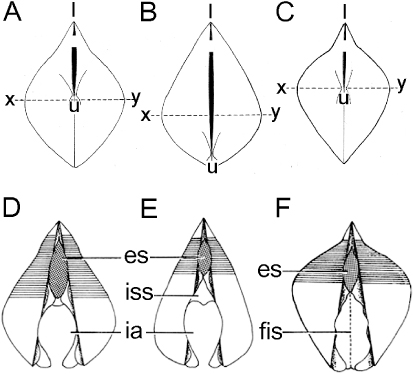
The posterior regions of the shell valves and the entrance and exit to and from the mantle cavity of M. modiolus (A), M. galloprovincialis (B) and B. thermophilus (C) are illustrated diagrammatically in . In all cases, the exhalant siphon (es) comprises a low, unadorned, cone. It is situated posterior to the posterior adductor muscle and, in each case, approximates this in its dorso-ventral dimensions. The inhalant apertures (ia) of M. modiolus and M. galloprovincialis are long, not separated from the pedal gape by pallial fusions, and are marginally apapillate. In the Mytilidae, the entrance to the inhalant aperture is typically reduced by a siphonal septum (iss), as illustrated in for M. galloprovincialis. Modiolus modiolus does not possess this structure, but, interestingly, the presence of the septum in the former but not the latter results in the inhalant apertures of both suspension feeders being of the same relative sizes. The septum thus serves in the intertidal, habitat-forming Mytilus edulis to maintain an appropriate inhalant aperture size and thereby serves to limit it to this dynamic environment. Also interestingly, B. thermophilus does not possess an inhalant aperture, this being closed by fusion of the inner mantle lobes (Kenk &Wilson Citation1985). This is related in this species to the possession of ctenidial populations of chemosymbiotic bacteria.
Climatic adaptations
Modiolus modiolus has a boreal distribution (Jeffreys Citation1863; Jensen Citation1912; Soot-Ryen Citation1955; Bernard et al. Citation1993; Coan et al. Citation2000) and occurs from the lowest intertidal to depths of ≥ 200 m. Byssally attached, M. modiolus live either on or in a variety of substrata (Roberts Citation1975; Wildish et al. Citation2009). Individuals may be: (1) epibyssate on bedrock, boulders, cobbles, shell debris and other conspecifics; (2) partly buried/embedded in sand, gravel, pebbles and shell gravel; or (3) endobyssate in silty sand with scattered elements of gravel and pebbles. Modiolus modiolus commonly occurs on horizontal to gently sloping faces, but may also inhabit vertical faces, including Forties Bravo at ∼25 m (Anwar et al. Citation1990) and Kaldbak Fjord, the Faroe Islands, at 15–25 m.
The few individuals of M. modiolus found either in intertidal rock pools or at depths > 250 m (Davenport & Kjørsvik Citation1982; Dinesen & Ockelmann Citation2005) are most likely meta-population sinks rather than sources. Intertidal populations have been reported from the northern limits of its distribution in tidal rock pools and on north-facing shores where solar radiation is lowest and often where there is a growth of large kelp algae, such as species of Laminaria and Fucus, underneath which individuals are covered during low-tide periods. Only small populations have been found in rock pools in North Wales (Wilson Citation1977) and intertidally in northern Norway (Davenport & Kjørsvik Citation1982) and Northern Ireland (Brown & Seed Citation1977; Seed & Brown Citation1977), and on north-facing shores under kelp in Faroese fjords (Dinesen & Ockelmann Citation2005).
Notwithstanding its restricted intertidal occurrence, M. modiolus is most likely related to low tolerances of aerial exposure, desiccation and fluctuating temperatures, as compared with M. edulis (Winter Citation1970). During exposure to low seawater temperatures of between −15 and −22°C for 12 hours in vitro, adult M. modiolus individuals survived between 65% and 71% water loss caused by extracellular ice formation in the tissues (Kanwisher Citation1955, Citation1959). During aerial exposure of some M. modiolus individuals, heart rates slowed and became irregular and, after re-immersion, took between 30 and 45 min to return to normal (Coleman & Trueman Citation1971, Coleman Citation1973). In the same experiments, M. edulis also reduced its heart rate, but the shell valves remained closed and recovery was affected within a few minutes of re-immersion. Coleman (Citation1973) also showed that relative water loss is 15–20% higher in M. modiolus than in M. edulis of equal shell lengths (64–73 mm), but is of the same range in the largest individuals of both species – i.e. shell lengths of between 64 and 73 mm in M. edulis and 104 and 115 mm in M. modiolus. From in situ experiments with juvenile M. modiolus (SL < 20 mm), Gillmor (Citation1982) showed that growth was zero at aerial exposure times of 20–25%. In comparison, M. edulis showed zero growth after 80% aerial exposure. Gillmor (Citation1982) also recorded 100% mortality of M. modiolus at 40% aerial exposure. It is, however, unclear whether these results are due to desiccation or to food shortages, or both, because of a reduced filter-feeding time, and such conditions are unlikely to occur in the natural habitat of the species. Declines in British subtidal populations have been thought to be related to a susceptibility to increasing summer seawater temperature (Hiscock et al. Citation2004). Raised seawater temperatures could reduce reproductive output, larval fitness and survival to maturity of benthic, boreal species, particularly at the southern limits of their distribution.
In estuarine systems, such as the Baltic Sea, salinity probably restricts the subtidal distribution of M. modiolus to the belt seas (Middelfart, Svendborg Sund) and Øresund (Skovshoved) (Petersen Citation1913, Citation1918; Thorson Citation1950, Citation1957, Göransson & Karlsson Citation1998; Göransson et al. Citation2010), while irregular influxes of high-salinity water further inwards, such as in Kieler Bay (Winter Citation1970), may sustain minor sink populations. Davenport & Kjørsvik (Citation1982) showed that M. modiolus could survive short immersion periods in salinities of 16 by keeping the shell valves closed and, thereby, keeping pallial fluids at a salinity of 28–29. However, Schlieper et al. (Citation1958) demonstrated that after 3 days at a salinity of 15 and 10°C, ctenidial ciliary stroke was much weakened in M. modiolus whereas M. edulis was unaffected. Short-term tolerances to a salinity of 15 were similarly identified for White Sea M. modiolus, whereas salinity levels of between 30 and 35 appeared optimal (Bakhmet et al. Citation2012). Gainey (Citation1994) showed that M. modiolus has a lesser capability to adapt to reduced salinities in comparison with the intertidal M. edulis. The relative weight of M. modiolus also increased with decreasing salinity (at 10°C) because of an increase in intracellular liquid/water, whereas it remained unchanged in M. edulis (Gainey Citation1994). Lange (Citation1970) and Pierce (Citation1970) provided similar results for M. modiolus. From the Baltic Sea distribution pattern, it can be inferred that the lower, long-term salinity tolerance of adult M. modiolus is likely to be ∼26. In comparison, the salinity tolerance for M. edulis is ≥ 5–6 (Schlieper et al. Citation1958). In conclusion, therefore, it appears that low salinities limit the subtidal distribution of M. modiolus in brackish-water systems.
Food availability and feeding behaviour
In subtidal, high-salinity waters, food shortages may be important in explaining the distribution of Modiolus modiolus. That is, the southernmost distribution of the species could be limited by increased metabolism with increased temperatures, which are not matched by an increased availability of food. Conversely, the northernmost distribution could be limited by an abbreviated period of primary production. Lesser & Kruse (Citation2004) found reduced respiration during the winter months. At high latitudes it is, therefore, possible that reduced respiration during a longer winter period may not compensate sufficiently for the reduced food availability. This trade-off between food availability and metabolic rate would influence growth and survival of the planktotrophic larvae and early juveniles, as well as the growth and reproductive output of adult individuals.
In boreal waters, food availability for suspension feeders is linked closely to seasonal primary production, which may vary at the local scale due to prevailing hydrographic conditions. Adult M. modiolus occur commonly in areas with moderate to high water exchange in Nova Scotia (Wildish & Peer Citation1983; Wildish & Kristmanson Citation1985, Citation1994; Wildish & Fader Citation1998; Wildish et al. Citation1998), and low field densities have been correlated with low current regimes and reduced food availability. Densities of up to 220 individuals/m2 have been recorded from the Faroese shelf (Dinesen Citation1999) where maximal tidal current speed has been estimated to be between 79 and 98 cm/s at two M. modiolus sites (Nørrevang et al. Citation1994: BIOFAR Stn. 661 & 662). In the Gulf of Maine, however, individual growth of M. modiolus depended on concentrations, rather than flux, of particulate organic matter, which is the opposite of the situation in the (passive) suspension-feeding sea anemone, Metridium senile (Linnaeus, 1761) as described by Lesser et al. (Citation1994).
In vitro filter-feeding experiments at 10–12°C and a salinity of 30 showed that at a particle size of ≥ 4 µm, M. modiolus retained 100% while at ≥ 2 µm only 80% was retained, and even less at smaller particle sizes (Møhlenberg & Riisgård Citation1978, Citation1979). Filtration rates as a function of soft tissue dry weight were lower than in Mytilus edulis (Møhlenberg & Riisgård Citation1979). Prolonged periods of darkness alternating with periods of light appeared to favour growth of M. modiolus from the Norwegian west coast at 63°N (Strömgren Citation1976). If this were a common pattern it would be expected to affect growth negatively in shallow waters at high latitudes. Preliminary studies by Earll (Citation1976) indicated that M. modiolus spent ≥ 95% of the time actively filtering. This is in contrast to the results of Winter (Citation1969, Citation1970), who showed that, over a 24 hour period, individuals of M. modiolus experienced two periods of active filter-feeding and two periods of digestion, corresponding to the prevailing tidal regime. In the latter, in vitro studies with two algal cultures (particle sizes between 5 and 10 µm), filtration rate and particle uptake increased when temperatures increased from 4 to 20°C. With an increased particle concentration from 10 to 40 × 106, the filtration rate decreased and pseudofaeces production increased. Navarro & Thompson (Citation1994) demonstrated that there was a risk of underestimating the absorption effectiveness of M. modiolus when the ratio of inorganic versus organic matter was low. In situ, M. modiolus may select particles qualitatively and not solely on the basis of size. Navarro & Thompson (Citation1996, Citation1997) demonstrated, moreover, an annual variation in filtration capacity with increased clearance rates recorded during spring and summer, regardless of individual size, and correlated this with a phytoplankton bloom.
Growth and longevity
Food availability may also influence the growth rate and individual maximum size of Modiolus modiolus, which grows to a shell length of between 65 and 230 mm, depending on locality. Numbers of growth bands may be useful in establishing the growth rate of younger individuals, especially if there are references to growth of marked (capture–mark–recapture) shells (Seed & Brown Citation1978; Anwar et al. Citation1990). Anwar et al. (Citation1990) also established growth lines for seven populations of M. modiolus (Forties Bravo, 25 m; West Scotland, subtidal; northern North Sea, 73–77 m; southern North Sea, 20 m; Northern Ireland, 11–16 m; Isle of Man, subtidal; Irish Sea, 80–90 m), and compared them with five other populations from West Scotland (Comely Citation1978), wild stocks (Seed & Brown Citation1975) and from cages (Brown & Seed Citation1977), North Wales (Wilson Citation1977) and Norway (Wiborg Citation1946), the latter with growth rates similar to those reported in Lande et al. (Citation1973). The lowest growth rate was recorded from the deepest population (Comely Citation1978), that is, individuals possessing 20 growth rings were of ∼120 mm shell length at 10–15 m, ∼105 mm at 1.5 m and only 65 mm at 180–200 m. In this study from four Scottish sites, a maximum shell length of ∼160 mm was reported from 10 to 15 m, whereas at 1.5 m it was slightly smaller at < 150 mm, and in deep waters between 180 and 200 m it was < 85 mm (Comely Citation1978). Maximum shell lengths of 100, ∼95 and 80 mm have been recorded from Strangford Lough, Friday Harbour and the Isle of Man, respectively (Brown & Seed Citation1977). Anwar et al. (Citation1990) showed that the maximum shell length was 110, 95 and 80 mm at Forties Bravo (25 m), Isle of Man (subtidal) and the Irish Sea (80–90 m), respectively. In the Faroese Kaldbak Fjord (15 m), the maximum recorded shell length was ∼150 mm (Dinesen Citation1996), whereas on the Faroese shelf between 60 and 90 m it was ∼95 mm. Wildish et al. (Citation2009) showed that individual growth rates during the early years of life differ between substrates, with faster growth rates on sand with bioherms and gravel compared with on cobbles and mottled gravel in the Bay of Fundy, northwestern Atlantic.
Individuals of M. modiolus of 100 mm shell length from Northern Ireland were estimated to be between 14 and 29 years old (Seed & Brown Citation1975, Citation1978). Individuals from Shetland of 100 mm shell length were estimated to be between 11 and 17 years old (Comely Citation1981), and those from Norway were 10–18 (13–19) years old (Wiborg Citation1946). In Norway, M. modiolus commonly reaches an age of 22–23 years, with one individual estimated to be 36 (Wiborg Citation1946). Anwar et al. (Citation1990) established that the oldest individual studied, from the northern North Sea at a depth of 73–77 m, was ∼48 years old.
Reproduction
Reproduction and larval development of Modiolus modiolus has been studied by Nordgaard (Citation1901), Williamson (Citation1906), Rowell (Citation1967), Seed & Brown (Citation1975, Citation1977, Citation1978), de Schweinitz & Lutz (Citation1976), Brown & Seed (Citation1977), Lutz & Hidu (Citation1979), Comely (Citation1981), Brown (Citation1984) and Fuller & Lutz (Citation1989). Modiolus modiolus is dioecious with no apparent, externally identifiable, sexual dimorphism. Reproduction and spawning duration vary between depth and location (). Eggs and sperm are released in synchronized broadcast spawning events with fertilization taking place in the water column (de Schweinitz & Lutz Citation1976). Individual M. modiolus may spawn epidemically within 24 h and/or with several years in between (Lilleskare Citation1905; Wiborg Citation1946). Depending on the state of maturity, male and female gonads are light to strongly yellow and light to strongly red–orange, respectively (Wiborg Citation1946). Gonad development and spawning length in the Northeast Atlantic and adjacent seas vary between locations (). In Norway, M. modiolus has been reported to become sexually mature at 3 years of age, although most individuals do so at an age of 5–6 (and up to 8) years (Wiborg Citation1946). Sexual maturity is independent of shell length between localities. Around the Isle of Man, the youngest mature individuals were 3–4 years old (Jasim & Brand Citation1989). In Canada, the earliest mature individuals were four years old, and most individuals did not reach maturity until the age of 7–8 years (Rowell Citation1967). In Northern Ireland, most individuals mature at a shell length of 40–50 mm (∼4–6 years), but some were already mature at a shell length of 10–20 mm (Seed & Brown Citation1977). Here, there appears to be a ‘catch-up’ phenomenon in maturing M. modiolus, that is, the simultaneous maturing of the remaining immature individuals in the population. This occurs at a shell length of ∼60 mm, at the time when variation is smallest in tissue dry weight, shell weight and shell length (Brown et al. Citation1976; Brown & Seed Citation1977).
Table I. Modiolus modiolus. Variation in the timing of spawning and gonadal maturation in relation to depth and geographic location (na: information not available; months abbreviated).
Sex ratio has been recorded as close to 1:1 for M. modiolus on the Norwegian west coast (Wiborg Citation1946), Scotland (Comely Citation1978), Isle of Man (Jasim & Brand Citation1989) and the Faroe Islands (Dinesen Citation1996). In some populations, however, there are more females than males. In some Norwegian populations, for example, Wiborg (Citation1946) showed that the ratio can be 1.26:1 or 1.7:1. At a single locality, the sex ratio differed between three size classes, that is, at shell lengths of 25–34, 35–44 and 45–54 mm it was 1:2.75, 2:1 and 1.3:1, respectively, and for the total population it was 0.94:1. This indicates a size differential in gender maturity. In a Faroese shelf population, males appear to mature at a smaller shell length of 32 mm than females (SL ∼50 mm) (Dinesen, personal observations).
Experiments with induced in vitro spawning in M. modiolus, for example by a temperature increase, injection of either 0.5 mol KCl or the release of gametes from other individuals, did not succeed (de Schweinitz & Lutz Citation1976). An abrupt and considerable temperature increase (to 25°C) was, however, effective in maturing M. modiolus (Jasim & Brand Citation1989).
The onset and duration of spawning in M. modiolus varies considerably between localities (). Brown (Citation1984) compared gametogenesis and spawning season in four subtidal populations of M. modiolus from a depth of 15 m and showed that both may occur simultaneously. Spawned eggs from Strangford Lough, Northern Ireland, measured 60–75 µm in diameter and, hence, eggs > 60 µm were considered mature. In Strangford Lough too, gametogenesis and spawning may occur throughout the year, with peak months varying between years. Comparison with a local intertidal population showed a peak in gonad maturity and spawning during the summer months (Seed & Brown Citation1977). In the Gullmar Fjord, on the Swedish west coast, spawning takes place in June with subsequent periods of gametogenesis and spawning occurring until mid autumn. Subsequently, gametogenesis declines until the following spring. In Bergen, western Norway, spawning occurs in June, with subsequent periods of gametogenesis and repeated spawning occurring until autumn. Gametogenesis begins again late in winter. In Tromsø, northern Norway, spawning occurs only from July to September and is followed by a return to gametogenesis and ∼75% of individuals over-wintering with mature gonads (Brown Citation1984). The intertidal population of M. modiolus in Belfast Lough, Northern Ireland, showed a clear seasonal variation in its reproductive cycle. Spawning season was considerably shorter than in the subtidal Strangford Lough population (4–9 versus 12 months) (Brown & Seed Citation1977; Seed & Brown Citation1975, Citation1977). Intertidal environments experience larger annual temperature variations as well as a lower average and/or accumulated temperature and such findings do not, therefore, contradict the correlations identified by Brown (Citation1984). The population of M. modiolus at Tromsø, Norway, where the spawning season is short, was ready to spawn with the onset of summer.
Variations between the onset and duration of gametogenesis and spawning have been correlated with depth and geographic distribution (de Schweinitz & Lutz Citation1976; Seed & Brown Citation1977). Geographic differences play an important role in the timing of maturity and there appear to be differences between populations even within short distances at similar depths. Similarly, populations in the same area but at different depths show variations. A negative correlation between annual temperature range and the relative frequency of mature oocytes was identified and explained as variability in temperature adaptation in different populations. Brown (Citation1984) also obtained a positive correlation between spawning season duration and accumulated temperature regime (day °C−year). This could be an adaptation to food availability for the planktotrophic larvae.
Larval development and settlement
Larval development of Modiolus modiolus is planktrotrophic, with a short trochophore stage prior to the veliger stages, Prodissoconch I and II, and takes ∼4 weeks from fertilization to competency. During the Prodissoconch II stage, the larva develops several structures, including a pair of ocelli and a foot (de Schweinitz & Lutz Citation1976), which are retained during benthic, post-metamorphic, life. Sources of and sink areas for recruitment are influenced by prevailing hydrographic conditions and current dynamics. The Strangford Lough populations appear to be self-recruiting (Brown Citation1990; Elsässer et al. Citation2013), and hence the hypothesis that most M. modiolus populations depend on external recruitment needs testing. Gregarious recruitment was first suggested by Roberts (Citation1979). Dinesen & Ockelmann (unpublished data) observed recently that competent larvae settle preferentially in response to the exhalant water of adults. In declining populations, this behaviour would not only result in fewer recruited offspring but also the strength of the signal to settling larvae would be lessened, leading eventually to recruitment failure. Following settlement, the larva undergoes metamorphosis and lays down a nepioconch shell. Some prodissoconch and nepioconch features were described by Jukes-Brown (Citation1905), Rees (Citation1950), Lutz & Hidu (Citation1979), Ockelmann (Citation1983, Citation1995) and Fuller & Lutz (Citation1989). At a shell length of ∼750 µm the dissoconch shell is formed, with a different structure and dentition. For details of prodissoconch and nepioconch features, see Fuller & Lutz (Citation1989) and Ockelmann (Citation1995).
The byssal gland and ontogenetic changes of function
Mucous strings of many molluscan larvae, such as Mytilus edulis, enhance particle capture during planktonic feeding and may serve as a floatation device whereby the larva can tether itself in an otherwise liquid environment (Fenchel & Ockelmann Citation2002). Upon settlement on the seabed, the trailing mucous string functions as an anchor and attachment device (Abelson et al. Citation1994). During ontogeny, the Modiolus modiolus foot undergoes functional changes, and mucous string-producing cells at the base of the larval foot are thought to be a precursor of the byssal gland complex of juvenile and adult individuals. Post-metamorphosis, mucous strings are laid down by the foot across the shell, for both attachment and camouflage (Ockelmann & Dinesen, submitted). Within 10 days, at a shell length of ∼750 µm, the byssal gland complex of the foot begins to produce threads for attachment and setae – the latter possibly for protection against predators.
Byssal thread production in adult M. modiolus (individual SL of 75 mm, San Juan Island, Washington) is inhibited at water flow velocities exceeding 16 cm/s (Carrington et al. Citation2008). Therefore, strong currents could limit the occurrence of individuals outside the aggregations, inside which velocities are much reduced. The length and number of byssal threads produced by adult M. modiolus are higher than in M. edulis, regardless of sediment grain size (Meadows & Shand Citation1989; Pearce & LaBarbera Citation2009). These authors suggested that such a difference might be explained by: (1) endobyssate (Modiolus) versus epibyssate (Mytilus) habits; (2) the different maximum sizes achieved by the two species; or (3) adult M. edulis are highly mobile and detach their byssal threads frequently whereas adult M. modiolus are not known to do this. As M. modiolus individuals are often epifaunal where the substratum allows it, the first explanation appears less likely. The latter two explanations are plausible, as larger individuals of the same species produce more byssal threads and adult M. modiolus are more sedentary. However, a fourth explanation may be that byssus production is related to predator avoidance. While this has not been studied for M. modiolus, the numbers of byssal threads produced by Perna viridis (Linnaeus, 1758) increased when individuals were exposed to pheromones of the crab predator Thalamita danae Stimpson, 1858 (Cheung et al. Citation2004). Other mytilid anti-predator devices include a thick periostracum (Harper & Skelton Citation1993) and byssal setae (Wright & Francis Citation1984), the latter seeming particularly pertinent with regard to M. modiolus, as herein described and discussed.
The mucous smear, or glue, often found particularly on the posterior region of the shell of larger juveniles of M. modiolus (SL ≥ 10 mm) and adults, may be produced by glands in the middle mantle folds beneath the postero-dorsal regions of the shell () and described herein for the first time for mytilids. Alternatively, the glue may be produced by the basiphilic mucous cells of the foot (). Wherever it is produced (perhaps from both areas), the mucous smear may serve a similar function as the initial byssal smear laid down by the foot in the newly settled spat – i.e. camouflage and the additional protection afforded by the sand grains that become attached to the shell as the individual ages.
Byssal setae have been considered to be of periostracal origin, that is, ‘periostracal setae’ or ‘hairs’ (Bottjer & Carter Citation1980; Dixon et al. Citation1995), but are actually laid down by the foot as in species of Mytilus (Board Citation1983), Dacrydium (Ockelmann Citation1983) and Adula (Ockelmann & Dinesen Citation2009). The byssal setae of M. modiolus are structurally similar to and homologous with the distal regions of a byssal thread – i.e. the ‘plaque foam’ of Silverman & Roberto (Citation2007). This distal region of a thread comprises a primary layer attached to a surface – the plaque foam – and a more proximal region of collagen that, yet further proximally, unites into a bundle (or stem) attached to the byssal retractor muscles (Silverman & Roberto Citation2007). The byssal setae of M. modiolus thus terminate as a tapering thread of, presumably, collagen. The sticky mucous smear may serve to camouflage the exposed posterior regions of the shell through sand grain attachment. It may also prevent a firm ‘foot grip’ by gastropod predators.
Predation and defence mechanisms
In the Northeast Atlantic, the known gastropod predators of Modiolus modiolus are Buccinum undatum Linnaeus, 1758 and Neptunea antiqua (Linnaeus, 1758) and, possibly, Nucella lapillus (Linnaeus, 1758) intertidally (Wiborg Citation1946; Sebens Citation1985).
In vitro experiments have shown that N. lapillus prefers to prey on Mytilus edulis rather than M. modiolus (Wright & Francis Citation1984). However, if the byssal setae are scraped off the shells of M. modiolus, this preference becomes less obvious. This suggests that either the byssal setae provide some protection from the drilling activities of N. lapillus or prevent the predator obtaining a good grip on the shell. It is also possible that after failed attacks, the gastropod recognizes M. modiolus with byssal setae as ‘bad’ prey (Wright & Francis Citation1984). In addition, the explanation for the reduced frequency of successful attacks could be the thicker and more solid shell and/or periostracum of M. modiolus compared with that of M. edulis. In the study by Wright & Francis (Citation1984), the byssal setae of M. modiolus were scraped off using a razor blade, which could have damaged the bivalve's periostracum, thereby allowing the gastropod to attack more easily and drill the shell more successfully. In older individuals with thicker shells, the periostracum is often damaged postero-dorsally above the digestive diverticulae. Harper & Skelton (Citation1993), studying other bivalves, showed that N. lapillus preferred drilling M. edulis in this highly nutritious area and, especially, those individuals where the periostracum had been either thinned or removed through abrasion. However, Morton (Citation2011) showed that this was not the case and that this region of the mytild's shell was attacked by the muricid because it was the most accessible. Kabat (Citation1990) cited experimental work wherein drilling naticids could not distinguish between artificially thinned shells and intact conspecifics, although optimal foraging and prey handling may be learned. Drilling gastropods are considered to have difficulties in mechanically accessing a thick periostracum (Wright & Francis Citation1984; Harper & Skelton Citation1993), possibly because of resistance to secretions from the accessory boring organ.
Roberts (Citation1975) showed that at lengths of < 60 mm, shell strength is higher in M. edulis compared with M. modiolus, which may also assist in countering the attacks of such drilling predators. Adult M. modiolus may keep their valves closed for long enough to make Buccinum undatum abort an attack, so that only individuals with corroded and, thereby, thinned shells are eaten. Buccinum undatum is known to use the rim of its shell to break the valves of M. modiolus, whereas, conversely, the valves of large, thick-shelled individuals may tighten so firmly as to result in breakage of the predator's shell, thereby leaving scars on it (Nielsen Citation1975; Roberts Citation1975; Seed & Brown Citation1975).
Carcinus maenas (Linnaeus, 1758) predates young M. modiolus (Seed & Brown Citation1975) by cracking their shells with its chelae. Juvenile M. modiolus of ≤ 25 mm and ≤ 35 mm shell length are also attacked by Pagurus bernhardus (Linnaeus, 1758) and Liocarcinus depurator (Linnaeus, 1758), respectively (Roberts Citation1975). Necora puber (Linnaeus, 1767) is a known predator of juvenile M. modiolus too (Roberts Citation1975). Cancer irroratus Say, 1817 and Homarus americanus H. Milne Edwards, 1837 are both considered significant predators of adult M. modiolus (Jamieson et al. Citation1982).
The seastar Asterias rubens Linnaeus, 1758 predates M. modiolus of ≤ 50 mm shell length (Wiborg Citation1946; Roberts Citation1975; Seed & Brown Citation1975), whereas in the Northwest Atlantic juveniles fall prey to Asterias vulgaris Verrill, 1866 (Sebens Citation1985). In Kaldbak Fjord up to five individuals of A. rubens have been observed in situ attached to and eating a large M. modiolus individual (≥ 120 mm shell length; Dinesen, personal observations). Wiborg (Citation1946) suggested that Marthasterias glacialis (Linnaeus, 1758) predated M. modiolus, although Jensen (Citation1966) identified sea urchins, such as Strongylocentrotus droebachiensis (O.F. Müller, 1776), as their main predator.
Gardiner (Citation1927) listed the parasites of M. modiolus, none of which are apparently obligatory. Some species of pea crab, Pinnotheres, are well-known mantle cavity-dwelling commensal and/or parasites that reduce the growth of their host (Wiborg Citation1946; Brown & Seed Citation1977; Comely Citation1978).
Several fish species have been caught together with M. modiolus aggregations where they were thought to find shelter and food (Wiborg Citation1946; Roberts Citation1975). Jensenius Madsen (Citation1949) reported from Icelandic waters that small individuals were often found in fish stomachs. The negatively skewed population structure of M. modiolus indicates that a higher predation pressure exists for smaller individuals than adults, but the quantitative significance of this is unknown.
Habitat structure
Modiolus modiolus forms aggregations that range from a few individuals grouped in small clusters to extensive beds and reefs with densities reaching up to 400 individuals/m2 (Lindenbaum et al. Citation2008) and stretching patchily for between several square metres to kilometres of the subtidal shelf (Dinesen Citation1999; Bruntse & Tendal Citation2001; Dinesen & Bruntse Citation2001; Lindenbaum et al. Citation2008). Petersen (Citation1913) first noticed that M. modiolus aggregations harboured a rich epifauna. Subsequently, biogenic habitats formed by M. modiolus have been recorded widely from northern Atlantic and Pacific waters. The ecological importance of M. modiolus as a habitat for a diverse associated biota has been reported upon from the North Atlantic and adjacent seas (Petersen Citation1918; Spärck Citation1929, Citation1935, Citation1937; Einarsson Citation1941; Thorson Citation1950, Citation1957; Jones Citation1951; Roberts Citation1975; Noble et al. Citation1976; Brown & Seed Citation1977; Warwick & Davies Citation1977; Comely Citation1978; Logan et al. Citation1984; Sebens Citation1985; Witman Citation1985, Citation1987; Davoult et al. Citation1988; Ojeda & Dearborn Citation1989; Brown Citation1990; Magorrian Citation1996; Dinesen Citation1999; Mair et al. Citation2000; Dinesen & Bruntse Citation2001; Moore et al. Citation2006; Lindenbaum et al. Citation2008; Rees et al. Citation2008; Sanderson et al. Citation2008; Rees Citation2009; Roberts et al. Citation2011; Solyanko et al. Citation2011; Ragnarsson & Burgos Citation2012) and Northeast Pacific coastal shelf areas (Shelford Citation1935; Soot-Ryen Citation1955; Selin Citation2011). Modiolus modiolus aggregations include three major community components: (1) a single dense layer of living individuals residing on top of shell debris and supporting a community of sessile epifauna (and epiflora in the photic zone); (2) a mobile megafauna of suspension-feeders, grazers, predators and scavengers; and (3) an either mobile or sedentary macrofauna living in crevices and among the intertwined byssus threads. Where such aggregations occur on sedimentary substrata, an associated infauna often forms a fourth community component. Most of the above studies identified between 100 and 200 macrofaunal taxa associated with M. modiolus, yet overall species numbers may reach ≥ 400 (Göransson & Karlsson Citation1998; Rees et al. Citation2008; Göransson et al. Citation2010) at sites with soft substrates. Similar numbers gave been recorded from an area of extensive Sabellaria reefs (George & Warwick Citation1985). Composition of the associated species complex varies geographically and no obligatory species have been reported to live with M. modiolus. In Strangford Lough, reef restoration by translocating clumps of live M. modiolus increased numbers of recruited spat and associated fauna within the mussel aggregations (Fariñas-Franco et al. Citation2013). Species richness of megafaunal epibenthos has been correlated positively with mussel abundance (Magorrian & Service Citation1998; Ragnarsson & Burgos Citation2012). The biogenic habitat created by M. modiolus thus enhances overall megafaunal diversity in those areas where it occurs.
Mutualistic megafaunal relationships between M. modiolus beds and associated invertebrates were found on exposed rocky coasts in the Gulf of Maine (Witman Citation1985, Citation1987). Mortality from decapod and fish predation on the bivalve Hiatella arctica (Linnaeus, 1767), the brittlestar Ophiopholis aculeata (Linnaeus, 1767) and the sea urchin Strongylocentrotus droebachiensis was higher outside than inside M. modiolus beds at 8 m depth near the Isles of Shoals (Witman Citation1985). There appeared to be a diel difference, where predation at night was by lobsters and crabs, whereas daytime predation was dominated by fish (Witman Citation1985). Grazing S. droebachiensis at depths of between 10 and 12 m resulted in larger changes in the associated fauna outside M. modiolus beds, compared with inside, which supports the theory that this biogenic habitat provides a spatial refuge from predation for associated infaunal invertebrates (Witman Citation1985). Witman (Citation1987) further showed that kelp dominated the upper 8 m depth, its lower distribution being limited by grazing S. droebachiensis. Storms dislodged M. modiolus with attached kelp, but only the latter recolonized faster than the disturbance rate (Witman Citation1987). Modiolus modiolus dominated intermediate depths of 11–18 m, where the sea urchins’ grazing of kelp growing on the shells reduced the risk of mussel dislodgment (Witman Citation1987). A similar facultative mutualism may exist between M. modiolus and other associated fauna.
Relationships between M. modiolus and its rich sessile epifauna are little understood. The filter-feeding Capulus ungaricus (Linnaeus, 1758) lives sedentarily on serpulids, gastropods and bivalves including M. modiolus, where it scavenges particles collected by its host (Jones Citation1948; Orton Citation1948; Sharman Citation1956). Pyramidellids, such as Odostomia eulimoides Hanley, 1844, O. unidentata (Montagu, 1803) and O. turrita Hanley, 1844, occur on M. modiolus shells in Swedish waters as well as on conspecifics in Faroese waters (Schander Citation1995) where they may feed on the body fluids of both serpulids and mytilids (Christoffer Schander, personal communication 1995). Bivalve pectinids are among the larger filter-feeders that often occur on top of deeper M. modiolus aggregations, where they may find shelter and, possibly, benefit from the feeding currents of the hosts. A sea cucumber, Cucumaria frondosa (Gunnerus, 1767), is another large suspension-feeder found as epifauna on M. modiolus, up to six individuals occurring on a single mussel in Faroese waters (Dinesen Citation1996), and possibly benefiting from the feeding currents of and at the same time providing predator protection for their host.
Perturbation impacts
Biogenic habitats formed by Modiolus modiolus have been impacted adversely, sometimes critically, by fishery activities using bottom-towed gear. Artisanal fisheries have targeted M. modiolus as bait for the long-ling fishery (Jeffreys Citation1863; Wiborg Citation1946) and, more locally, for human consumption around the British Isles (Jeffreys Citation1863; Holt et al. Citation1998) and the Faroe Islands (Dinesen & Ockelmann Citation2005).
Scallop dredging targeting Pecten maximus (Linnaeus, 1758) and Aequipecten opercularis (Linnaeus, 1758) is thought to have deteriorated M. modiolus aggregations, removing larger epifaunal elements, including the base individuals, in Strangford Lough in the Irish Sea (Brown Citation1989; Magorrian Citation1996; Service & Magorrian Citation1997; Magorrian & Service Citation1998; Roberts et al. Citation2004, Citation2011; Rees Citation2009; Strain et al. Citation2012) and off the Isle of Man (Kaiser et al. Citation1996; Veale et al. Citation2000; Bradshaw et al. Citation2002; Lambert et al. Citation2011). A survey of scallop dredging impacts targeting Chlamys islandica (O.F. Müller, 1776), conducted between 1993 and 2001 in Breiðafjörður, Iceland, showed that the by-catch of epibenthic megafauna and fish was ∼33% of the scallop catch and one-third of this was M. modiolus (Garcia & Ragnarsson Citation2006). These authors concluded, nevertheless, that a total by-catch of ∼32 tonnes of M. modiolus had no negative impact on the biomass of this species in the two exploited areas during the survey period. It was further not considered that there was a scarcity of M. modiolus in a third recently unexploited area, which had been subject to an intensive scallop fishery between 1972 and 1990. A recent impact study in the Irish Sea by Cook et al. (Citation2013) conversely showed that a single pass of bottom-towed gear significantly reduces epifauna abundances by 59% (scallop dredging) and 90% (trawling, probably by an otter trawl). In the Gulf of Maine, in the Northwest Atlantic, bottom fisheries for lobsters and scallops appear to have impacted negatively the epibenthic megafauna, including M. modiolus (Auster et al. Citation1996; Collie et al. Citation1997, Citation2000). Similarly, in Nova Scotia, Canada, bottom trawling has been demonstrated to change the feeding patterns of several demersal fish species, resulting in increased predation pressures on M. modiolus (Kenchington et al. Citation2005).
An index of sensitivity evaluated M. modiolus as ‘partly fragile and slowly recovering’ from bottom-towed fishing gear (MacDonald et al. Citation1996). Recovery times for perturbated M. modiolus aggregations following more intensive fisheries at larger temporal and spatial scales are, however, largely unassessed. Wiborg (Citation1946) reported a recovery time of between 12 and 20 years following dredging for long-line bait in Norwegian fjords. On Georges Bank in the Northwestern Atlantic, M. modiolus larvae recruited onto test panels within two years (Collie et al. Citation2009), although, due to slow growth (and recruitment) of the species it will take 10–15 years for clusters of large individuals to form. At Knæhagen in western Swedish waters, the M. modiolus reef appears to have diminished in recent years (Göransson et al. Citation2010), in spite of a fisheries ban implemented in the 1930s. In the North Sea, M. modiolus populations declined between the 1900s and 2000, this being linked to bottom dredging and trawling targeting demersal shellfish and finfish species for human consumption (Callaway et al. Citation2007). The North Sea fauna has, however, been subject to other changes, such as alterations in trophic structure and increasing sea temperatures, which appears to have favoured crustacean and echinoderm larvae in the plankton, displacing those of molluscs (Kirby et al. Citation2008). We conclude that this could have negatively affected larval survival and recruitment of boreal bivalve species such as M. modiolus. The effect of altered trophic structure, including predation pressure, as well as increased sea temperatures on M. modiolus larval metabolism and altered diet composition on larval and post-metamorphic survival have not been investigated. It may, however, occur that well-fed larvae may survive post-metamorphic starvation (Ockelmann & Dinesen, personal observations), which may be an adaptation in the autumn-settling larvae of boreal species anticipating food shortages during the winter period.
Fishing activities using bottom-towed gear could have diminished M. modiolus populations by: (1) depleting populations and thereby negatively affecting recruitment patterns within mega-populations and (2) altering ecosystem trophic structure and thereby increasing the numbers of scavengers and, thus, predators of larvae and adults. Coincidentally, increasing seawater temperature could have aggravated such changes by reducing larval and adult fitness and survival, and favouring predators. Such cumulative effects could explain the failing recruitment and maintenance of once thriving M. modiolus populations.
However, it is difficult to determine long-term causal effects of fishing activities on biogenic habitats, such as M. modiolus aggregations, and distinguish these from other pressures. Heavy metal pollution and increasing seawater temperature effects have not been investigated for M. modiolus larvae. After fishery cessations, recovery times may, for example, be prolonged by other cumulative pressures. Increasing seawater temperatures may alter trophic structures and thereby increase predation pressures and decrease food availability for planktotrophic larvae. Larval malnutrition could result in the poorer survival of recruiting spat in boreal species, such as M. modiolus. Rising sea temperatures and fisheries effects combined thus could enhance survival of predators and scavengers feeding on M. modiolus larvae, juveniles, and possibly adults.
Conclusions
The established life-history trait of Modiolus modiolus leaves it vulnerable to anthropogenic disturbances. This marine, habitat-forming species is typified by: (1) endo- and epi-byssal attachment to various seabed substrata where it forms aggregations; (2) large (≤ 210 mm shell length), long-lived (≥ 45 years) adult individuals, which are slow to reproduce with fecundity increasing exponentially with shell growth; (3) spawning frequencies and periodicities varying considerably at spatial and temporal scales and correlated with accumulated temperature–hours (possibly a proxy for food and temperature); (4) planktotrophic larval development, which lasts for ∼4 weeks in boreal waters; (5) recruitment and maintenance of aggregations by both males and females, ensured via the preferential settling of competent larvae in response to adult exhalant water flows, pheromonal for females and, possibly, males; and (6) variability in prevailing hydrographic conditions that determine advection of larvae between local populations, most of which are probably interconnected and some highly important as larval sources for the maintenance of other, sink, populations, although a few are probably self-recruiting.
Deterioration of M. modiolus populations has been related to bottom dredging for this species and different scallop species and to bottom trawling for other shellfish and demersal finfish. The magnitude of the impacts include: (1) aggregation scattering; (2) direct mortality from physical sea bed damage; (3) by-catch discards; and (4) increased exposure to scavengers and otherwise alien predators. Modiolus modiolus bed recovery times have been estimated to be 12–20 years from hand-dredging activities, which is considered to be a lightly impacting fishery. Due to its life-history trait, longer recovery times are anticipated for M. modiolus habitats in more intensively fished areas of overall greater extent and impacts.
The protection, including conservation, of deteriorating M. modiolus populations could be facilitated by rearing larvae and juveniles for the replenishment of wild juvenile stocks among existing aggregations of adults in new, single-layered clusters. Such stock enhancement would be particularly valuable in localities identified to harbour potential natural source populations of this biogenic habitat-forming bivalve.
Editorial responsibility: Christiane Todt
Acknowledgements
We wish to thank Professor R.S.S. Wu, School of Biological Sciences, The University of Hong Kong, for arranging the sectioning of the juvenile Modiolus modiolus reported upon herein and Dr John D. Taylor and Dr Emily A. Glover (The Natural History Museum, London) for providing the juvenile specimens obtained during an international workshop in 2010 organized and funded by The Swedish Species Information Centre under the Swedish Taxonomy Initiative. Also acknowledged is the late Professor Liu Ruiyu (Institute of Oceanology, Academia Sinica, Qingdao, China) for providing specimens of M. modiolus from the Yellow Sea, China. The Zoological Museum of the National History Museum of Denmark (SNM-ZMUC), University of Copenhagen, Denmark, is acknowledged for allowing us to examine specimens of Bathymodiolus thermophilus. We wish to thank Associated Professor Emeritus Kurt W. Ockelmann (Elsinore, Denmark), and Dr Ole S. Tendal and Dr Kathe R. Jensen (SNM-ZMUC) for stimulating discussions on bivalve biology and ecology. We also thank Ole S. Tendal for critical comments on the first draft of this manuscript and two anonymous referees for their constructive inputs. We finally acknowledge the late Dr Jørgen Knudsen for sharing his knowledge of bivalve systematics.
References
- Abbott RT. 1974. American Seashells. The Marine Mollusca of the Atlantic and Pacific Coasts of North America. New York: Van Nostrand Reinhold. 663 pages.
- Abelson A, Weihs D, Loya Y. 1994. Hydrodynamic impediments to settlement of marine propagules, and adhesive filament solutions. Limnology and Oceanography 39:164–69. 10.4319/lo.1994.39.1.0164
- Anwar NA, Richardson CA, Seed R. 1990. Age determination, growth rate and population structure of the horse mussel Modiolus modiolus. Journal of the Marine Biological Association of the United Kingdom 70:441–57.
- Atkins D. 1937. On the ciliary mechanisms and interrelationships of lamellibranchs. Part III. Types of lamellibranch gills and their food currents. Quarterly Journal of Microscopical Science 79:375–421.
- Auster PJ, Malatesta RJ, Langton RW, Watling L, Valentine PC, Donaldson CLS, et al. 1996. The impacts of mobile fishing gear on seafloor habitats in the Gulf of Maine (Northwest Atlantic): Implications for conservation of fish populations. Reviews in Fisheries Science 4:185–202. 10.1080/10641269609388584
- Bakhmet IN, Komendantov AJ, Smurov AO. 2012. Effect of salinity change on cardiac activity in Hiatella arctica and Modiolus modiolus, in the White Sea. Polar Biology 35:143–48. 10.1007/s00300-011-1033-y
- Bayne BL. 1964. Primary and secondary settlement in Mytilus edulis L. (Mollusca). Journal of Animal Ecology 33:513–23. 10.2307/2569
- Beedham GE. 1958. Observations on the non-calcareous component of the shell of the Lamellibranchia. Quarterly Journal of Microscopical Science 51:341–57.
- Bernard F. 1898. Studies of shell ontogeny and morphology of lamellibranchs. Annales des Sciences Naturelles (Zoologie), Série 8, 8:1–208. (in French)
- Bernard FR, Cai YY, Morton B. 1993. Catalogue of the Living Marine Bivalve Molluscs of China. Hong Kong: Hong Kong University Press. 146 pages.
- Board P. 1983. The settlement of post larval Mytilus edulis. Journal of Molluscan Studies 49:53–60.
- Bottjer DJ, Carter JG. 1980. Functional and phylogenetic significance of projecting periostracal structures in the Bivalvia (Mollusca). Journal of Paleontology 54:200–16.
- Bouchet P, Rocroi J-P, Bieler R, Carter JG, Coan EV. 2010. Nomenclature of bivalve families with a classification of bivalve families. Malacologia 52:1–184. 10.4002/040.052.0201
- Bradshaw C, Veale LO, Brand AR. 2002. The role of scallop-dredge disturbance in long-term changes in Irish Sea benthic communities: A re-analysis of an historical dataset. Journal of Sea Research 47:161–84. 10.1016/S1385-1101(02)00096-5
- Brown CH. 1952. Some structural proteins of Mytilus edulis. Quarterly Journal of Microscopical Science 93:487–502.
- Brown RA. 1984. Geographical variations in the reproduction of the horse mussel, Modiolus modiolus (Mollusca: Bivalvia). Journal of the Marine Biological Association of the United Kingdom 64:751–70. 10.1017/S0025315400047214
- Brown RA. 1989. Bottom trawling in Strangford Lough: Problems and policies. In: ten Hallers-Tjabbes CC, Auke B, editors. Distress Signals: Signals from the Environment in Policy and Decision-Making. Proceedings of the 3rd North Sea Seminar, Rotterdam. Amsterdam: Werkgroep Noordzee, p 117–27.
- Brown R. 1990. How the lough was made (Chapter 2) and Life below the waves (Chapter 4). In: Brown R, editor. Strangford Lough, the Wildlife of an Irish Sea Lough. Belfast: The Institute of Irish Studies, The Queen's University of Belfast, p 7–31 and 53–81.
- Brown RA, Seed R. 1977. Modiolus modiolus (L). – An autecological study. In: Keegan BF, Céidigh PÓ, Boaden PJS, editors. Biology of Benthic Organisms. Proceedings of the 11th European Marine Biology Symposium, Galway, Ireland, 1976. Oxford: Pergamon Press, p 93–100.
- Brown RA, Seed R, O'Connor RJ. 1976. A comparison of relative growth in Cerastoderma (=Cardium) edule, Modiolus modiolus, and Mytilus edulis (Mollusca: Bivalvia). Journal of Zoology 179:297–315. 10.1111/j.1469-7998.1976.tb02298.x
- Bruntse G, Tendal OS, editors. 2001. Marine Biological Investigstions and Assemblages of Benthic Invertebrates from the Faroe Islands. Kaldbak, Faroe Islands: Kaldbak Marine Biological Laboratory. 80 pages.
- Callaway R, Engelhard GH, Dann J, Cotter J, Rumohr H. 2007. A century of North Sea epibenthos and trawling: Comparison between 1902–1912, 1982–1985 and 2000. Marine Ecology Progress Series 346:27–43. 10.3354/meps07038
- Carrington E, Moeser GM, Thompson SB, Coutts LC, Craig CA. 2008. Mussel attachment on rocky shores: The effect of flow on byssus production. Integrative and Comparative Biology 48:801–07.
- Carter JG. 1990a. Evolutionary significance of shell microstructure in the Palaeotaxodonta, Pteriomorphia, and Isofilibranchia (Bivalvia: Mollusca). In: Carter J, editor. Skeletal Biomineralization: Patterns, Processes and Evolutionary Trends, volume 1. New York: Van Nostrand Reinhold, p 135–296.
- Carter JG. 1990b. Shell microstructural data for the Bivalvia. In: Carter J, editor. Skeletal Biomineralization: Patterns, Processes and Evolutionary Trends, volume 1. New York: Van Nostrand Reinhold, p 297–411.
- Carter JG, Altaba CR, Anderson LC, Araujo R, Biakov AS, Bogan EA, et al. ( 45 additional authors). 2011. Paleontological Contributions, 4. A Synoptical Classification of the Bivalvia (Mollusca). Lawrence, KS: Paleontological Institute, The University of Kansas. 47 pages.
- Carter JG, Harries PJ, Malchus N, Sartori AF, Anderson LC, Bieler R, et al. ( 15 additional authors). 2012. Treatise Online 48. Part N, Revised, Volume 1, Chapter 31. Illustrated Glossary of the Bivalvia. Lawrence, KS: Paleontological Institute, The University of Kansas. 209 pages.
- Cheung SG, Lam S, Gao F, Mak KK, Shin PKS. 2004. Induced anti-predator responses of the green mussel, Perna viridis (L.), on exposure to the predatory gastropod, Thais clavigera Küster, and the swimming crab, Thalamita danae Stimpson. Marine Biology 144:675–84. 10.1007/s00227-003-1233-2
- Chichvarkin AY. 2002. Letter to the Editor. Re: Phylogeny and Taxonomy of Marine Mussels: Comments on the paper by Distel (2000). Molecular Phylogenetics and Evolution 22:330–32. 10.1006/mpev.2001.1066
- Coan EV, Scott PV, Bernard FR. 2000. Bivalve Seashells of Western North America. Marine Bivalve Mollusks from Arctic Alaska to Baja California. Santa Barbara, CA: Santa Barbara Museum of Natural History Monographs 2. 764 pages.
- Coleman N. 1973. Water loss from aerially exposed mussels. Journal of Experimental Marine Biology and Ecology 12:145–55. 10.1016/0022-0981(73)90010-5
- Coleman N, Trueman ER. 1971. The effect of aerial exposure on the activity of the mussels Mytilus edulis L. and Modiolus modiolus (L.). Journal of Experimental Marine Biology and Ecology 7:295–304. 10.1016/0022-0981(71)90011-6
- Collie JS, Escanero GA, Valentine PC. 1997. Effects of bottom fishing on the benthic megafauna of Georges Bank. Marine Ecology Progress Series 155:159–72. 10.3354/meps155159
- Collie JS, Escanero GA, Valentine PC. 2000. Photographic evaluation of the impacts of bottom fishing on benthic epifauna. Journal of Marine Science 57:987–1001.
- Collie JS, Hermsen JM, Valentine PC. 2009. Recolonization of gravel habitats on Georges Bank (northwest Atlantic). Deep-Sea Research II 56:1847–55. 10.1016/j.dsr2.2009.05.025
- Comely CA. 1978. Modiolus modiolus (L.) from the Scottish west coast. I. Biology. Ophelia 17:167–93. 10.1080/00785326.1978.10425481
- Comely CA. 1981. The physical and biochemical condition of Modiolus modiolus (L.) in selected Shetland voes. Proceedings of the Royal Society of Edinburgh, Series B 80:299–321.
- Connor DW, Allen JH, Golding N, Howell KL, Lieberknecht LM, Northen KO, et al. 2004. The Marine Habitat Classification for Britain and Ireland, v.04.05. Peterborough: Joint Nature Conservation Council. 49 pages. http://jncc.defra.gov.uk/MarineHabitatClassification (accessed 1 May 2013).
- Cook R, Fariñas-Franco JM, Gell FR, Holt RHF, Holt T, Lindenbaum C, et al. 2013. The substantial first impact of bottom fishing on rare biodiversity hotspots: A dilemma for evidence-based conservation. PLOS ONE 8(8): e69904. 10 pages. 10.1371/journal.pone.0069904
- Davenport J, Kjørsvik E. 1982. Observations on a Norwegian intertidal population of the horse mussel Modiolus modiolus (L.). Journal of Molluscan Studies 48:370–71.
- Davoult D, Dewarumez J-M, Prygiel J, Richard A. 1988. Benthic Communities Map of the French Part of the North Sea. IFREMER, Station Marine de Wimereux: Université des Sciences et Techniques de Lille Flandres Artois. 30 pages + 1 map.
- de Schweinitz E, Lutz RA. 1976. Larval development of the northern horse mussel, Modiolus modiolus (L.), including a comparison with the larvae of Mytilus edulis as an aid in plankton identification. Biological Bulletin 150:348–60. 10.2307/1540677
- Dinesen GE. 1996. The Horse Mussel Modiolus modiolus (Linnaeus, 1758) and Associated Fauna from the Faroe Islands. MSc Thesis. Copenhagen: University of Copenhagen, Zoological Museum. 198 pages. ( in Danish)
- Dinesen GE. 1999. Modiolus modiolus and the associated fauna. In: Bruntse G, Lein TE, Nielsen R, editors. Marine Benthic Algae and Invertebrate Communities from the Shallow Waters of the Faroe Islands. A Baseline Study. Kalbak and Thorshavn, Faroe Islands: Kalbak Marine Biological Laboratory and The National History Museum, p 66–71.
- Dinesen GE, Bruntse G. 2001. Modiolus modiolus beds. In: Bruntse G, Tendal OS, editors. Marine Biological Investigation and Assemblages of Benthic Invertebrates from the Faroe Islands. Kalbak, Faroe Islands: Kalbak Marine Biological Laboratory, p 33–36.
- Dinesen GE, Ockelmann KW. 2005. Spatial distribution and species distinction of Modiolus modiolus and syntopic Mytilidae (Bivalvia) in Faroese waters (NE Atlantic). BIOFAR Proceedings 2005, Annales Societas Scientarium Færoenses (Fróðskapparit) Suppl. 41:125–36.
- Distel DL. 2000. Phylogenetic relationships among Mytilidae (Bivalvia): 18S rRNA data suggest convergence in mytilid body plans. Molecular Phylogenetics and Evolution 15:25–33. 10.1006/mpev.1999.0733
- Dixon DR, Solecava AM, Pascoe PL, Holland PWH. 1995. Periostracal adventitious hairs on spat of the mussel Mytilus edulis. Journal of the Marine Biological Association of the United Kingdom 75:363–72. 10.1017/S0025315400018233
- Dodge H. 1952. A historical review of the mollusks of Linnaeus. Part 1. The Classes Loricata and Pelecypoda. Bulletin of the American Museum of Natural History 100:1–263.
- Earll R. 1976. The activity patterns of sublittoral bivalves. Journal of Molluscan Studies 42:454–55.
- Einarsson H. 1941. Survey of the benthonic anima communities of Faxa Bay (Iceland). Meddelelser fra Kommissionen for Danmarks Fiskeri- og Havundersøgelser, Serie Fiskeri 11:1–46.
- Elner RW, Campbell A. 1987. Natural diets of lobster Homarus americanus from barren ground and macroalgal habitats off southwestern Nova Scotia, Canada. Marine Ecology Progress Series 37:131–40. 10.3354/meps037131
- Elsässer B, Fariñas-Franco JM, Wilson CD, Kregting L, Roberts D. 2013. Identifying optimal sites for natural recovery and restoration of impacted biogenic habitat in a special area of conservation using hydrodynamic and habitat suitability modelling. Journal of Sea Research 77:11–21. 10.1016/j.seares.2012.12.006
- Fariñas-Franco JM, Allcock L., Smyth D, Roberts D. 2013. Community convergence and recruitment of keystone species as performance indicators of artificial reefs. Journal of Sea Research 78:59–74. 10.1016/j.seares.2012.10.008
- Fenchel T, Ockelmann KW. 2002. Larva on a string. Ophelia 56:171–78. 10.1080/00785236.2002.10409497
- Flyachinskaya LP, Naumov AD. 2003. Distribution and larval development in the horse mussel Modiolus modiolus (Linnaeus, 1758) (Bivalvia, Mytilidae) from the White Sea. Proceedings of the Zoological Institute, Russian Academy of Science 299:39–50. 10.1080/00785236.2002.10409497
- Fuller SC, Lutz RA. 1989. Shell morphology of larval and post-larval mytilids from the north-western Atlantic. Journal of the Marine Biological Association of the United Kingdom 69:181–218. 10.1017/S0025315400049183
- Gainey LF. 1994. Volume regulation in three species of marine mussels. Journal of Experimental Marine Biology and Ecology 181:201–11. 10.1016/0022-0981(94)90128-7
- Garcia EG, Ragnarsson SA. 2006. Impact of scallop dredging on the macrobenthic community in Breiðafjörður, West Iceland. In: Garcia EG, Ragnarsson SA, Steingrímsson SA, Nævestad D, Haralsson Hþ, Fosså JH, et al., editors. Bottom Trawling and Scallop Dredging in the Arctic. Copenhagen: Nordic Council of Ministers, TemaNord 2006:529, p 129–44.
- Gardiner A. 1927. The parasites of Modiolus modiolus (Linné). Journal of Conchology 18:143.
- Genio L, Kiel S, Cunha MR, Grahame J, Little CTS. 2012. Shell microstructure of mussels (Bivalvia: Mytilidae: Bathymodiolinae) from deep-sea chemosynthetic sites: Do they have a phylogenetic significance? Deep-Sea Research I 64:86–103. 10.1016/j.dsr.2012.02.002
- George CL, Warwick RM. 1985. Annual macrofauna production in a hard-bottom reef community. Journal of the Marine Biological Association of the United Kingdom 65:713–35. 10.1017/S0025315400052553
- Gillmor RB. 1982. Assessment of intertidal growth and capacity adaptations in suspension-feeding bivalves. Marine Biology 68:277–86. 10.1007/BF00409594
- Göransson P, Karlsson M. 1998. Knähagen Reef – Pride of the Öresund. A 100 Year Perspective of Biological Diversity in a Marine Coastal Area. Helsingborg: Report to the Malmöhus County Board and the Environmental Agency of Helsingborg City. 58 pages. ( in Swedish)
- Göransson P, Vuksan SB, Karlfelt J, Börjesson L. 2010. The Haploops Community and the Modiolus Community off Helsingborg 2002–2009. Helsingborg: The Environmental Agency of Helsingborg City. 79 pages. ( in Swedish)
- Graham A. 1949. The molluscan stomach. Transactions of the Royal Society of Edinburgh 61:737–78. 10.1017/S008045680001913X
- Gray JE. 1847. A list of the genera of Recent Mollusca, their synonyma and types. Proceedings of the Zoological Society of London 15:129–219.
- Halanych KM, Vodoti ET, Sundberg P, Dahlgren TG. 2013. Phylogeography of the horse mussel Modiolus modiolus. Journal of the Marine Biological Association of the United Kingdom 93:1857–69. 10.1017/S0025315413000404
- Hansson HG. 1998. Marine Invertebrates of Southern Scandinavia, 2nd edition. Göteborg: The County Board of Västra Götaland 1998:4. 294 pages. ( in Swedish)
- Harper E, Skelton PW. 1993. A defensive value of the thickened periostracum in the Mytiloidea. The Veliger 36:36–42.
- Healy JM, Keyes JL, Daddow LYM. 2000. Comparative sperm ultrastructure in pteriomorphian bivalves with special reference to phylogenetic and taxonomic implications. In: Harper EM, Taylor JD, Crame JA, editors. The Evolutionary Biology of the Bivalvia, Special Publications 177. London: The Geological Society, p 169–90.
- Higo S, Callomon P, Goto Y. 1999. Catalogue and Bibliography of the Marine Shell-Bearing Mollusca of Japan. Osaka: Elle Scientific Publications. 749 pages.
- Hiscock K, Southward A, Tittley I, Hawkins S. 2004. Effects of changing temperature on benthic marine life in Britain and Ireland. Aquatic Conservation: Marine and Freshwater Ecosystems 14:333–62. 10.1002/aqc.628
- Holt TJ, Rees EIS, Hawkins SJ, Seed R. 1998. Biogenic reefs. An overview of dynamic and sensitivity characteristics for conservation management of marine SACs. United Kingdom Marine SACs Project for SNH, DOE(NI), CCW, EN, JNCC and SAMS, IX. 170 pages. http://www.ukmarinesac.org.uk/pdfs/biogreef.pdf (accessed 1 May 2013)
- Huber M. 2010. Compendium of Bivalves. Hackenheim: ConchBooks. 901 pages.
- Jamieson GS, Stone H, Etter M. 1982. Predation of sea scallops (Placopecten megallanicus) by lobsters (Homarus americanus) and rock crabs (Cancer irroratus) in underwater cage enclosures. Canadian Journal of Fisheries and Aquatic Sciences 39:499–505. 10.1139/f82-068
- Jasim AK, Brand AR. 1989. Observations on the reproduction of Modiolus modiolus in Isle of Man waters. Journal of the Marine Biological Association of the United Kingdom 69:373–85. 10.1017/S0025315400029489
- Jeffreys JG. 1863 [ recte 1864]. Marine Shells. British Conchology, or an Account of the Mollusca Which Now Inhabit the British Isles and the Surrounding Seas. Vol. 2. Marine Shells, Comprising the Brachiopoda, and Conchifera from the Family Anomiidae to that of the Mactridae. London: John van Voorst. 566 pages.
- Jensen AS. 1912. Lamellibranchiata (Part I). The Danish Ingolf-Expedition 2:1–119.
- Jensen M. 1966. The response of two sea-urchins to the sea-star Marthasterias glacialis (L.) and other stimuli. Ophelia 3:209–19. 10.1080/00785326.1966.10409643
- Jensenius Madsen F. 1949. Marine Bivalvia. The Zoology of Iceland, volume 4, part 63. Copenhagen and Reykjavík: Ejnar Munksgaard. 116 pages.
- Jones NS. 1948. Biological notes on Capulus ungaricus. Annual Report of the Marine Biological Station at Port Erin, Isle of Man 61:29.
- Jones NS. 1951. The bottom fauna off the south of the Isle of Man. Journal of Animal Ecology 20:132–44. 10.2307/1651
- Jukes-Brown AJ. 1905. A review of the genera of the family Mytilidae. Proceedings of the Malacological Society of London 6:211–24.
- Kabat AR. 1990. Predatory ecology of naticid gastropods with a review of shell boring predation. Malacologia 32:155–93.
- Kafanov AI, Drozdov AL. 1998. Comparative sperm morphology and phylogenetic classification of recent Mytiloidea (Bivalvia). Malacologia 39:129–39.
- Kaiser MJ, Hill AS, Ramsay K, Spencer BE, Brand AB, Veale LO, et al. 1996. Benthic disturbance by fishing gear in the Irish Sea: a comparison of beam trawling and scallop dredging. Aquatic Conservation: Marine and Freshwater Ecosystems 6:269–85. 10.1002/(SICI)1099-0755(199612)6:4%3C269::AID-AQC202%3E3.0.CO;2-C
- Kanwisher JW. 1955. Freezing in intertidal animals. Biological Bulletin 109:56–63. 10.2307/1538658
- Kanwisher JW. 1959. Histology and metabolism of frozen intertidal animals. Biological Bulletin 116:258–64. 10.2307/1539211
- Kenchington EL, Gordon DC, Bourbonnais-Boyce C, MacIsaac KG, Gilkinson KD, McKeown DL, et al. 2005. Effects of experimental otter trawling on the feeding of demersal fish on Western Bank, Nova Scotia. American Fisheries Society Symposium 41:391–409.
- Kenk VC, Wilson BR. 1985. A new mussel (Bivalvia: Mytilidae) from hydrothermal vents in the Galapagos Rift zone. Malacologia 26:253–71.
- Kirby RR, Beaugrand G, Lindley JA. 2008. Climate-induced effects on the meroplankton and the benthic-pelagic ecology of the North Sea. Limnology and Oceanography 53:1805–15. 10.4319/lo.2008.53.5.1805
- Lambert GI, Jennings S, Kaiser MJ, Hinz H, Hiddink JG. 2011. Quantification and prediction of the impact of fishing on epifaunal communities. Marine Ecology Progress Series 430:71–86. 10.3354/meps09112
- Lande E, Gulliksen B, Bræk R. 1973. Growth of horse mussels (Modiolus modiolus (L.)) in the Borgen Fjord. In: Strømgren T, Gulliksen B, editors. The Borgen Fjord Investigations, Preliminary Report 1971–72. Trondheim: Det Kgl. Norske Videnskabers Selskab – Museet, p 4–9. ( in Norwegian)
- Lange R. 1970. Isosmotic intracellular regulation and euryhalinity in marine bivalves. Journal of Experimental Marine Biology and Ecology 5:170–79. 10.1016/0022-0981(70)90015-8
- Lesser MP, Kruse VA. 2004. Seasonal temperature compensation in the horse mussel, Modiolus modiolus: Metabolic enzymes, oxidative stress and heat shock proteins. Comparative Biochemistry and Physiology A, 137:495–504. 10.1016/j.cbpb.2003.10.022
- Lesser MP, Witman JD, Sebens KP. 1994. Effects of flow and seston availability on scope for growth of benthic suspension-feeding invertebrates from the Gulf of Maine. Biological Bulletin 187:319–35. 10.2307/1542289
- Lilleskare J. 1905. Deep water distribution of bivalve fishing bait. Norsk Fiskeritidende 24:50–53. ( in Norwegian)
- Lindenbaum C, Bennell JD, Rees EIS, McClean D, Cook W, Wheeler AJ, et al. 2008. Small-scale variation within a Modiolus modiolus (Mollusca: Bivalvia) reef in the Irish Sea: I. Seabed mapping and reef morphology. Journal of the Marine Biological Association of the United Kingdom 88:133–41. 10.1017/S0025315408000374
- List T. 1902. Fauna und Flora des Golfes von Neapel und der angrenzenden Meeres-Abschnitte, I. I. Die Mytiliden. Mittheilungen aus der Zoologischen Station zu Neapel 27:1–312.
- Logan A, Page FH, Thomas MLH. 1984. Depth zonation of epibenthos on sublittoral hard substrates off Deer Island, Bay of Fundy, Canada. Estuarine, Coastal and Shelf Science 18:571–92. 10.1016/0272-7714(84)90091-X
- Lorion J, Duperron S, Gros O, Cruaud C, Samadi S. 2009. Several deep-sea mussels and their associated symbionts are able to live both on wood and whale falls. Proceedings of the Royal Society of London, Series B 276:177–85. 10.1098/rspb.2008.1101
- Lorion J, Buge B, Cruaud C, Samadi S. 2010. New insights into diversity and evolution of deep-sea Mytilidae (Mollusca: Bivalvia). Molecular Phylogenetics and Evolution 57:71–83. 10.1016/j.ympev.2010.05.027
- Lutz RA, Hidu H. 1979. Hinge morphogenesis in the shells of larval and early post-larval mussels (Mytilus edulis L. & Modiolus modiolus (L.)). Journal of the Marine Biological Association of the United Kingdom 59:111–21. 10.1017/S002531540004621X
- Lutz RA, Jablonski D, Rhoads DC, Turner RD. 1980. Larval dispersal of a deep-sea hydrothermal vent bivalve from the Galápagos Rift. Marine Biology 57:127–33. 10.1007/BF00387378
- MacDonald DS, Little M, Eno NC, Hiscock K. 1996. Disturbance of benthic species by fishing activities: A sensitivity index. Aquatic Conservation: Marine and Freshwater Ecosystems 6:257–68. 10.1002/(SICI)1099-0755(199612)6:4%3C257::AID-AQC194%3E3.0.CO;2-7
- Magorrian BH. 1996. The Impact of Commercial Trawling on the Benthos of Strangford Lough. PhD Thesis. Belfast: The Queen's University of Belfast. 218 pages.
- Magorrian BH, Service M. 1998. Analysis of underwater visual data to identify the impact of physical disturbance on horse mussel (Modiolus modiolus) beds. Marine Pollution Bulletin 6:354–59. 10.1016/S0025-326X(97)00192-6
- Mair JM, Moore CG, Kingston PF, Harris DB. 2000. A Review of the Status, Ecology and Conservation of Horse Mussel Modiolus modiolus Beds in Scotland. Edinburgh: Scottish Natural Heritage Commissioned Report No. F99PA08. 89 pages.
- Meadows PS, Shand P. 1989. Experimental analysis of byssus thread production by Mytilus edulis and Modiolus modiolus in sediments. Marine Biology 101:219–26. 10.1007/BF00391461
- M'Intosh WC. 1894. Notes from the St. Andrews Marine Laboratory (under the Fishery Board for Scotland). 5. On a hermaphrodite example of Mytilus modiolus. Annals and Magazine of Natural History ( 6)14(81):196.
- Morton B. 1973. Some aspects of the biology and functional morphology of the organs of feeding and digestion of Limnoperna fortunei (Dunker) (Bivalvia: Mytilacea). Malacologia 12:265–81.
- Morton B. 1977a. The biology and functional morphology of Modiolus metcalfei Hanley 1844 (Bivalvia: Mytilacea) from the Singapore mangrove. Malacologia 16:500–18.
- Morton B. 1977b. An estuarine bivalve (Modiolus striatulus) fouling raw water supply systems in West Bengal, India. Journal of the Institution of Water Engineers and Scientists 31:441–52.
- Morton B. 1992. The evolution and success of the heteromyarian form in the Mytiloida. In: Gosling EM, editor. The Mussel Mytilus: Ecology, Physiology, Genetics and Culture. New York: Elsevier, p 21–52.
- Morton B. 2008. The evolution of eyes in the Bivalvia: New insights. American Malacological Bulletin 26:35–45. 10.4003/006.026.0205
- Morton B. 2011. Predator-prey-scavenging interactions between Nucella lapillus (Linnaeus, 1758), Carcinus maenas and Eulalia viridis all exploiting Mytilus galloprovincialis on a rocky shore recovering from tributyl-tin (TBT) pollution. Journal of Natural History 45:2397–417. 10.1080/00222933.2011.596637
- Moore CG, Saunders GR, Harries DB, Mair JM, Bates CR, Lyndon AR. 2006. The establishment of site condition monitoring of the subtidal reefs of Loch Creran Special Area of Conservation. Edinburgh: Scottish Natural Heritage Commissioned Report No. 151. 119 pages.
- Møhlenberg F, Riisgård HU. 1978. Efficiency of particle retention in 13 species of suspension feeding bivalves. Ophelia 17:239–46. 10.1080/00785326.1978.10425487
- Møhlenberg F, Riisgård HU. 1979. Filtration rate, using a new indirect technique, in thirteen species of suspension-feeding bivalves. Marine Biology 54:143–47. 10.1007/BF00386593
- Navarro JM, Thompson RJ. 1994. Comparison and evaluation of different techniques for measuring absorption efficiency in suspension feeders. Limnology and Oceanography 39:159–64. 10.4319/lo.1994.39.1.0159
- Navarro JM, Thompson RJ. 1996. Physiological energetics of the horse mussel Modiolus modiolus in a cold ocean environment. Marine Ecology Progress Series 138:135–48. 10.3354/meps138135
- Navarro JM, Thompson RJ. 1997. Biodeposition by the horse mussel Modiolus modiolus (Dillwyn) during the spring diatom bloom. Journal of Experimental Marine Biology and Ecology 209:1–13. 10.1016/0022-0981(96)02681-0
- Nelson TC. 1918. On the origin, nature and function of the crystalline style of lamellibranchs. Journal of Morphology 31:53–111. 10.1002/jmor.1050310105
- Nielsen C. 1975. Observations on Buccinum undatum L. attacking bivalves and on prey responses, with a short review on attack methods of other prosobranchs. Ophelia 13:87–108. 10.1080/00785326.1974.10430593
- Noble JPA, Logan A, Webb GR. 1976. The recent Terebratulina community in the rocky subtidal zone of the Bay of Fundy, Canada. Lethaia 9:1–17. 10.1111/j.1502-3931.1976.tb00942.x
- Nordgaard O. 1901. Contribution to the biology of horse mussels. Norsk Fiskeritidende 20:541–51. (in Norwegian)
- Nørrevang A, Brattegard T, Josefson AB, Sneli J-A, Tendal OS. 1994. List of BIOFAR stations. Sarsia 79:165–80.
- Ockelmann KW. 1983. Descriptions of mytilid species and definition of the Dacrydiinae n. subfam. (Mytilacea – Bivalvia). Ophelia 22:81–123. 10.1080/00785326.1983.10427225
- Ockelmann KW. 1995. Ontogenetic characters of mytilaceans. Phuket Marine Biological Center, Special Publication 15:85–88.
- Ockelmann KW, Dinesen GE. 2009. Systematic relationship of the genus Adula and its descent from a Mytilus-like ancestor (Bivalvia, Mytilidae, Mytilinae). Steenstrupia 30:141–52.
- Ockelmann KW, Dinesen GE. 2011. Life on wood – The carnivorous deep-sea mussel Idas argenteus (Bathymodiolinae, Mytilidae, Bivalvia). Marine Biology Research 7:71–84. 10.1080/17451001003714504
- Ojeda FP, Dearborn JH. 1989. Community structure of macroinvertebrates inhabiting the rocky subtidal zone in the Gulf of Maine: Seasonal and bathymetric distribution. Marine Ecology Progress Series 57:147–61. 10.3354/meps057147
- Orton JH. 1948. Note on the feeding habitat of Capulus ungaricus. Annual Report of the Marine Biological Station at Port Erin, Isle of Man 61:29–30.
- OSPAR. 2008. Case reports from the OSPAR List of threatened and/or declining species and habitats. London: OSPAR Commission, Biodiversity Series, p 239–42.
- Pearce T, LaBarbera M. 2009. A comparative study of the mechanical properties of mytilid byssal threads. The Journal of Experimental Biology 212:1442–48. 10.1242/jeb.025544
- Petersen CGJ. 1913. Valuation of the sea II. The animal communities of the sea-bottom and their importance for marine zoogeography. Report from the Danish Biological Station to the Board of Agriculture 21:1–68.
- Petersen CGJ. 1918. The sea bottom and its production of fish food. A survey of work done in connection with the valuation of the Danish waters from 1883–1917. Reports of the Danish Biological Station 25:1–62. ( in Danish)
- Pierce SK. 1970. The water balance of Modiolus (Mollusca: Bivalvia: Mytilidae): Osmotic concentrations in changing salinities. Comparative Biochemistry and Physiology 36:521–33. 10.1016/0010-406X(70)91028-5
- Pujol JP. 1967. Formation of the byssus in the common mussel (Mytilus edulis L.). Nature 214:204–05. 10.1038/214204a0
- Purchon RD. 1957. The stomach in the Filibranchia and Pseudolamellibranchia. Proceedings of the Zoological Society of London 129:27–60. 10.1111/j.1096-3642.1957.tb00279.x
- Ragnarsson SA, Burgos JM. 2012. Separating the effects of a habitat modifier, Modiolus modiolus and substrate properties on the associated megafauna. Journal of Sea Research 72:55–63. 10.1016/j.seares.2012.05.011
- Rees CB. 1950. The identification and classification of lamellibranch larvae. Hull Bulletin of Marine Ecology 3:73–104.
- Rees I. 2009. Assessment of Modiolus modiolus Beds in the OSPAR Area. To the OSPAR Commission, on behalf of Joint Nature Conservation (JNCC), Peterborough. 22 pages.
- Rees EIS, Sanderson WG, Mackie ASY, Holt RHF. 2008. Small-scale variation within a Modiolus modiolus (Molluscs: Bivalvia) reef in the Irish Sea. III. Crevice, sediment infauna and epifauna from targeted cores. Journal of the Marine Biological Association of the United Kingdom 88:151–56. 10.1017/S0025315408000052
- Reid DG. 1990. Trans-Arctic migration and speciation induced by climate change: The biogeography of Littorina (Mollusca: Gastropoda). Bulletin of Marine Science 47:35–49.
- Reid RGB. 1965. The structure and function of the stomach in bivalve molluscs. Journal of Zoology 147:156–84. 10.1111/j.1469-7998.1965.tb04640.x
- Ritt B, Duperron S, Lorion J, Lazar CS, Sarrazin J. 2012. Integrative study of a new cold-seep mussel (Mollusca: Bivalvia) associated with chemosynthetic symbionts in the Marmara Sea. Deep-Sea Research I 67:121–32. 10.1016/j.dsr.2012.05.009
- Roberts CD. 1975. Investigations into a Modiolus modiolus (L.) (Mollusca: Bivalvia) community in Strangford Lough, Northern Ireland. Report from the Underwater Association 1:27–49.
- Roberts CD. 1979. The colonial behaviour of Modiolus modiolus (L.) (Bivalvia) and its ecological significance. In: Larwood G, Rosen BR, editors. Biology and Systematics of Colonial Organisms. Systematics Association, special volume no. 11. London and New York: Academic Press, p 255–56.
- Roberts D, Davies C, Mitchell A, Moore H, Picton B, Portig A, et al. 2004. Strangford Lough Ecological Change Investigation (SLECI). Report to Environment and Heritage Service by the Queen's University, Belfast. 76 pages.
- Roberts D, Allcock L, Fariñas JM, Gorman E, Maggs CA, Mahon AM, et al. 2011. Modiolus Restoration Research Project. Final Report and Recommendations (20th May 2011). Department of Agriculture and Rural Development & Northern Ireland Environment Agency. Belfast: Queen's University. 246 pages. http://www.qub.ac.uk/research-centres/ModiolusRestorationResearchGroup/Documents/Filetoupload,245109,en.pdf (accessed 1 May 2013).
- Rowell TW. 1967. Some Aspects of the Ecology, Growth and Reproduction of the Horse Mussel (Modiolus modiolus). MSc Thesis. Kingston, ON: Queen's University. 136 pages.
- Samadi S, Quemere E, Lorion J, Tillier A, Cosel R, Lopez P, et al. 2007. Molecular phylogeny in mytilids supports the wooden steps to deep-sea vents hypothesis. Comptes Rendus Biologies 330:446–56. 10.1016/j.crvi.2007.04.001
- Sanderson WG, Holt RHF, Kay L, Ramsay K, Perrins J, McMath AJ, et al. 2008. Small-scale variation within a Modiolus modiolus (Mollusca: Bivalvia) reef in the Irish Sea. II. Epifauna recorded by divers and cameras. Journal of the Marine Biological Association of the United Kingdom 88:143–49. 10.1017/S0025315408000040
- Schander C. 1995. Pyramidellidae (Mollusca, Gastropoda, Heterobranchia) of the Faroe Islands. Sarsia 80:55–65.
- Schlieper C, Kowalski R, Erman P. 1958. Contribution to the ecological and cell-physiological characteristics of the boreal lamellibranch Modiolus modiolus L. Kieler Meeresforschungen 14:3–10. (in German)
- Sebens KP. 1985. The ecology of the rocky subtidal zone. American Scientist 73:548–57.
- Seed R. 1976. Ecology. In: Bayne BL, editor. Marine Mussels, their Ecology and Physiology. Cambridge: Cambridge University Press, p 13–66.
- Seed R. 1996. Patterns of biodiversity in the macro-invertebrate fauna associated with mussel patches on rocky shores. Journal of the Marine Biological Association of the United Kingdom 76:203–10. 10.1017/S0025315400029131
- Seed R, Brown RA. 1975. The influence of reproductive cycle, growth, and mortality on population structure in Modiolus modiolus (L.), Cerastoderma edule (L.), and Mytilus edulis L., (Mollusca: Bivalvia). In: Barnes H, editor. Proceedings of the 9th European Marine Biological Symposium. Aberdeen: Aberdeen University Press, p 257–74.
- Seed R, Brown RA. 1977. A comparison of the reproductive cycles of Modiolus modiolus (L.), Cerastoderma (=Cardium) edule (L.), and Mytilus edulis L. in Strangford Lough, Northern Ireland. Oecologia 30:173–88. 10.1007/BF00345419
- Seed R, Brown RA. 1978. Growth as a strategy for survival in two marine bivalves, Cerastoderma edule and Modiolus modiolus. Journal of Animal Ecology 47:283–92. 10.2307/3936
- Seed R, Suchanek TH. 1992. Population and community ecology of Mytilus. In: Gosling E, editor. The Mussel Mytilus: Ecology, Physiology, Genetics and Culture. Amsterdam: Elsevier, p 87–169.
- Seed R, Richardson CA, Smith K. 2000. Marine mussels, their evolutionary success, ecological significance and use as chronometers of environmental change. In: Harper EM, Taylor JD, Crame JA, editors. The Evolutionary Biology of the Bivalvia. Special Publications 177. London: The Geological Society, p 465–78.
- Selin NI. 2011. Use of the photo method in the study of the composition and abundance of soft-bottom macrobenthos. Russian Journal of Marine Biology 37:232–38.
- Service M, Magorrian BH. 1997. The extent and temporal variation of disturbance to epibenthic communities in Strangford Lough, Northern Ireland. Journal of the Marine Biological Association of the United Kingdom 77:1151–64. 10.1017/S0025315400038686
- Sharman M. 1956. Note on Capulus ungaricus (L.). Journal of the Marine Biological Association of the United Kingdom 35:445–50. 10.1017/S0025315400010262
- Shelford VE. 1935. General survey of the communities – Their extent and dynamics. The major communities. Ecological Monographs 5:251–92.
- Silverman HG, Roberto FF. 2007. Understanding marine mussel adhesion. Marine Biotechnology 9:661–81. 10.1007/s10126-007-9053-x
- Solyanko K, Spiridonov V, Naumov A. 2011. Biomass, commonly occurring and dominant species of macrobenthis in Onega Bay (White Sea, Russia): Data from three different decades. Marine Ecology 32(Suppl. 1):36–48. 10.1111/j.1439-0485.2011.00438.x
- Soot-Ryen T. 1955. A report on the family Mytilidae (Pelecypoda). Allan Hancock Pacific Expedition 20:1–175.
- Soot-Ryen T. 1969. Superfamily Mytilacea Rafinesque, 1815. In: Moore RC, editor. Treatise on Invertebrate Paleontology. Part N, Mollusca 6, Bivalvia 1 (of 3). Lawrence, KS: Geological Society of America Inc. and University of Kansas Press, p N271–N280.
- Spärck R. 1929. Preliminary survey of the results of quantitative bottom investigations in Iceland and Faroe waters 1926–1927. Rapports et Procès-Verbaux des Réunions 57:1–28.
- Spärck R. 1935. On the importance of quantitative investigations of the bottom fauna in marine biology. Journal du Conseil Permanent International pour l'Exploration de la Mer 10:1–19.
- Spärck R. 1937. The benthic animal communities of the coastal waters. In: Friðriksson Á, Tuxen SL, editors. The Zoology of Iceland, volume 1, part 6. Copenhagen: Andreas Frederik Høst & Søn. 45 pages.
- Stanley SM. 1970. Relation of shell form to life habits of the Bivalvia (Mollusca). The Geological Society of America Inc. Memoir 125:i–xiii,1–296.
- Stanley SM. 1972. Functional morphology and evolution of byssally attached bivalve molluscs. Journal of Paleontology 46:165–212.
- Stasek CR. 1963. Synopsis and discussion of the association of ctenidia and labial palps in the bivalved Mollusca. The Veliger 6:91–97.
- Strain EMA, Allcock AL, Goodwin CE, Maggs CA, Picton BE, Roberts D. 2012. The long-term impacts of fisheries on epifaunal assemblage function and structure, in a Special Area of Conservation. Journal of Sea Research 67:58–68. 10.1016/j.seares.2011.10.001
- Strand Ø, Vølstad JH. 1997. The molluscan fisheries and culture of Norway. NOAA Technical Report NMFS 129:7–24.
- Strömgren T. 1976. Growth rates of Modiolus modiolus (L.) and Cerastoderma edule (L.) (Bivalvia) during different light conditions. Sarsia 61:41–46.
- Suchanek TH. 1985. Mussels and their role in structuring rocky shore communities. In: Moore PG, Seed R, editors.The Ecology of Rocky Coasts. London: Hodder & Stoughton, p 79–96.
- Taylor JD, Kennedy WJ, Hall A. 1969. The shell structure and mineralogy of the Bivalvia. Introduction. Nuculacae-Trigonacae. Bulletin of the British Museum, Natural History (Zoology) Suppl. 3:1–125.
- Tebble N. 1976. British Bivalve Seashells, a Handbook for Identification, 2nd edition. London: Trustees of the British Museum (Natural History). 212 pages.
- Termier G, Termier H. 1950. Paléontologie Marocaine. 2, Invertébrés de l'Ère Primaire. Fasc. 3, Mollusques. Service Géologique du Maroc, Notes et Mémoires 78. Paris: Hermann. 246 pages, plates 123–183.
- Thiele J. 1934. Handbook of Systematic Malacology, Part III (Scaphopoda, Bivalvia, Cephalopoda). Family Mytilidae. Jena: Gustav Fisher, p 797–801. (in German)
- Thorson G. 1950. Life in the Sea. In: Bræstrup FW, Thorson, G, Wesenberg-Lund, E, editors. Our Country's Animals, Immigration, Animal Communities, Life Habits. Volume III. Copenhagen: Nordisk Forlag, p 35–75. ( in Danish)
- Thorson G. 1957. Bottom communities. Sublittoral or shelf. In: Hedgepeth JW, editor. Treatise on Marine Ecology and Paleoecology. Volume I. Ecology. Washington, DC: The Geological Society of America Memoir 67:461–534.
- Van Dover CL. 2002. Community structure of mussel beds at deep-sea hydrothermal vents. Marine Ecology Progress Series 230:137–58. 10.3354/meps230137
- Van Dover CL, Trask JL. 2000. Diversity at deep-sea hydrothermal vent and intertidal mussel beds. Marine Ecology Progress Series 195:169–78. 10.3354/meps195169
- Veale LO, Hill AS, Hawkins SJ, Brand AR. 2000. Effects of long-term physical disturbance by commercial scallop fishing on subtidal epifaunal assemblages and habitats. Marine Biology 137:325–37. 10.1007/s002270000357
- Vermeij GJ. 1991. Anatomy of an invasion: The trans-Arctic interchange. Paleobiology 17:281–307.
- Vokes HE. 1980. Genera of the Bivalvia: A Systematic and Bibliographic Catalogue (revised and updated), 2nd edition. Ithaca, NY: Paleontological Research Institution. 307 pages.
- Warwick RM, Davies JR. 1977. The distribution of sublittoral macrofauna communities in the Bristol Channel in relation to the substrate. Estuarine and Coastal Marine Science 5:267–88. 10.1016/0302-3524(77)90022-6
- White KM. 1937. Mytilus. Liverpool Marine Biological Committee Memoirs on Typical British Marine Plants and Animals 31:1–117.
- Wiborg KF. 1946. Investigations of the horse mussel (Modiola modiolus (L.)). Reports on the Norwegian Fishery and Marine Investigations 8(5):1–85. (in Norwegian)
- Wildish DJ, Fader GBJ. 1998. Pelagic-benthic coupling in the Bay of Fundy. Hydrobiologia 375/376:369–80. 10.1023/A:1017080116103
- Wildish DJ, Kristmanson DD. 1985. Control of suspension feeding bivalve production by current speed. Helgoländer Meeresuntersuchungen 39:237–43. 10.1007/BF01992771
- Wildish DJ, Kristmanson DD. 1994. Importance of the benthic boundary layer. Canadian Journal of Fisheries and Aquatic Sciences 41:1618–25. 10.1139/f84-200
- Wildish DJ, Peer D. 1983. Tidal current speed and production of benthic macrofauna in the lower Bay of Fundy. Canadian Journal of Fisheries and Aquatic Sciences (Suppl.) 40:309–21. 10.1139/f83-292
- Wildish DJ, Fader GBJ, Lawton P, MacDonald AJ. 1998. The acoustic detection and characterization of sublittoral bivalve reefs in the Bay of Fundy. Continental Shelf Research 18:105–13. 10.1016/S0278-4343(98)80002-2
- Wildish DJ, Fader GBJ, Parrott DR. 2009. A model of horse mussel reef formation in the Bay of Fundy based on population growth and geological processes. Atlantic Geology 45:157–70. 10.4138/atlgeol.2009.007
- Williamson HC. 1906. The spawning, growth, and movement of the mussel (Mytilus edulis, L.), horse mussel (Modiolus modiolus, L.) and the spoutfish (Solen siliqua, L.). 25th Annual Report of the Fishery Board for Scotland 3:221–55.
- Wilson DP. 1977. Modiolus modiolus (L.) in small mid-tidal rock pools at Penrhyn Bay, North Wales. Estuarine and Coastal Marine Science 5:215–22. 10.1016/0302-3524(77)90018-4
- Winter J. 1969. About the influence of food concentration and other factors on filtration performance and food utilisation in the bivalves Arctica islandica and Modiolus modiolus. Marine Biology 4:87–135. (in German) 10.1007/BF00347037
- Winter J. 1970. Filter feeding and food utilization in Arctica islandica L. and Modiolus modiolus at different food concentrations. In: Steele JH, editor. Marine Food Chains. Berkeley, CA: University of Berkeley, p 196–206.
- Witman JD. 1985. Refuges, biological disturbance, and rocky subtidal community structure in New England. Ecological Monographs 55:421–45. 10.2307/2937130
- Witman JD. 1987. Subtidal coexistence: Storms, grazing, mutualism, and the zonation of kelps and mussels. Ecological Monographs 57:167–87. 10.2307/1942623
- Wright MM, Francis L. 1984. Predator deterrence by flexible shell extensions of the horse mussel Modiolus modiolus. The Veliger 27:140–42.
- Yonge CM. 1978. Significance of the ligament in the classification of the Bivalvia. Proceedings of the Royal Society of London, Series B 202:231–48. 10.1098/rspb.1978.0065