ABSTRACT
Norway lobsters, Nephrops norvegicus, are sediment-dwelling decapod crustaceans that excavate burrows from which they make short excursions to feed by predation and scavenging. The females of this species are known to reside within their burrows for an extended period of time over the winter while brooding their eggs. The aim of this study was to assess the likelihood of these females being able to feed during this brooding period. Biophysical and biochemical measurements that had previously been shown to change with starvation under laboratory conditions in male N. norvegicus were taken for female N. norvegicus under similar conditions. These measurements were also compared in both sexes obtained from monthly trawl samples from the Clyde Sea Area, Scotland, UK, together with trawl composition data. The laboratory study showed that the hepatosomatic index, and the copper, lipid and water content of the hepatopancreas can be used as indicators of the state of starvation in females, as in males. In the wild, both sexes have reduced nutritional status during the winter, but not to the degree seen in animals starved for 20 weeks in aquarium trials. This study does not support the hypothesis that females cease feeding over winter, during their brooding period. Firstly, some females were unable to sustain ovary development during starvation under controlled conditions, contrary to field observations. Secondly, field data suggest that there is no sex-specific reduction in nutritional status.
RESPONSIBLE EDITOR:
Introduction
Norway lobsters, Nephrops norvegicus (Linnaeus, 1758), are carnivores that emerge from their burrows to feed. Periods of burrow emergence vary in relation to ambient light conditions, sex and season (Bell et al. Citation2006). The nutritional status of a wild animal depends on a variety of factors, such as abundance and quality of food, search and capture ability, conspecific and interspecific competition, predator avoidance and periodical physiological changes (Macleod et al. Citation2008). Animals have developed coping mechanisms to deal with periods of food shortage, which range from reducing their metabolic rate to varying the extent to which tissue reserves are utilized to obtain energy (Roots Citation2006). Norway lobsters experience a number of these limiting nutritional conditions throughout their life.
Norway lobsters have been shown to be opportunistic predators and scavengers (Thomas & Davidson Citation1962) with stomach contents reflecting prey abundance rather than feeding preference (Bell et al. Citation2006). Fluctuations in primary production will influence the abundance of the benthic organisms on which they feed and may result in a reduction in food availability in the winter (Stephens et al. Citation1967). The nutritional status of a population of N. norvegicus in a particular area is also driven by density-dependent factors. In high density areas competition for food may limit scope for growth. Moreover, increased aggressive social behaviour in high density areas could drive up the metabolic rate and thus energy requirements (Tuck et al. Citation1997b; Chapman & Bailey Citation1987; Parslow-Williams Citation1998; Tuck et al. Citation1999; Bell et al. Citation2006; Campbell et al. Citation2009).
The reproductive cycle of female N. norvegicus shows latitudinal differences in the times of spawning, incubation, egg hatching and mating (Bell et al. Citation2006). Those in the Clyde Sea Area contain both annual and biennial spawners (Bailey Citation1984). Females become reproductively mature at around three years of age (Bell et al. Citation2006). As age is not easy to determine, due to the fact that there are no morphological structures that change in an age-related manner and are retained across successive moults, the size of the female is most often used to determine the stage at which it becomes reproductively active. This is known as the ‘Size at Onset Maturity’ or SOM, which has been defined by Bailey (Citation1984) and Tuck et al. (Citation1997a) as the size at which 50% of females (L50) have ovaries in a reproductively active condition – an indicator of ‘physiological maturity’. As an alternative indicator of SOM, both Bailey (Citation1984) and Tuck et al. (Citation1997a) have also used the size of the smallest ovigerous female as an indicator of ‘functional maturity’ and found no significant difference between these two methods.
Farmer (Citation1974), Rotllant et al. (Citation2005) and Mente et al. (Citation2009) described in detail the development of the ovary maturation cycle. Immature females and those between reproductive events have cream-coloured ovaries. As they develop, the ovaries are coloured by a green vitellogen protein (Avarre et al. Citation2003). Ovary development commences during the winter, only reaching pale green coincident with emergence in spring. Most development takes place as a result of active feeding after spawning, moulting and mating (Farmer Citation1974). They mature through the spring and summer when animals are actively feeding, with egg laying occurring in late summer and autumn, after which females retreat to their burrows (Bell et al. Citation2006).
Nephrops norvegicus need to balance their food-searching behaviour with the need to avoid predators. These trade-offs are resolved by the animals staying in close proximity to their burrows during feeding excursions (Chapman & Rice Citation1971). Many of these limiting factors could be experienced by both sexes of N. norvegicus, but some will particularly affect ovigerous (so-called ‘berried’) females as they are known to reside within their burrows over winter while brooding their eggs, presumably in order to reduce their predation risk (Aguzzi et al. Citation2007). Newland (Citation1985) showed that abdominal tail flipping (the method of escape swimming) is suppressed in egg-bearing N. norvegicus and that any flexions performed involve only the tail fan (uropods and telson) which does not carry eggs. Therefore, there would be a greater predation risk for females in leaving their burrows to feed. Aguzzi et al. (Citation2007) suggested that females can be attracted out of their burrows when food is available, as indicated by catching ovigerous females in creels, but then stay within close proximity to a burrow opening. With this limited foraging range, it is entirely possible that females reduce their feeding rate, or completely cease feeding. Aguzzi et al. (Citation2007) showed that the percentage of ovigerous females with empty stomachs was significantly higher (60%) than that of non-ovigerous females (50%). Also, Oakley (Citation1978) showed that ovigerous females were less likely to react to chemical food stimuli than either males or non-ovigerous females. Female N. norvegicus possibly enter a period of torpor while incubating their eggs in burrows, although there remains a requirement for them to ventilate and clean the eggs (Waddy et al. Citation1995). Such studies hypothesized that ovigerous females have a reduced nutritional state. It has been suggested that suspension feeding may provide an additional food source (Loo et al. Citation1993; Bell et al. Citation2006) but this is unlikely to be of benefit during the winter, though suspended matter derived from phytoplankton blooms may be available in late autumn and early spring, near the beginning and end of the period of burrow residence.
We have recently established that male N. norvegicus can survive for over six months without feeding (Watts et al. Citation2014), and since this is a similar length of time to the burrow residence time for female N. norvegicus, it is entirely possible that they can also survive for this duration without feeding.
Determining biomarkers of starvation that could be used reliably with specimens sampled in the field would help to show whether the females have a reduced nutritional status through the winter. Females are inaccessible for such assessments while they remain within their burrows (they are difficult to sample), but due to their relatively synchronized emergence from burrows post-brooding (Milligan et al. Citation2009), meaningful data can be obtained by targeting newly emerged females.
Watts et al. (Citation2014) identified appropriate measures for establishing the nutritional status of male N. norvegicus when they had not fed for 20 weeks. These were the hepatosomatic index (HSI) and the water, lipid and copper content of the hepatopancreas and the carbon: nitrogen ratio of the abdominal tail muscle.
A reduction of whole body weight due to a reduced nutritional status does not occur within N. norvegicus, since when reserves such as lipids become depleted in the hepatopancreatic tissue there is a corresponding increase in water content (Comoglio et al. Citation2005). In male N. norvegicus lipid content decreased from an initial percentage of 15.22 ± 2.78 to 2.58 ± 1.32 (wet weight), with a corresponding increase of water content from 68.05 ± 2.22% to 75.62 ± 2.39% (Watts et al. Citation2014). This was also seen by Karapanagiotidis et al. (Citation2015) where crude lipids from the hepatopancreas (measured as dry weight) were 50.22 ± 1.28% in well fed N. norvegicus and 13.35 ± 1.19% in N. norvegicus starved over similar time scales as in Watts et al. (Citation2014). As a result, the combined weight of lipid and water held within the hepatopancreas did not significantly vary over time, remaining at approximately 80% of the tissue weight. The hepatopancreas itself, however, has also been shown to decrease in size with starvation. Therefore, HSI (the proportion compared with whole body weight) can be an effective indicator of starvation in male N. norvegicus. Copper content of the hepatopancreas was shown in Watts et al. (Citation2014) to increase with the level of starvation, from an initial 153.53 ± 42.17 μg g−1 to 423.37 ± 158.88 μg g−1 by week 12. This was also noted by Baden et al. (Citation1994) with a drop in copper concentration in the haemolymph being accompanied by an increase in the hepatopancreas. Starvation showed very few effects on the constituents of the tail muscle: its water content did not vary significantly, perhaps due to the fact that it contains only c. 2% lipid. A decrease in lipid content was not directly recorded, although the carbon:nitrogen ratio, which is known to be a proxy indicator of lipid metabolism, did decrease.
The aims of the present study were therefore to establish whether these measures of starvation effects in males were also appropriate for assessing the nutritional status of female N. norvegicus by performing a laboratory fasting experiment and if so, to use such measures on newly emerged field-caught females to determine if they are in a reduced nutritional state (compared with males), having been confined to their burrows through the winter.
Materials and methods
The study comprised an aquarium investigation of the effect of food deprivation on the biophysical dimensions and biochemical reserves of female N. norvegicus over a period of approximately six months. Biophysical and biochemical measurements were taken from field-caught individuals, and then compared with those from the aquarium study to assess the likelihood of food deprivation in the wild and thus the nutritional status for both males and females. Data presented in Watts et al. (Citation2014) were used to compare the nutritional status of males in the field.
Aquarium study: physiological effects of long-term starvation
Nephrops norvegicus were collected (October 2010) by trawling from the Clyde Sea Area (CSA), Scotland, UK along an established transect north of the Isle of Cumbrae (55°51.35′N 4°54.42′W to 55°48.97′N 4°54.05′W) (Beevers et al. Citation2012; Watts et al. Citation2014). Females with a mean (± SD) carapace length of 33.2 mm ± 5.1 mm were selected randomly (n = 62) and transferred to a recirculating natural seawater aquarium (12 h:12 h light:dark photoperiod, at 9.4°C ± 0.6°C SD) in the University of Glasgow for two weeks. All animals were fed with c. 1 g squid mantle three times a week (the ‘standard food ration’) for an initial two-week period, until the trial started on 26 October 2010.
Animals were assigned randomly to one of 14 numbered tanks. The animals in two tanks had the standard food ration (‘fed’ group), and food was removed from the tanks after 20 h. Those in the other 12 tanks were starved (‘unfed’ group). One unfed animal was removed from each even-numbered tank at weeks 0, 8 and 16, and one unfed animal was removed from each odd-numbered tank at weeks 4, 12 and 20. As there were no major changes in nutritional status over the course of the trial in the male fed group (Watts et al. Citation2014), the fed group of females was sampled once at week 20.
Animals were put on ice for 20 minutes prior to measurements of carapace length (with dial callipers) and weight without claws (to avoid differences due to claw loss during trawling and post-capture handling). The hepatopancreas and ovary were then removed from the cephalothorax (noting the colour and weight of the organs), and all muscle tissue was removed from the abdomen. These three tissues were frozen in liquid nitrogen and then stored at −80°C prior to further analysis.
The biophysical measure of hepatosomatic index and the biochemical measures of hepatopancreas water, lipid and copper content, along with abdominal tail muscle protein, water, the stable isotopes of δ15N, δ13C and the carbon-nitrogen ratio (C:N), were made on each animal, using methods described by Watts et al. (Citation2014).
Field study
Starting in January 2009, Nephrops norvegicus were collected monthly (January–December) by trawling along the established transect in the CSA mentioned above. Three research vessels from University Marine Biological Station Millport were used throughout the sampling period (RV Aora, RV Aplysia and RV Actinia), each equipped with an otter trawl with a mesh size of 70 mm at the cod-end. Trawls lasted for 1 h, at a mean depth of 78.5 m ± 1.1 m SD.
Assessment of reproductive state
A sub-sample of Nephrops norvegicus was taken non-selectively from each catch before any other animals were removed for other purposes. A random sample of the catch was placed into a fishing basket. The by-catch was then removed and discarded, leaving approximately 200 N. norvegicus (depending on the size of the catch). When the catch had fewer than 200 animals the entire catch was recorded. The sample was placed in a polystyrene box and covered with ice and transported to the University of Glasgow. This sample was measured within 24 h of the catch, and when not measured the same night the animals were refrigerated overnight (5°C).
The carapace length, weight without chelipeds, and sex of all N. norvegicus were recorded. The colour and weight of the hepatopancreas, and the colour and weight of the ovaries in females, were also recorded in a randomly selected sample of 25 of each sex.
Assessment of nutritional state
An additional 15 Nephrops norvegicus of each sex were sampled from the remainder of the catch from each haul. Their moult stage, according to carapace hardness, was determined as pre/post moult (soft), immediate post-moult (‘jelly’) or intermoult (hard) (Milligan et al. Citation2009). Intermoult animals were taken preferentially, and postmoult animals were used only when low numbers of animals were caught. Animals were put on ice and taken ashore, and were processed within 4 h. The carapace length, body weight, hepatopancreas colour and weight, and gonad colour, stage and weight were recorded. Samples of abdominal tail muscle and hepatopancreas were taken, frozen in liquid nitrogen and transported to the University of Glasgow, where they were stored at −80°C until all samples were collected and processed further. Biophysical and biochemical measures were then taken for each animal and assessed against the biophysical and biochemical measurements.
Statistics
Variation in biophysical or biochemical measurements over time and in relation to nutritional status were tested with general linear models (GLM, computed in the statistical program Minitab). For unfed animals in the aquarium study the factor ‘time’ was represented by weeks since last fed as a categorical variable. Differences between fed and unfed animals were tested in Nephrops norvegicus sampled at week 20. For the field study the factor ‘date’ was represented by the months caught, with males and females assessed separately. Normality of residuals and homogeneity of variances were assessed visually. A significant result was determined when P < 0.05 and the Tukey multiple comparison procedure was followed for both studies.
Results
Aquarium study
The results obtained from the fed and unfed groups of female Nephrops norvegicus during the 20-week experimental period are presented in (means + SE). Values of fed and unfed females at week 20 are shown in Table SI (supplementary material).
Figure 1. Means (±SE) of (a) copper concentration in the hepatopancreas (in relation to wet weight); (b) hepatosomatic index (HSI); (c) lipid content of the hepatopancreas; (d) water content of the hepatopancreas in unfed (black bars) and fed (grey bars) groups of female Nephrops norvegicus during the starvation trial. Letters indicate the results of a Tukey test on the unfed group. Means that do not share a letter are significantly different (P < 0.05). Asterisks indicate a significant difference between fed and unfed groups at week 20 (P < 0.05), n = 6.
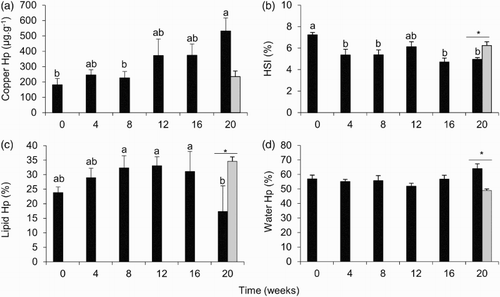
The concentration of copper in the hepatopancreas in unfed females increased significantly between week 0 and week 20 (F5,35 = 3.45, P = 0.014) (a). There was also a significant difference in the copper concentration in the hepatopancreas between fed and unfed animals at week 20 (F1,10 = 10.25, P = 0.011).
The HSI in unfed females decreased significantly between week 0 and week 4 and then remained relatively constant throughout the rest of the trial (F5,35 = 5.60, P = 0.001) (b). At week 20, fed females had a significantly higher HSI than unfed animals (F1,10 = 12.61, P = 0.006).
The lipid concentration in the hepatopancreas in unfed females initially increased between week 0 and weeks 8–16 and then decreased to lower than the initial value (F5,28 = 4.89, P = 0.003) (c). Hepatopancreas lipid content was significantly lower in unfed female N. norvegicus at week 20 (F1,9 = 10.45, P = 0.012).
The water content in the hepatopancreas in unfed females did not increase significantly between week 0 and week 20 (F5,35 = 2.29, P = 0.071) (d). However, there was a significant difference in the water content of the hepatopancreas between fed and unfed female N. norvegicus at week 20, being higher in unfed females (F1,8 = 10.13, P = 0.015).
For female N. norvegicus there was no significant variation among weeks or between fed and unfed groups in the measurements of abdominal tail muscle water (F5,35 = 0.86, P = 0.518; F1,8 = 3.31, P = 0.112), abdominal tail muscle δ13C (F5,35 = 2.03, P = 0.103; F1,8 = 1.63, P = 0.243), abdominal tail muscle δ15N (F5,35 = 0.54, P = 0.745; F1,8 = 1.38, P = 0.279) or the abdominal tail muscle C:N ratio (F5,35 = 0.69, P = 0.635; F1,8 = 1.02, P = 0.347) (Table SI).
The proportion of each ovary stage at each time period is shown in . Within the first four weeks four out of six females reabsorbed their ovaries. From the females that were fed throughout the trial, two of them displayed white gonads and one had cream gonads, showing no utilization of ovaries as reserves in these individuals during the trial. Ovary development did not occur within half of the unfed individuals, but rather there was an increasing amount of reabsorption.
Figure 2. Number of females at various ovary stages sampled during the aquarium trial. White, stage 0 (white ovaries); light grey, stage 1 (cream ovaries); grey, stage 2 (pale green ovaries); black, stage 3 (dark green ovaries); white with dots, stage 4 (dark green and swollen eggs visible); white with hatching, stage 5 (mottled green reabsorbed). After Tuck et al. (Citation1997c).
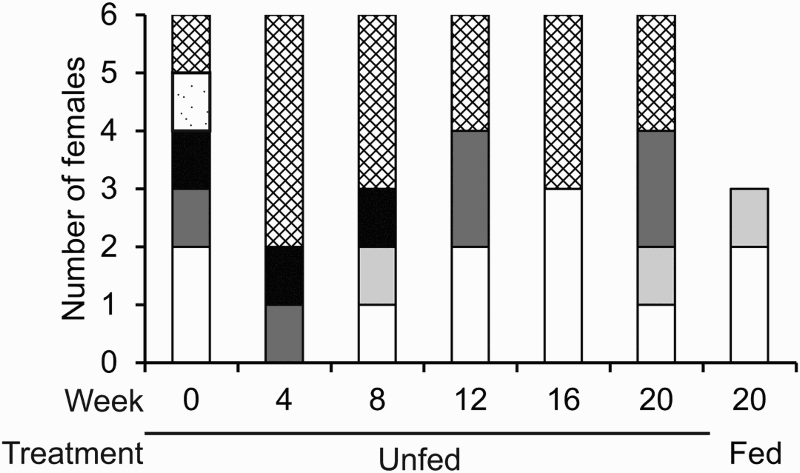
Field studies
Nutritional status
Females
Only one female was collected in the whole catch in January, and therefore this month was removed from all further analyses. Only mature females (<26 mm) were used for nutritional status measurements. Average sizes of male and females each month can be seen in Table SII.
When female Nephrops norvegicus were measured monthly throughout 2009 there was significant seasonal variation in hepatopancreas copper concentration (F10,115 = 3.98, P < 0.001) (a), lipid content (F9,67 = 5.29, P < 0.001) (e), and water content (F9,133 = 9.90, P < 0.001) (g). However there was no significant variation in HSI (F7,107 = 1.76, P = 0.104) (c). Females caught in February had an elevated copper concentration in the hepatopancreas, as found in males but to a smaller extent. The water content of the hepatopancreas was high in the months February–June and lipid content of the female hepatopancreas was 14–24% throughout the year and only exceeded 30% (30.5 ± 3.47%) in December (Table SIII).
Figure 3. Means (±SE) of (a,b) copper concentration in the hepatopancreas (in relation to wet weight); (c,d) hepatosomatic index (HSI); (e,f) lipid content of the hepatopancreas; (g,h) water content of the hepatopancreas in field-caught female (left panels) and male (right panels) Nephrops norvegicus in 2009 (circles). With unfed (square with cross) and fed (square no cross) means ± SE shown in each graph as a comparison; values derived from this study (females) and from Watts et al. (Citation2014) (males) shown.
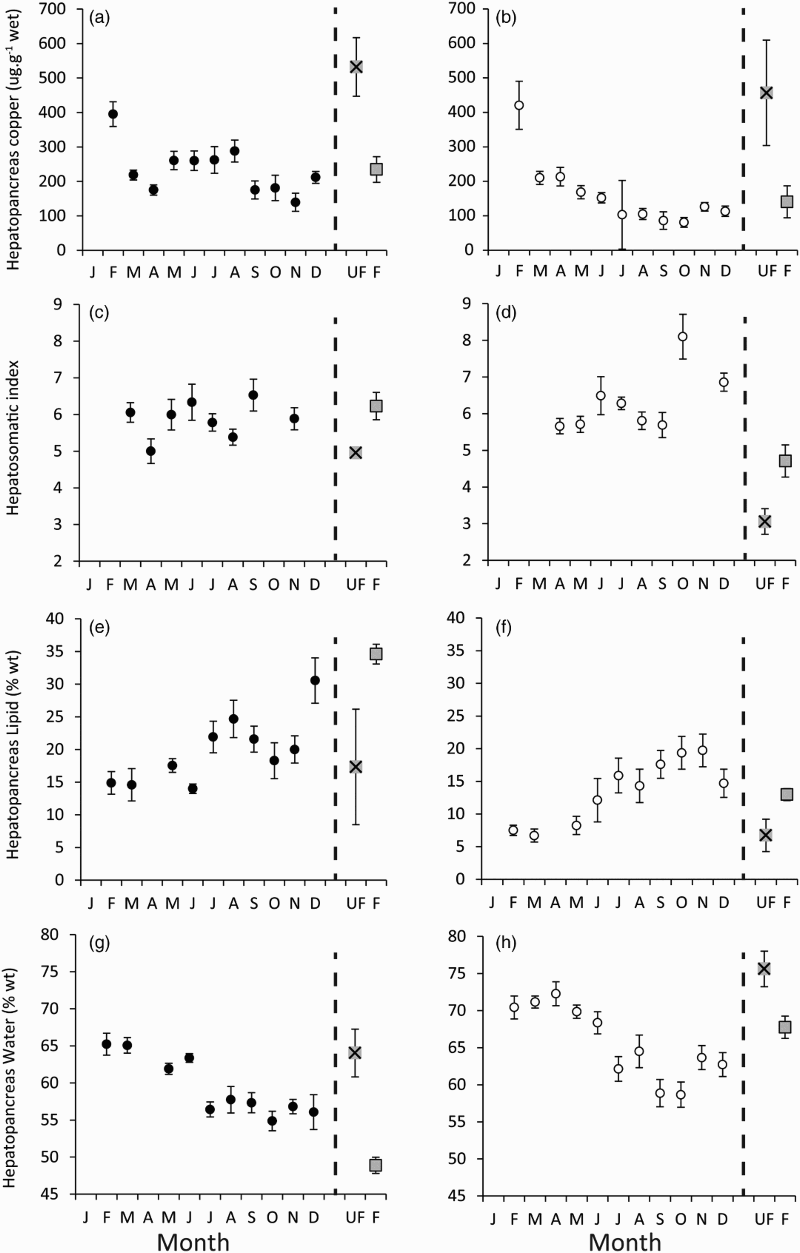
Males
In males, there was significant seasonal variation in hepatopancreas copper concentration (F10,129 = 15.22, P < 0.001) (b), HSI (F7,112 = 5.17, P < 0.001) (d), hepatopancreas lipid content (F9,63 = 4.34, P < 0.001) (f) and hepatopancreas water content (F10,140 = 7.72, P < 0.001) (h). The hepatopancreas copper values in males collected from the field in February were 420.34 ± 69.81 μg g−1. Males caught between February and April also had values of hepatopancreas water and lipid content comparable to the values observed in unfed individuals (Table SIII). Later in the year (September) all parameters measured indicated that males were well fed, with values closer to the fed group (aquarium trial).
Female reproductive state
The seasonal pattern in the proportion of all females caught in the trawl catches along the sampled transect is shown in a. The main features for females were low numbers during the winter months, an increasing proportion in the spring, peak abundance in the summer, a declining proportion in the autumn and a return to low numbers in the winter.
Figure 4. (a) Sex composition of the trawl catch from the Clyde Sea Area transect in each month of 2009. White section of bar represents proportion of the catch which are immature females and the black bars represent the proportion of the catch which are mature females; (b) percentage of females at each ovary maturation stage (see scale in legend); (c) percentage of females that were ovigerous.
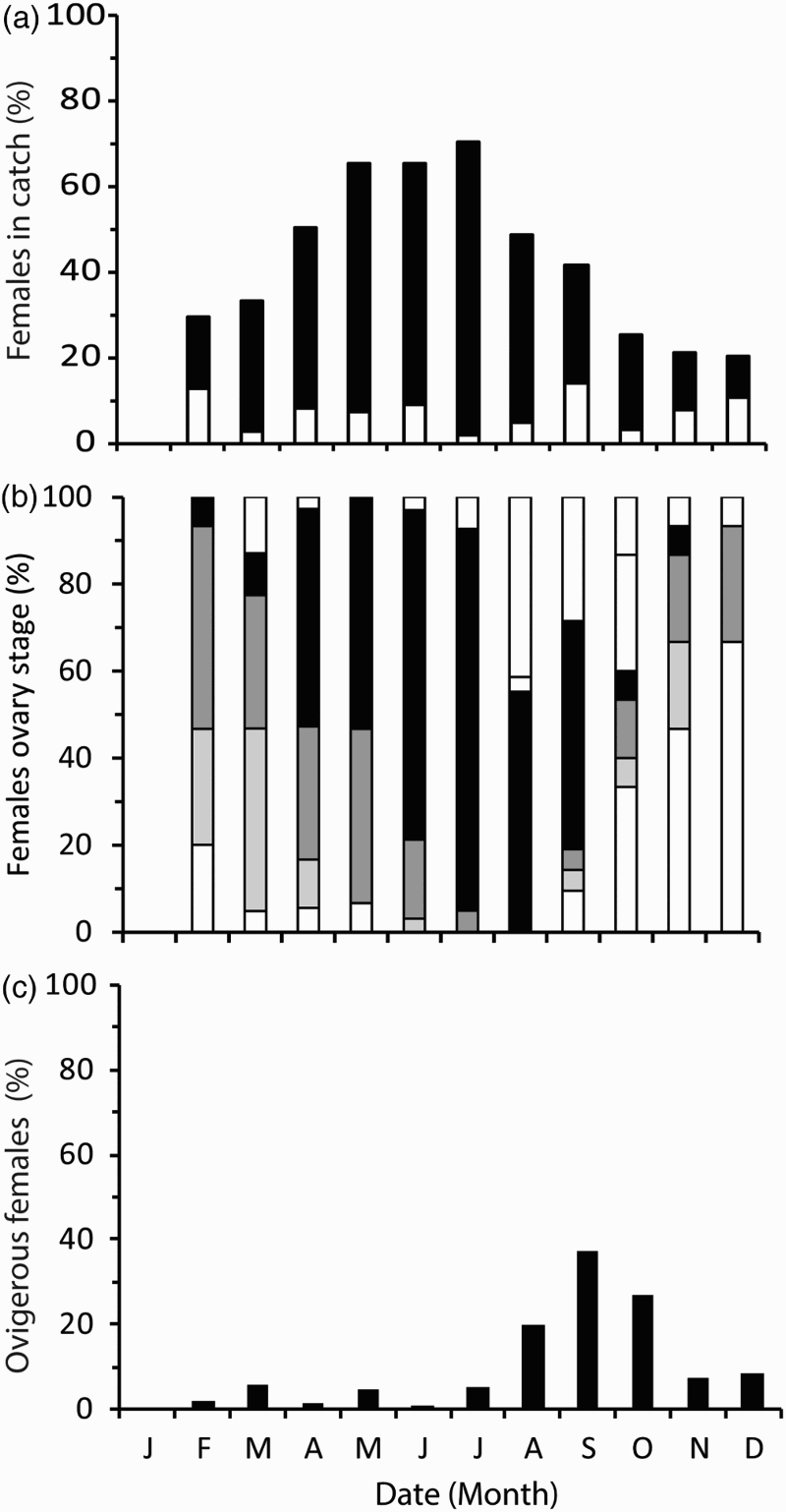
The seasonal pattern in the proportion of females in each stage of ovary maturation is shown in b. The females caught in the winter months had less-well developed ovaries than those in the summer (although as shown in a some of these may be immature). A progression in the maturation cycle can be seen within the first half of the year (January–July). In April 50% of females had green ovaries, and were therefore mature and ready to mate. This increased to 87.8% in July, as all females sampled had ovaries developed to stage 3 or beyond. In the second half of the year an increased proportion of females caught had spent ovaries, indicating that they had spawned recently.
The seasonal pattern in the proportion of females with eggs is shown in c. Ovigerous females were caught in highest numbers in September, with 37% of all females being berried prior to their winter burrow period. Only 1% and 4% of the newly emerged females in April and May, respectively, were ovigerous, suggesting that they had released the eggs prior to the collection.
Expressing the sizes of both sexes according to size categories (10–19.9, 20–29.9, 30–39.9, 40–49.9 and 50+ mm CL), a distinct sex difference was found in the seasonal fluctuation of body size of the animals in the random sample (a females, b males). The size distribution of the males fluctuated throughout the year. Females caught in the winter were mainly less than 30 mm CL, while in the summer there was a greater proportion in the 30–39.9 mm CL category. The dotted line indicates the division between mature (above) and immature (below) female Nephrops norvegicus. This shows that a higher proportion of female N. norvegicus caught in the months of February, November and December were immature.
Figure 5. Size distributions (according to carapace length) of (a) female and (b) male Nephrops norvegicus in trawl catch from the Clyde Sea Area transect in each month of 2009. White (10–19.9 mm), light grey (20–29.9 mm), grey (30–39.9 mm), dark grey (40–49.9 mm), black (50 mm+). Black dashed line represents size at onset of maturity of females (SOM = 26 mm CL).
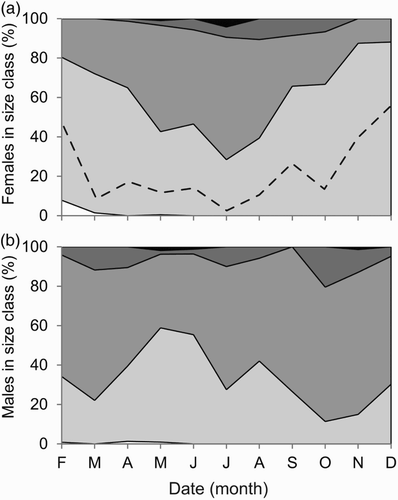
Discussion
The aim of this study was to determine if the burrow-bound behaviour of female Nephrops norvegicus through the winter months, which is related to their annual reproductive cycle, leads to a reduced nutritional state compared with males.
To do this, biochemical and biophysical measurements that have been shown to indicate the nutritional state of male N. norvegicus (Watts et al. Citation2014) were determined in females both under controlled conditions and from the wild. In males, the HSI, hepatopancreas copper concentration, and lipid and water content all changed significantly during starvation under controlled conditions (Watts et al. Citation2014). HSI and lipid content decreased as the hepatopancreas decreased in size, whereas the water and copper content of the hepatopancreas increased due to a replacement of lipids with water. In the present study, in starved females (i.e. food deprivation) the HSI, hepatopancreas copper, and lipid and water content also changed due to nutritional limitation (as in males). However, the C:N ratio of female abdominal tail muscle did not decrease significantly in unfed individuals as it did in males. Also, females maintained lipid reserves longer in the hepatopancreas (around 31% ± 6.9% remaining at week 16, compared with 13% in males).
It is therefore likely that under nutritionally limited conditions females will utilize lipids stored in the hepatopancreas only as a last resort. Females will still develop ovaries up to stage 2 over the six months of the burrow dwelling period (Farmer Citation1974). Tuck et al. (Citation1997c) suggest that starvation could be one reason for ovary reabsorption. In the present study, ovary development did not occur within half of the unfed individuals, but rather there was an increasing amount of reabsorption. Thus, for the population to remain stable females would still have to consume food to gain energy for this development. For reproductive events to take place in the following years it is likely that females will have to eat while in their burrows. The sex ratios recorded monthly through the year conformed to the traditional pattern of increasing emergence of females from their burrows during the spring (Oakley Citation1978; Bailey Citation1984; Briggs Citation1995; Tuck et al. Citation1997a; Milligan et al. Citation2009). Over the winter, females were far less abundant in trawl catches, consistent with them spending much of their time within their burrows. Females in the summer dominated catches, rising to 70% of all N. norvegicus in the catch; this was also seen in Milligan et al. (Citation2009) who sampled the same population in 2005–2006. The reason for this is currently unclear; however, it could relate to an increased exploitation of males over the winter, or females having greater foraging dominance than males at this time. This would be interesting to investigate further. Furthermore, females captured in trawls during the winter months were small (<30 mm CL) and in December around 50% were immature. Thomas & Figueiredo (Citation1965) showed that immature females show no seasonal variation in capture abundance. Females caught in April and May therefore include a high proportion of the females emerging from their burrows after egg hatching, and their nutritional status may thus reflect a long period of burrow residence. Consistent with Milligan et al. (Citation2009), the number of females appearing in the May catches was higher than that of males (70% females, 30% males). It is not clear why this is not 50:50.
In field-caught N. norvegicus there was significant variation in lipid, water and copper content of the hepatopancreas measured in both female and male individuals throughout the year. The results obtained from animals caught from February to June indicated that during this period animals may have a reduced nutritional status compared with that seen in July to December. Copper concentrations in the hepatopancreas were high in February in both males and females. Lipid and water contents of the hepatopancreas were also elevated. These findings suggest that during the winter both male and female N. norvegicus have reduced nutritional status, but not to the degree seen after 20 weeks of starvation in the aquarium trials. Temperatures in the aquarium trials were consistent with temperatures in the summer, but around 2°C higher than in the winter. Therefore, the greater effect seen in the aquarium trial could be due to an increased metabolism in these animals. There is no evidence that females had a lower nutritional status than males when comparing sex-specific starvation rates (even though females had higher copper values than males from May to October and lipids from May to September). In September, when the maximum numbers of ovigerous females were found, their nutritional state was consistent with that of fed animals in the aquarium trial. This suggests that females are entering their winter burrowing period in a good nutritional state. This is consistent with the results from quarterly samples of N. norvegicus taken in 1992–93 (IMBC et al. Citation1994) and bimonthly samples in 1995–96 (Parslow-Williams Citation1998). Those studies showed that the lowest lipid and highest water content in the hepatopancreas occurred in the spring and vice versa for the summer. However, HSI did not vary significantly between these two seasons in this study.
It is therefore likely that sex-specific interactions play a fundamental part in the nutritional status of N. norvegicus. Reasons for the reduced nutritional status could include lower food availability and less consumption of the food that is present. As explained in the introduction, food available to benthic organisms ultimately comes from the water column above, and in the winter months there is a reduction in primary production in the water column and thus the amount being driven down to the benthos. This theory was also suggested by IMBC et al. (Citation1994). Less consumption of any food that is present is also possible during the winter due to a reduced burrow emergence. This might be as a response to lower light levels or lower temperatures, causing N. norvegicus to remain in their burrows for more extended periods of time in the winter months (Maynou & Sarda Citation2001). It is known that spring represents the time of post-brooding burrow emergence of females in the Clyde population of N. norvegicus (Milligan et al., Citation2009), and similarly in our study April was the first month in which the proportion of females in the catch reached 50%. At this time female N. norvegicus do seem somewhat limited nutritionally (due to a high hepatopancreas water percentage), but, when considering hepatopancreas copper concentrations, no more so than male N. norvegicus and less so than females in March and May. Oakley (Citation1978) observed that in the winter months of a controlled experiment under artificial conditions (November–May) ovigerous females rarely came out beyond 25 cm from the burrow entrance to collect food that had been detected, and carried large food items into their burrow systems for consumption. It is also known that N. norvegicus will bury food within their burrows, and females kept for an extended period of time in a mesocosm have shown ‘caching’ behaviour by burying food items away from the burrow, presumably so as not to attract predators (Atkinson & Eastman Citation2015). This suggests that females could in fact ‘prepare’ for the winter months by creating a readily accessible food store.
In conclusion, this study does not support the hypothesis that females cease feeding over winter. Firstly, some females were unable to sustain ovary development during starvation under controlled conditions, contrary to field observations. Secondly, field data suggest that there is no sex-specific reduction in nutritional status.
Disclosure statement
No potential conflict of interest was reported by the authors.
Tables SI-SIII
Download MS Word (34.6 KB)ORCID
Andrew J. R. Watts http://orcid.org/0000-0003-0623-4601
Additional information
Funding
References
- Aguzzi J, Company JB, Sarda F. 2007. The activity rhythm of berried and unberried females of Nephrops norvegicus (Decapoda, Nephropidae). Crustaceana 80:1121–34. doi:10.1163/156854007782008577
- Atkinson RJA, Eastman LB. 2015. Burrow dwelling in Crustacea. In: Thiel M, Watling L, editors. Lifestyle and Feeding Biology. The Natural History of the Crustacea Vol. 2. Oxford: Oxford University Press, p 78–117.
- Avarre JC, Michelis R, Tietz A, Lubzens E. 2003. Relationship between vitellogenin and vitellin in a marine shrimp (Penaeus semisulcatus) and molecular characterization of vitellogenin complementary DNAs. Biology of Reproduction 69:355–64. doi:10.1095/biolreprod.102.011627
- Baden SP, Depledge MH, Hagerman L. 1994. Glycogen depletion and altered copper and manganese handling in Nephrops norvegicus following starvation and exposure to hypoxia. Marine Ecology Progress Series 103(1–2):65–72. doi: 10.3354/meps103065
- Bailey N. 1984. Some aspects of reproduction in Nephrops. International Council for the Exploration of the Sea C.M. 1984/K 33:1–39.
- Beevers ND, Kilbride E, Atkinson RJA, Neil DM. 2012. Hematodinium infection seasonality in the Firth of Clyde (Scotland) Nephrops norvegicus population: a re-evaluation. Diseases of Aquatic Organisms 100(2):95–104. doi:10.3354/dao02473
- Bell MC, Redant F, Tuck ID. 2006. Nephrops species. In: Phillips BF, editor. Lobster. Biology, Management, Aquaculture and Fisheries. Oxford: Wiley-Blackwell Scientific Publishing, p 412–61.
- Briggs RP. 1995. Variability in northwest Irish Sea Nephrops populations. Fisheries Research 23:175–87. doi:10.1016/0165-7836(94)00332-Q
- Campbell N, Allan L, Weetman A, Dobby H. 2009. Investigating the link between Nephrops norvegicus burrow density and sediment composition in Scottish waters. ICES Journal of Marine Science 66:2052–59. doi:10.1093/icesjms/fsp176
- Chapman CJ, Bailey N. 1987. Biological research on fish and shellfish stocks – recent progress in Norway lobster research. In: Bailey RS, Parrish BB, editors. Developments in Fisheries Research in Scotland. Farnham, UK: Fishing News Books, p 99–111.
- Chapman CJ, Rice AL. 1971. Some direct observations on ecology and behaviour of Norway lobster Nephrops norvegicus. Marine Biology 10:321–29. doi:10.1007/BF00368092
- Comoglio L, Smolko L, Amin O. 2005. Effects of starvation on oxygen consumption, ammonia excretion and biochemical composition of the hepatopancreas on adult males of the False Southern King crab Paralomis granulosa (Crustacea, Decapoda). Comparative Biochemistry and Physiology B 140(3):411–16. doi: 10.1016/j.cbpc.2004.11.003
- Farmer ASD. 1974. Reproduction in Nephrops norvegicus (Decapoda: Nephropidae). Journal of Zoology 174:161–83. doi:10.1111/j.1469-7998.1974.tb03150.x
- IMBC, UMBSM, IRPEM. 1994. Nephrops norvegicus: stock variability and assessment in relation to fishing pressure and environmental factors. Final report to EC, Contract XIV-1/MED/91/003. Institute of Marine Biology of Crete, IMBC, University Marine Biological Station Millport, Istituto di Ricerche sulla Pesca Marittima, Crete. 84 pages.
- Karapanagiotidis IT, Mente E, Berillis P, Rotllant G. 2015. Measurement of the feed consumption of Nephrops norvegicus feeding on different diets and its effect on body nutrient composition and digestive gland histology. Journal of Crustacean Biology 35:11–19. doi:10.1163/1937240X-00002299
- Loo LO, Baden SP, Ulmestrand M. 1993. Suspension feeding in adult Nephrops norvegicus (L.) and Homarus gammarus (L.) (Decapoda). Netherlands Journal of Sea Research 31:291–97. doi:10.1016/0077-7579(93)90029-R
- Macleod R, Clark J, Cresswell W. 2008. The starvation-predation risk trade-off, body mass and population status in the common starling Sturnus vulgaris. Ibis 150:199–208. doi:10.1111/j.1474-919X.2008.00820.x
- Maynou F, Sarda F. 2001. Influence of environmental factors on commercial trawl catches of Nephrops norvegicus (L.). ICES Journal of Marine Science 58:1318–25. doi:10.1006/jmsc.2001.1091
- Mente E, Karapanagiotidis IT, Logothetis P, Vafidis D, Malandrakis E, Neofitou N, et al. 2009. The reproductive cycle of Norway lobster. Journal of Zoology 278:324–32. doi:10.1111/j.1469-7998.2009.00579.x
- Milligan RJ, Albalat A, Atkinson RJA, Neil DM. 2009. The effects of trawling on the physical condition of the Norway lobster Nephrops norvegicus in relation to seasonal cycles in the Clyde Sea Area. ICES Journal of Marine Science 66:488–94. doi:10.1093/icesjms/fsp018
- Newland PL. 1985. The Control of Escape Behaviour in the Norway Lobster, Nephrops norvegicus (L). PhD Thesis. University of Glasgow. 158 pages.
- Oakley S. 1978. Food, Feeding Behaviour and Some Aspects of the Ecology of Nephrops norvegicus in the Irish Sea. PhD thesis. University of Liverpool. 231 pages.
- Parslow-Williams PJ. 1998. Nutritional Limitation in Populations of the Norway Lobster, Nephrops norvegicus (L.) in the Firth of Clyde, Scotland. PhD thesis. University of Glasgow. 238 pages.
- Roots C. 2006. Hibernation. London: Greenwood Press. 219 pages.
- Rotllant G, Ribes E, Company JB, Durfort M. 2005. The ovarian maturation cycle of the Norway lobster Nephrops norvegicus (Linnaeus, 1758) (Crustacea, Decapoda) from the western Mediterranean Sea. Invertebrate Reproduction and Development 48:161–69. doi:10.1080/07924259.2005.9652182
- Stephens K, Sheldon RW, Parsons TR. 1967. Seasonal variations in availability of food for benthos in a coastal environment. Ecology 48:852–55. doi:10.2307/1933743
- Thomas HJ, Davidson C. 1962. The food of the Norway lobster. Marine Research 3:1–15.
- Thomas HJ, Figueiredo MJ. 1965. Seasonal variations in the catch composition of the Norway lobster, Nephrops norvegicus (L.) around Scotland. ICES Journal of Marine Science 30:75–85. doi:10.1093/icesjms/30.1.75
- Tuck ID, Chapman CJ, Atkinson RJA. 1997a. Population biology of the Norway lobster, Nephrops norvegicus (L.) in the Firth of Clyde, Scotland – I: growth and density. ICES Journal of Marine Science 54:125–35. doi:10.1006/jmsc.1996.0179
- Tuck ID, Chapman CJ, Atkinson RJA, Bailey N, Smith RSM. 1997b. A comparison of methods for stock assessment of the Norway lobster, Nephrops norvegicus, in the Firth of Clyde. Fisheries Research 32:89–100. doi:10.1016/S0165-7836(97)00035-0
- Tuck ID, Taylor AC, Atkinson RJA, Gramitto ME, Smith C. 1997c. Biochemical composition of Nephrops norvegicus: changes associated with ovary maturation. Marine Biology 129:505–11. doi:10.1007/s002270050191
- Tuck I, Bailey N, Atkinson J, Marrs S. 1999. Changes in Nephrops density in the Clyde Sea Area, from underwater TV survey data. Study group on life history of Nephrops. ICES CM 1999/G 13:24–31.
- Waddy SL, Aiken DE, de Kleijn DPV. 1995. Control of growth and reproduction. In: Factor JR, editor. Biology of the Lobster Homarus americanus. San Diego, CA: Academic Press, p 217–66.
- Watts AJR, McGill RAR, Albalat A, Neil DM. 2014. Biophysical and biochemical changes occur in Nephrops norvegicus during starvation. Journal of Experimental Marine Biology and Ecology 457:81–89. doi:10.1016/j.jembe.2014.03.020