Abstract
Metal-on-metal hip resurfacing arthroplasty has undergone a recent resurgence as an alternative treatment option for young and active patients with significant osteoarthritis. The claimed advantages of metal-on-metal hip resurfacing arthroplasty include lower wear rate, preservation of bone stock for subsequent revision procedures, restoration of anatomic hip mechanics, and enhanced stability due to the larger diameter of articulation. A disadvantage, however, is that the metal-on-metal resurfacing releases large amounts of very small wear particles and metal ions. The long-term biological consequences of the exposure to these Co-Cr particles and ions remain largely unknown. The purpose of this review is to provide an overview of the current literature on the adverse periprosthetic biological reactions associated with metal-on-metal hip resurfacing arthroplasty.
With the introduction of the metal-on-metal (MoM) bearing to hip resurfacing, there has been a recent and rapid increase in hip resurfacing (Daniel et al. Citation2004). According to the National Joint Registry England and Wales Annual Report (2008), the total number of hip resurfacing in the patient group younger than 55 years of age accounted for 46% (1585 of 3471) of primary hip arthroplasty performed in the United Kingdom in 2004. In Australia, the hip resurfacing procedures accounted for 29% of the overall number of primary total hip arthroplasties for the patients in the same age group (Buergi and Walter Citation2007). In Norway and Sweden hip resurfacing has been used conservatively and it accounted for 0.4% and 0.6% respectively in 2006 (2006, 2007). Hip resurfacings are used particularly in younger, more active patients because of the limitations of conventional total hip arthroplasty (THA) (Daniel et al. Citation2004). MoM bearings have better wear properties than conventional metal-on-polyethylene (MoP) bearings (Sieber et al. Citation1999, MacDonald et al. Citation2003). They have the potential to substantially reduce long-term wear-induced osteolysis as the major cause of failure. Short-term clinical follow-up reports on MoM hip resurfacing have been encouraging (Sieber et al. Citation1999, MacDonald et al. Citation2003, Treacy et al. Citation2005, Hing et al. Citation2007, Steffen et al. Citation2008) with extremely low rates of failure reported in spite of the fact that patients have resumed high-level occupational and leisure activities. Medium- to long-term outcome is, however, unknown.
The reported wear rate from MoM bearings is substantially lower than from MoP bearings. An annual linear wear rate of 5 µm for the whole articulation per year has been reported (Sieber et al. Citation1999). This is at least 20 times less than that of MoP bearings. The metal particles produced are less than 50 nm—approximately one order of magnitude smaller than retrieved polyethylene particles (Doorn et al. Citation1998). Although the wear rates at the surfaces of MoM bearings are lower than for conventional MoP bearings, the number of nano-meter-sized particles generated is up to 500 times more than for MoP bearings (Sieber et al. Citation1999). The dissolution of metal wear particles results in measurable increases in cobalt (Co) and chromium (Cr) ions in the serum, erythrocytes, and urine of patients with MoM bearings (Coleman et al. Citation1973, Jacobs et al. Citation1998, Savarino et al. Citation2002, Brodner et al. Citation2003, MacDonald et al. Citation2003, Rasquinha et al. Citation2006). The potential effects of elevated levels of metal particles and ions are poorly defined, but they may lead to adverse biological reactions including local soft tissue toxicity, hypersensitivity reactions, bone loss, and risk of carcinogenesis (Gillespie et al. Citation1996, Shimmin et al. Citation2005, Willert et al. Citation2005, Keegan et al. Citation2007, Lidgren Citation2008). There is also concern about chromosomal aberrations in the patient, as well as the risk of passing chromosomal abnormalities to the next generation (Case et al. Citation1996, Doherty et al. Citation2001, Ladon et al. Citation2004, Brodner et al. Citation2004a, Papageorgiou et al. Citation2007, Ziaee et al. Citation2007)
In this review we present the available evidence on adverse periprosthetic biological reactions in patients with the MoM hip resurfacing arthroplasty. The possible role of the metal particle-induced immunological and cellular responses in the pathogenesis of adverse periprosthetic reactions is outlined.
MoM hip resurfacing arthroplasty
The first generation of hip resurfacing arthroplasties with MoP bearings that were implanted during the 1970s and early 1980s had poor outcome (Head Citation1981, Bell et al. Citation1985). The clinical failures were largely due to high volumetric wear as a function of the large-diameter metal femoral component articulating within polyethylene cups or liners, leading to wear debris induced osteolysis. The renewed interest in hip resurfacing follows the introduction of the MoM articulation. This was in part based on the observation that some of the early MoM prostheses have survived for over 20 years, with low wear and no incidence of osteolysis (McKellop et al. Citation1996, Howie et al. Citation2005).
Particles generated from the articular surfaces of MoM prostheses have a size range in the µm scale. Doorn et al. (Citation1998) used transmission electron microscopy to visualize the wear particles generated in vivo following enzymatic digestion of peri-prosthetic tissues from 13 revised MoM prostheses. It was found that most of the particles were round, although a small proportion were shard or needlelike in morphology. Mean particle size was 81 (51– 116) nm. It has been estimated that the implants in 3 of the patients investigated would have generated between 6.7×1012 and 2.5×1014 metal particles per year. Buschner et al. (Citation2004) theorized that generation of wear particles in MoM implants may be due to fatigue of martensitic bands (quadratic organization of iron atoms in the metal crystal), and at areas of high contact pressures the grain size of the metal alloy could influence the morphology of the particles generated.
The results of published short-term survivorship studies of the current generation of MoM hip resurfacing prostheses are encouraging and represent a substantial improvement from the earlier generation of hip resurfacing prostheses (). Treacy et al. (Citation2005) reported 98% survivorship of the Birmingham Hip Resurfacing (BHR) arthroplasty (Midland Medical Technologies, Birmingham, UK) after 5 years. In a recent independent study, Steffen et al. (Citation2008) reported a survival analysis of 610 BHRs implanted with a minimum follow-up of 2 years and a review of patients 5 years postoperatively. The survivorship was 95% at 5 years. Another independent prospective review of 230 BHRs by Hing et al. (Citation2007) reported 98% survivorship at a mean of 5 years.
Table 1. Survivorship for the currently used hip resurfacing systems
The published reports on the modern MoM bearings have uniformly shown higher serum Co and Cr levels when compared to preoperative values, and in comparison to the levels in patients with conventional MoP bearings (Savarino et al. Citation2002, Brodner et al. Citation2003, Clarke et al. Citation2003, MacDonald et al. Citation2003, Back et al. Citation2005b). The serum Co and Cr levels have been monitored prospectively following implantation of BHR prostheses (Back et al. Citation2005b, Daniel et al. Citation2007). The peak serum levels of Co (a 10-fold increase) and Cr (a 16-fold increase) occurred at 6 and 9 months respectively, compared to the preoperative levels. These peaks were followed by a gradual decline over the next 15 months.
There is recent evidence to suggest that Co and Cr levels are influenced by factors such as the type, design, and positioning of the implant. It is difficult to compare studies, however, because there is variation in the study design, the sources of samples (serum, whole blood, erythrocytes, and urine), the methods of laboratory analysis techniques used, and the various combinations of types of implant (Daniel et al. Citation2007) ().
Table 2. Studies on cobalt and chromium ion levels
The effect of the diameter of MoM bearings on metal levels remains controversial. For instance, a greater increase in Co and Cr levels in patients with MoM hip resurfacing (mean diameter 48 (38–54) mm) in comparison to patients with 28-mm MoM THAs has been demonstrated (Clarke et al. Citation2003). In contrast, a more recent study found no significant difference in Co and Cr levels between the resurfacing group (50 mm or 54 mm diameter) and the MoM THA group (28 mm diameter) (Daniel et al. Citation2006). Comparison of these study results is difficult due to the different sources of samples that were used to measure Co and Cr levels. Serum analyses were used in the first study whereas whole blood levels were measured in the second study. Furthermore, implant design variables between the different types of MoM resurfacing hip arthroplasties and MoM THAs investigated in each study may have influenced the Co and Cr levels.
Metal ion levels have been shown to be influenced by specific MoM resurfacing implant designs (). Vendittoli et al. (Citation2007) measured whole blood Co and Cr ion concentrations in patients with the Durom hip resurfacing system (Zimmer, Winterthur, Switzerland), which has a high-carbon-content wrought-forged MoM resurfacing hip arthroplasty design. The authors reported lower mean serum Co and Cr levels in patients with this implant in comparison with other surface replacement arthroplasty prostheses.
Table 3. Currently available hip resurfacing systems
In addition to the design and the diameter of the MoM bearings, the position of the implant—which is influenced by the technical skill of the surgeon inserting the prosthesis—also plays a role in the degree of metal ion release. The effect of acetabular component abduction angle on serum metal levels has been investigated. 10 to 53-fold elevated Co levels and 9 to 30-fold elevated Cr levels were observed in patients with acetabular cup inclinations of 58° and 63° (Brodner et al. Citation2004a). However, no statistically significant correlation between cup inclination and serum Co and Cr was found in this study.
Unlike most organic chemicals, metals cannot be eliminated from tissues by metabolic degradation. Thus, they can only be eliminated from tissues by renal or gastrointestinal excretion (Cobb and Schmalzreid Citation2006). There is evidence from a recent animal study to suggest that Cr ions can accumulate in the liver (Jakobsen et al. Citation2007). Marker et al. (Citation2007) investigated potential long-term effects on kidney function resulting from Co and Cr wear in MoM THA by measuring serum metal ion levels and creatinine clearance at 10-year follow-up in 75 patients. The serum creatinine clearance was normal in all patients. The study cohort included 7 patients with bilateral THA; their creatinine clearance was similar to the values of patients with unilateral THA. Furthermore, the steady-state metal ion levels appear to be insensitive to short-term changes in activity level (Heisel et al. Citation2005).
Adverse periprosthetic effects of metal wear debris
Although it is still unclear how metal particles or ions cause periprosthetic problems, clinical cases of periprosthetic soft-tissue masses () and osteolysis have recently been reported.
Table 4. Reports of periprosthetic soft-tissue mass in MoM bearings
Periprosthetic soft-tissue mass
Pandit et al. (Citation2008) reported the presence of symptomatic soft-tissue masses in a series of 20 MoM resurfaced hips (17 female patients). Locally, the mass was highly destructive and the presenting symptoms varied between pain, rash, dislocation, femoral nerve palsy, and the presence of a lump. In all the cases, white cell count was normal. The inflammatory markers erythrocyte sedimentation rate (ESR) and C-reactive protein (CRP) were normal in 17 of the 20 patients. The main imaging abnormalities included a cystic mass located lateral or posterior to the joint and a predominantly solid mass located anteriorly involving the psoas bursa and muscle. 13 patients required revision surgery to a conventional hip replacement, which led to the resolution of symptoms in 11 patients. Histologically, the lesions were characterized by the presence of B cells, T lymphocytes, and plasma cells, and extensive necrosis was noted. The authors suggested that the soft-tissue mass is either an allergic response to a normal level of metal wear particles, or a toxic effect of a very high level of particles. Although the precise incidence is unknown, the authors estimated the incidence to be 1% in the first 5 years postoperatively.
The presence of similar cystic soft-tissue reactions has been reported in a review of MRI findings in 19 patients (11 females) with early postoperative pain following Co-Cr on Co-Cr alloy THA prostheses (De Puy International Ltd., Leeds, UK) (Toms et al. Citation2008). The median postoperative time to MRI was 35 (11–63) months. These collections, surrounded by irregular walls, always maintained contact with the neck of the prosthesis, extending into the gluteal compartment in 18 cases. The MRI appearance was consistent with a periprosthetic abscess, but inflammatory markers and microscopy were normal in all cases. At the time of revision surgery, discrete periprosthetic soft tissue thickening, a fluid-filled cavity, or a combination of these findings was found in all 15 revised hips. Corrosion and severe pitting of the femoral components was demonstrated in 8 hips. Histological examination showed necrosis in 12 cases and perivascular lymphocytic infiltration in 5 cases. The authors suggested that early postoperative pain (less than 3 years) after MoM THA may be due to an abnormal soft tissue reaction. Isolated case reports of peri-prosthetic soft-tissue masses in patients with MoM hip resurfacing or MoM THA have been reported in the literature in the past (Madan et al. Citation2000, Boardman et al. Citation2006, Gruber et al. Citation2007).
Periprosthetic osteolysis
Periprosthetic osteolysis is a cellular response to wear debris (Harris Citation1995). It is a common reason for implant failure in MoP THA (Maloney et al. Citation1999, Sochart Citation1999). The likelihood of osteolysis has been correlated with the amount of wear particles (Dumbleton et al. Citation2002). The generation of wear particles depends on many factors including the properties of the bearing couple, component alignment, component fixation stability, and individual patients (Kadoya et al. Citation1998).
Osteolysis, occurring as a result of reaction to metal wear debris, has been reported in association with MoM THA in a small number of cases. Periprosthetic osteolysis was observed in 7 of 19 metal-on-metal implants revised for pain and swelling (Willert et al. Citation2005). Park et al. (Citation2005) reported a 6% incidence of periprosthetic osteolysis at 2 years following second-generation MoM THA (10/169 cases). The osteolysis was confined to the greater trochanter. A higher rate of hypersensitivity reactions to cobalt chloride skin testing was observed in patients with osteolysis compared to controls without osteolysis, indicating a delayed-type hypersensitivity reaction. The histological examination showed a perivascular accumulation of T lymphocytes. Immunohistochemical analysis showed elevated levels of bone-resorbing cytokines such as IL-1 and TNF-α produced by infiltrating lymphocytes and activated macrophages. Although a causal relationship could not be established from the study, the findings suggested that early osteolysis is associated with abnormalities consistent with delayed-type hypersensitivity to metal.
Histopathology of periprosthetic tissues
Assessment of the periprosthetic tissue reaction plays an important role as a diagnostic tool in evaluation of implant failures (Kobayashi et al. Citation1997b, Goodman et al. Citation1998). The retrieval study findings of an intense macrophage reaction to polyethylene wear debris in MoP THA explain the formation of osteolysis (Campbell et al. Citation1996, Green et al. Citation1998). There is recent evidence from retrieval studies to suggest a T lymphocyte-mediated immune reaction in patients with MoM articulation (Savarino et al. Citation2002, Davies et al. Citation2005, Willert et al. Citation2005, Witzleb et al. Citation2007).
Lymphocyte infiltrations in periprosthetic tissue, described as ALVAL, were observed in first- and second-generation MoM THA (Davies et al. Citation2005, Willert et al. Citation2005, Witzleb et al. Citation2007) as well as in MoM hip resurfacing arthroplasty (Pandit et al. Citation2008, Toms et al. Citation2008). Willert et al. (Citation2005) analyzed the periprosthetic tissues from 19 MoM implants that were revised for pain and swelling. The authors observed intense perivascular lymphocytic cuffing and suggested that a T lympho-cyte-mediated hypersensitivity reaction of type IV (delayed-type hypersensitivity) had resulted from the metal-wear particulate debris from the MoM bearing couple. In comparison with the periprosthetic tissues from MoP bearing implants, periprosthetic tissues from MoM hip resurfacing and total hip implants were found to have more synovial surface layer ulceration and to contain more lymphocytes and plasma cells and less macrophages (Davies et al. Citation2005).
Similar synovial surface ulceration and dense infiltration of lymphocytes in the subsurface tissue layer were reported in an animal study investigating the effects of intraarticular injection of metal particles (Howie and Vernon-Roberts Citation1988). This finding has led to the hypothesis that exposure to Co-Cr results in ulceration of the synovial surface layer and that the perivascular lymphocytic infiltration occurs as a secondary event. In contrast, in a recent histological and immunochemical analysis of capsule tissue samples retrieved from 46 hips with MoM articulations (39 cases of hip resurfacing and 7 cases of non-cemented THA), the superficial necrosis was not associated with the extent of lymphocytic infiltration or the extent of perivascular lymphocytic infiltration (Witzleb et al. Citation2007).
Perivascular lymphocytic vasculitis has been identified in some forms of arteritis, contact dermatitis, and discoid lupus erythematosus (Howie and Vernon-Roberts Citation1988). However, as the infiltrates of lymphocytes or plasma cells have not been reported in studies of tissues from MoP or ceramic-on-ceramic articulations, the distinct lymphocytic infiltration is thought to be a characteristic histological pattern of tissue reactions to MoM bearings (Davies et al. Citation2005, Willert et al. Citation2005, Witzleb et al. Citation2007).
Metal-induced immune responses
It has been documented that the size, shape, number, and nature of the particles released from the articular surfaces of prostheses are responsible for the type and extent of the biological response (Kobayashi et al. Citation1997a, Green et al. Citation1998). For the MoP prostheses, most of the particles are in the µm range with few in the submicron size, which corresponds to the size of bacteria (Margevicius et al. Citation1994, Shanbhag et al. Citation1994, Campbell et al. Citation1995). Polyethylene particles have been shown to elicit a foreign-body, granulomatous response simulating infection, which is non-specific and consists mainly of macrophages and fibroblasts with occasional lymphocytes. Co-Cr particles have an effect on cells that is different to that of polyethylene and most other biomaterial. Particles are commonly phagocytosed by macrophages. Once phagocytosed, Co-Cr particles can be toxic and rapidly kill the cells. This is probably because they corrode quickly within the cells, because of the acidic environment in the phago-lysosome—and release ions in high concentrations within the cells, leading to toxicity. The cells will then lyse, releasing the particles and cell contents to cause further damage (Rae Citation1986). As outlined previously, there is recent evidence that metal particles, which are orders of magnitude smaller than the polyethylene particles, induce an acquired or antigen-specific immune response, a type IV delayed hypersensitivity reaction driven by T lymphocytes (Willert et al. Citation2005, Witzleb et al. Citation2007). This involves a specific antigen, co-stimulatory molecules, an antigen presenting cell, and T lymphocytes. Periprosthetic tissue analysis has shown that metal particles are contained within CD46+ macrophages (Doorn et al. Citation1996, Davies et al. Citation2005, Witzleb et al. Citation2007). Macrophages can act as antigen presenting cells. It is likely that the continuous release of metal particles or ions from the metal-on-metal articulation and their local accumulation within the effective joint space facilitates sensitization, with a consequent cell-mediated immunological response. In some susceptible patients, this may lead to adverse periprosthetic reactions such as periprosthetic mass and osteolysis. Release of metal particles and metal ions can have further adverse effects: they can be transported to areas other than the joint cavities and initiate further osteolysis away from the affected joint. This concept of “effective joint space” has been described in detail by Schmalzried et al. (Citation1992).
A relationship between metal ion levels and lymphocyte counts in patients with well-functioning MoM hip resurfacings has been reported (Hart et al. Citation2006). A threshold whole blood level of Co and Cr combined of greater than 5 µg/L was found to be associated with a reduction in the circulating levels of T cytotoxic (CD8+) subsets in the MoM group. Despite this decrease in the CD8+ cell count, the authors were unable to associate this observation with any adverse clinical symptoms.
The possible role of metal-specific adaptive immune response in the pathogenesis of poor implant performance such as aseptic osteolysis has been investigated by Hallab et al. (Citation2005). The authors measured the lymphocyte responses to implant metals (Cr3+, Co2+, and Ni2+ at 0.1 mM and Ti4+ at 0.001 mM) in 6 subject groups: group 1a, young controls; group 1b, age-matched controls; group 2a, subjects with a history of OA and no history of metal sensitivity; group 2b, OA subjects with a history of metal sensitivity; group 3a, THA subjects with no or mild osteolysis; and group 3b, THA subjects with moderate osteolysis. Groups 1 and 2 had no implanted prostheses. Lymphocyte proliferation, measured using lymphocyte transformation testing (LTT), and cytokine release were used as quantitative reactivity measurements. THA subjects were more than 3-fold more reactive to Cr than were controls or osteoarthritis subjects. THA subjects with osteolysis were more reactive to Co (incidence of 43% as opposed to 0%). Only osteolytic THA subjects demonstrated increased cytokine responses with more than 2-fold increases in soluble interferon gamma (IFN gamma) and interleukin-2 levels in response to Cr challenge. These findings suggested a metal-specific adaptive immune response and a possible relationship with aseptic osteolysis. Hypersensitivity reaction to implanted metallic materials has been estimated to occur in less than 1% of patients undergoing total joint arthroplasty (Hallab et al. Citation2001).
Metal ions may bind to specific serum proteins to form metal-protein complexes (Torti and Torti Citation1994, Borguet et al. Citation1995, Sun et al. Citation1999, Bar-Or et al. Citation2001, Clodfelder et al. Citation2001, Qian et al. Citation2002, Eitinger et al. Citation2005). Such metal-protein complexes produced from degradation of metal alloys are immunologically active in vitro (Hallab et al. Citation2001). The complexes have been shown to induce lymphocyte activation through proliferative responses. The mechanism by which metal-pro-tein complexes activate lymphocytes is unknown. It may include an antigen-independent pathway by crosslinking thiols of cell-surface molecules, which results in the activation of a tyrosine kinase; antigen-dependent activation via a cell-mediated delayed-type IV hypersensitivity involving activation of sensitized T lymphocytes; non-specific polyclonal activation by cross-linking lymphocyte receptors without the presence of an antigen presenting cell.
Currently, there are no reliable standardized predictive tests for metal allergy and hypersensitivity (Shimmin et al. Citation2008). A new technique to evaluate patients with possible delayed hypersensitivity through the use of a triple assay technique for blood has been described (Hallab et al. Citation2000). This technique includes (1) a proliferation assay of white blood cells, (2) a cytokine analysis using enzyme-linked immunosorbent assay, and (3) a migration inhibition assay. The latter assay identifies a migration inhibition factor, which was one of the first cytokines found to be associated with delayed-type hypersensitivity reactions. The metal-induced sensitivity is quantified by comparing the results from all three assays.
Effects of metal particles/ions on bone cells
The biological effects of metallic particles on cells relevant to bone, osteoblasts, and osteoclasts, have not been fully elucidated. In addition to the direct effects of metal particles on cells, it is likely that there is autocrine and paracrine regulation of cytokines, which may contribute to bone resorption by influencing the functions of bone cells.
Osteoblasts
Metal ions can influence the biology of osteoblasts. It has been reported by several authors that exposure to metal ions can result in a dysregulation of osteoblasts and osteoblast-like cells. Osteoblasts exposed to Co and Cr ions undergo a dose-depen-dent reduction in proliferation. Although the metal ion concentrations within bone in vivo has not been established, exposure of osteoblasts to Co particles at concentrations of 100 µg/L or higher leads to the development of cytoplasmic vacuolations (Allen et al. Citation1997, Anissian et al. Citation2002, Hallab et al. Citation2002). Moreover, Co and Cr ions are toxic for osteoblasts, leading to markedly reduced alkaline phosphatase activity (McKay et al. Citation1996, Fleury et al. Citation2006). Co and Cr ions induce oxidation and nitration of proteins and dysregulation of the expression of antioxidant enzymes (Fleury et al. Citation2006). The cells are capable of releasing proinflammatory cytokines into the microenvironment, such as IL-6 and TNF-α (Hallab et al. Citation2001, Anissian et al. Citation2002, Hallab et al. Citation2002). These cytokines can in turn activate the differentiation of preosteoclasts into mature bone-resorbing cells (Kudo et al. Citation2003). A decrease in protein, DNA, and RNA synthesis has been shown with Co and Cr ions (Hodges and Chipman Citation2002). Collagen type I synthesis is markedly reduced after exposure to Co and Cr particles or ions at concentrations of 10 µg/L or 100 µg/L (Wang et al. Citation1997, Hallab et al. Citation2001, Anissian et al. Citation2002). Proteins from the extracellular matrix such as bone sialoprotein, osteocalcin, and osteopontin are important for calcification of the bone matrix (Robey and Boskey Citation2006). These proteins are synthesized by osteoblasts and released into the extracellular matrix. Co and Cr inhibit the release of osteocalcin into the bone matrix, contributing to the delay in mineralization of bone tissue following exposure to metal (Morais et al. Citation1998, Fernandes Citation1999).
Osteoclasts
MacQuarrie et al. (Citation2004) have demonstrated that the mechanism by which Co and Cr particles induce osteoclastogenesis is via a RANKL-depen-dent mechanism. The authors found a reduction in numbers of these cells after treatment with anti-RANKL antibodies. However, the authors used particles close to the size of polyethylene debris, which is capable of inducing a macrophage response. The effects of metal ions also appear to be dependent on the state of differentiation of the cells. Nichols and Puleo (Citation1997) investigated the effects of Co and Cr ions on the generation of osteoclasts by co-culturing osteoblast cells with bone marrow cells. They showed that Co ions induced the death of osteoclast precursors after 2 weeks of co-culture, at a concentration of 0.1 mg/ L (equivalent to 1.6 µmol/L). Furthermore, there was a decrease in the area of resorbed dentine after 3 weeks, indicating a toxic effect of Co on bone marrow osteoclast precursors.
The effects of metal ions on mature osteoclasts isolated from the long bone of rabbits have been investigated (Rousselle et al. Citation2002). The study showed that Co ions at a concentration of 104 μg/L (∼170 µmol/L) and Cr ions at a concentration of 104 μg/L (∼200 µmol/L) did not induce apoptosis of mature osteoclasts. After 4 days of culture with Co ions, however, a decrease in the size of the mature osteoclasts was observed. As there was no change in the overall percentage of eroded surface, the authors suggested that Co induced a decrease in the size of the pits (which was correlated to the reduction in the size of the osteoclasts).
Metal ions in cells
Metal transport.
Albumin is the major carrier protein in the blood, and is capable of binding Co2+. A sequence of 4 amino acids (N-Asp-Ala-His-Lys) is indispensable for this binding (Bar-Or et al. Citation2001). In serum, Cr3+ can also bind to albumin, but it is still unclear whether this sequence of 4 amino acids plays a role in the uptake of Cr (Borguet et al. Citation1995). Metal ions are also known to bind ferritin (Torti and Torti Citation1994). Co and Cr ions can also bind to transferrins, which are a family of large non-heme iron-binding glycoproteins with a molecular weight of 80 kDa involved in the iron uptake (Sun et al. Citation1999, Qian et al. Citation2002).
Microparticles of Co and Cr can be phagocytosed by cells. In the phagolysosome, the particles are exposed to a series of oxidative mechanisms designed to destroy the foreign body, which leads to the generation of metal ions and free radicals (reactive oxygen species and reactive nitrogen species) (Galle et al. Citation1992, Lundborg et al. Citation1992). However, it is still unclear how metal ions can be released from the lysosome. It has been suggested that nanoparticles may be taken into the cells by pinocytosis, a well-characterized pathway used by the cells to absorb nutrients. Corrosion is the main degradation process of metals; it leads to the generation of metal ions of different valencies. Metals become protected against corrosion by the formation of an oxide layer at the surface. However, under certain conditions the oxide layer can be removed, allowing the surface of the metal to come into direct contact with the corrosive compounds of the biological fluids (Mabilleau et al. Citation2006). Metal ions may also enter cells directly by different pathways, such as using an ion transporter or using specific metal ion binding proteins ().
Different pathways used by metal ions to enter into the cells. Cr(VI) can cross the cytoplasmic membrane through the non-specific phosphate/sulphate anionic transporters. Within the cell, Cr(VI) undergoes rapid metabolic reduction to form Cr(V), Cr(IV), and finally Cr(III). At each step of Cr(VI) reduction, reactive oxygen species (ROS) and reactive nitrogen species (RNS) are generated. Whether or not Cr(III) is able to cross the cell membrane on its own without a carrier remains controversial. Co(II) can cross the cell membrane using the non-specific iron transporter and it is oxidized in the cytosol to Co(III). Co-oxidation leads to the generation of ROS and RNS. Co(II) and Cr(VI–III) can also bind some metal-binding proteins such as transferrin or ferritin. In the endosome, Co(II) and Cr(VI–III) are unbound from their protein carrier; via the DMT1 transporter, they are released in the cytosol where they can be reduced/ oxidized and result in the generation of ROS/RNS. ROS and RNS are known to be involved in protein oxidation, leading to their degradation, lipid peroxidation, and DNA damage. Cr(III) can also cross the nuclear membrane and participate in alteration of DNA. Metal particles, released from the articulating surface of the MoM prosthesis, can be phagocytosed by the cells. Inside the phago-lysosome, particles become corroded and release metal ions in the cytosol.
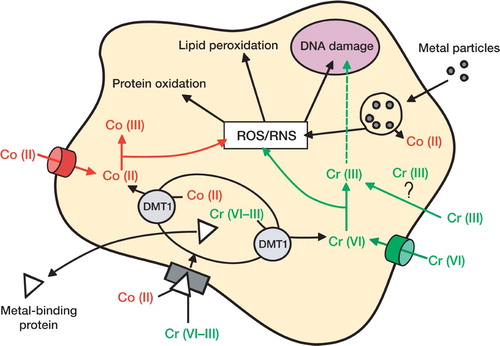
Under physiological conditions, Cr ions exist as chromate oxyanions (Cr6+) and cross the cell membrane by non-specific phosphate/sulfate anionic transporters (Buttner and Beyersmann Citation1985, Arslan et al. Citation1987). Within the cell, Cr6+undergoes rapid metabolic reduction by ascorbic acid and low-molecular-weight thiols, including reduced glutathione and cysteine. Although Cr3+ is known to exist, it is still unclear how it can cross the cell membrane. It has not been established whether Co2+ enters mammalian cells via a specific transporter. In primitive organisms (bacteria, yeast) there are some specific transporters for the uptake and release of Co ions (Eitinger et al. Citation2005, Eshaghi et al. Citation2006). Such systems have not yet been shown to exist in mammalian cells. It has, however, been shown that P2X7 transporter is involved in uptake of divalent cations and that it is a transporter for Co uptake (Virginio et al. Citation1997).
Metal-induced intracellular effects.
Reactions with metal ions can lead to generation of free radicals: reactive oxygen species (ROS) and reactive nitrogen species (RNS), which can, in turn, cause cellular dysfunction. Inside the cells, Cr6+ is oxidized to Cr3+ in a series of steps that generate free radicals. On the other hand, Co ions can participate in a Fenton-like reaction with hydrogen peroxide, leading to the generation of free radicals. Free radicals can react with DNA (both nuclear and mitochondrial) and induce damage to purine and pyrimidine bases as well as to the deoxyribose backbone (Dizdaroglu et al. Citation2002). They can also induce crosslinks in DNA. Free radicals catalyze the oxidation of protein and phospholipids (a process known as lipid peroxidation). Lipid peroxidation has 3 stages: initiation, propagation, and termination, and it leads to the formation of malondialdehyde, which can react with DNA bases to form DNA adducts, leading to the formation of DNA-DNA inter-strand crosslinks or DNA-protein crosslinks (Bacon et al. Citation1983, Marnett Citation1999). Permanent modification of genetic material resulting from this “oxidative damage” represents the first step in mutagenesis, carcinogenesis, and ageing. Although no evidence has been found for direct binding of Co2+ to DNA, direct binding of Cr3+ to DNA is well documented (Wolf et al. Citation1989). In cells, 2 main processes exist to correct DNA aberrations and to restore the integrity of the genome: base excision repair (BER) and nucleotide excision repair (NER) (Hartwig et al. Citation2003). Under stimulation by Co2+ or Cr6+, both of these repair mechanisms are inhibited (Witkiewicz-Kucharc-zyk and Bal 2006). Ladon et al. (Citation2004) investigated changes in metal ion levels and chromosome aberrations in patients within 2 years of receiving MoM hip arthroplasties. The authors noted an increase in chromosome translocations and aneuploidy in peripheral blood lymphocytes at 6, 12, and 24 months after surgery. The authors also noted that these changes were progressive. However, the authors did not find any statistically significant correlations between chromosomal translocation indices and Co or Cr concentrations in whole blood.
Conclusion
Although elevated levels of metal ions in the synovial fluid and in the peripheral blood after MoM hip resurfacing arthroplasty are a common finding, the importance of this observation remains unclear. The adverse biological responses to the metal ions produced can be local or systemic. Clinical cases of local periprosthetic soft- tissue reactions and osteolysis are being increasingly reported in the literature.
The precise biological pathway that leads to adverse periprosthetic soft-tissue effects of metallic particles remains unknown. The high concentrations of metal particles confined within the effective joint space may lead to activation of T lympho-cyte-mediated hypersensitivity reactions of type IV (delayed-type hypersensitivity). In turn, this may affect other local cell populations, including osteoclasts and osteoblasts. The individual biological response to the presence of wear debris appears to vary. This may reflect a different toxic-effect threshold or immunological intolerance. In some susceptible patients, the T cell-mediated cellular interplay between metal nanoparticles and immune cells may lead to adverse clinical outcomes such as painful soft-tissue masses or radiographic appearance of osteolysis.
As the metal-on-metal hip resurfacing procedures are being increasingly used in younger active patients whose life expectancy is considerably longer than that of elderly patients who traditionally receive hip replacements, the concerns about the unknown risks of long-term exposure to metal debris remain. As the latency time of the metal-on-metal hip resurfacing could be as long as 20 years, one can speculate that there may be development of tumors in response to excessive metal particles and metal ion production in these cases. This highlights the importance of long-term clinical follow-up and cellular research into the biological mechanisms involved in the pathogenesis of metal wear particle-induced adverse periprosthetic biological reactions.
This work was made possible by grants from the Furlong Research Charitable Foundation.
No competing interests declared.
- Allen M J, et al. The effects of particulate cobalt, chromium and cobalt-chromium alloy on human osteoblast-like cells in vitro. J Bone Joint Surg (Br) 1997; 79: 475–82
- Amstutz H C, et al. Resurfacing THA for patients younger than 50 year: results of 2- to 9-year followup. Clin Orthop 2007, 460: 159–64
- Anissian L, et al. Cobalt ions influence proliferation and function of human osteoblast-like cells. Acta Orthop Scand 2002; 73: 369–74
- Arslan P, et al. Intracellular chromium reduction. Biochim Biophys Acta 1987; 931: 10–5
- Back D L, et al. Early results of primary Birmingham hip resurfacings. An independent prospective study of the first 230 hips. J Bone Joint Surg (Br) 2005a; 87: 324–9
- Back D L, et al. How do serum cobalt and chromium levels change after metal-on-metal hip resurfacing?. Clin Orthop 2005b, 438: 177–81
- Bacon B R, et al. Hepatic lipid peroxidation in vivo in rats with chronic iron overload. J Clin Invest 1983; 71: 429–39
- Bar-Or D, et al. Characterization of the Co(2+) and Ni(2+) binding amino-acid residues of the N-terminus of human albumin. An insight into the mechanism of a new assay for myocardial ischemia. Eur J Biochem 2001; 268: 42–7
- Beaule P E, et al. Risk factors affecting outcome of metal-on-metal surface arthroplasty of the hip. Clin Orthop 2004a; 87–93, [no.??)]:
- Beaule P E, et al. Metal-on-metal surface arthroplasty with a cemented femoral component: a 7-10 year follow-up study. J Arthroplasty s2004b; 19: 17–22
- Bell R S, et al. A study of implant failure in the Wagner resurfacing arthroplasty. J Bone Joint Surg (Am) 1985; 67: 1165–75
- Boardman D R, et al. A benign psoas mass following metal-on-metal resurfacing of the hip. J Bone Joint Surg (Br) 2006; 88: 402–4
- Borguet F, et al. Study of the chromium binding in plasma of patients on continuous ambulatory peritoneal dialysis. Clin Chim Acta 1995; 238: 71–84
- Brodner W, et al. Serum cobalt levels after metal-on-metal total hip arthroplasty. J Bone Joint Surg (Am) 2003; 85: 2168–73
- Brodner W, et al. Does the placenta inhibit the passage of chromium and cobalt after metal-on-metal total hip arthroplasty?. J Arthroplasty 2004a; 19: 102–6
- Brodner W, et al. Cup inclination and serum concentration of cobalt and chromium after metal-on-metal total hip arthroplasty. J Arthroplasty 2004b; 19: 66–70
- Buergi M L, Walter W L. Hip resurfacing arthroplasty: the Australian experience. J Arthroplasty 2007; 22: 61–5
- Buschner G, et al. Subsurface microstructure of metal-on-metal hip joints and its relationship to wear particle generation. J Biomed Mater Res 2004; 72B: 206–14
- Buttner B, Beyersmann D. Modification of the erythrocyte anion carrier by chromate. Xenobiotica 1985; 15: 735–41
- Campbell P, et al. Isolation of predominantly submicron-sized UHMWPE wear particles from periprosthetic tissues. J Biomed Mater Res 1995; 29: 127–31
- Campbell P, et al. Wear and morphology of ultra-high molec ular weight polyethylene wear particles from total hip replacements. Proc Inst Mech Eng [H] 1996; 210: 167–74
- Case C P, et al. Preliminary observations on possible premalignant changes in bone marrow adjacent to worn total hip arthroplasty implants. Clin Orthop 1996, 329: S269–79
- Clarke M T, et al. Levels of metal ions after small- and large-diameter metal-on-metal hip arthroplasty. J Bone Joint Surg (Br) 2003; 85: 913–7
- Clodfelder B J, et al. The trail of chromium(III) in vivo from the blood to the urine: the roles of transferrin and chromodulin. J Biol Inorg Chem 2001; 6: 608–17
- Cobb A G, Schmalzreid T P. The clinical significance of metal ion release from cobalt-chromium metal-on-metal hip joint arthroplasty. Proc Inst Mech Eng [H] 2006; 220: 385–98
- Coleman R F, et al. Concentration of wear products in hair, blood, and urine after total hip replacement. Br Med J 1973; 1: 527–9
- Daniel J, et al. Metal-on-metal resurfacing of the hip in patients under the age of 55 years with osteoarthritis. J Bone Joint Surg (Br) 2004; 86: 177–84
- Daniel J, et al. The effect of the diameter of metal-on-metal bearings on systemic exposure to cobalt and chromium. J Bone Joint Surg (Br) 2006; 88: 443–8
- Daniel J, et al. The validity of serum levels as a surrogate measure of systemic exposure to metal ions in hip replacement. J Bone Joint Surg (Br) 2007; 89: 736–41
- Davies A P, et al. An unusual lymphocytic perivascular infiltration in tissues around contemporary metal-on-metal joint replacements. J Bone Joint Surg (Am) 2005; 87: 18–27
- De Smet K A. Belgium experience with metal-on-metal surface arthroplasty. Orthop Clin North Am 2005; 36: 20313, ix
- Dizdaroglu M, et al. Free radical-induced damage to DNA: mechanisms and measurement. Free Radic Biol Med 2002; 32: 1102–15
- Doherty A T, et al. Increased chromosome translocations and aneuploidy in peripheral blood lymphocytes of patients having revision arthroplasty of the hip. J Bone Joint Surg (Br) 2001; 83: 1075–81
- Doorn P F, et al. Tissue reaction to metal on metal total hip prostheses. Clin Orthop 1996, 329: S187–205
- Doorn P F, et al. Metal wear particle characterization from metal on metal total hip replacements: transmission electron microscopy study of periprosthetic tissues and isolated particles. J Biomed Mater Res 1998; 42: 103–11
- Dumbleton J H, et al. A literature review of the association between wear rate and osteolysis in total hip arthroplasty. J Arthroplasty 2002; 17: 649–61
- Eitinger T, et al. Secondary transporters for nickel and cobalt ions: theme and variations. Biometals 2005; 18: 399–405
- Eshaghi S, et al. Crystal structure of a divalent metal ion transporter CorA at 2.9 angstrom resolution. Science 2006; 313: 354–7
- Fernandes M H. Effect of stainless steel corrosion products on in vitro biomineralization. J Biomater Appl 1999; 14: 113–68
- Fleury C, et al. Effect of cobalt and chromium ions on human MG-63 osteoblasts in vitro: morphology, cytotoxicity, and oxidative stress. Biomaterials 2006; 27: 3351–60
- Galle P, et al. Role of alveolar macrophages in precipitation of mineral elements inhaled as soluble aerosols. Environ Health Perspect 1992; 97: 145–7
- Gillespie W J, et al. Development of hematopoietic cancers after implantation of total joint replacement. Clin Orthop 1996, 329: S290–6
- Goodman S B, et al. In vitro, in vivo, and tissue retrieval studies on particulate debris. Clin Orthop 1998, 352: 25–34
- Green T R, et al. Polyethylene particles of a ‘critical size’ are necessary for the induction of cytokines by macrophages in vitro. Biomaterials 1998; 19: 2297–302
- Grigoris P, et al. The evolution of hip resurfacing arthroplasty. Orthop Clin North Am 2005; 36: 125–34, vii
- Gruber F W, et al. Cystic lesion of the groin due to metallosis: a rare long-term complication of metal-on-metal total hip arthroplasty. J Arthroplasty 2007; 22: 923–7
- Hallab N J, et al. A triple assay technique for the evaluation of metal-induced, delayed-type hypersensitivity responses in patients with or receiving total joint arthroplasty. J Biomed Mater Res 2000; 53: 480–9
- Hallab N, et al. Metal sensitivity in patients with orthopaedic implants. J Bone Joint Surg (Am) 2001; 83: 428–36
- Hallab N J, et al. Concentration- and composition-depen-dent effects of metal ions on human MG-63 osteoblasts. J Biomed Mater Res 2002; 60: 420–33
- Hallab N J, et al. Lymphocyte responses in patients with total hip arthroplasty. J Orthop Res 2005; 23: 384–91
- Harris W H. The problem is osteolysis. Clin Orthop 1995, 311: 46–53
- Hart A J, et al. The association between metal ions from hip resurfacing and reduced T-cell counts. J Bone Joint Surg (Br) 2006; 88: 449–54
- Hartwig A, et al. Modulation of DNA repair processes by arsenic and selenium compounds. Toxicology 2003; 193: 161–9
- Head W C. Wagner surface replacement arthroplasty of the hip. Analysis of fourteen failures in forty-one hips. J Bone Joint Surg (Am) 1981; 63: 420–7
- Heisel C, et al. The relationship between activity and ions in patients with metal-on-metal bearing hip prostheses. J Bone Joint Surg (Am) 2005; 87: 781–7
- Hing C B, et al. The results of primary Birmingham hip resurfacings at a mean of five years: An independent prospective review of the first 230 hips. J Bone Joint Surg (Br) 2007; 89: 1431–8
- Hodges N J, Chipman J K. Down-regulation of the DNA-repair endonuclease 8-oxo-guanine DNA glycosylase 1 (hOGG1) by sodium dichromate in cultured human A549 lung carcinoma cells. Carcinogenesis 2002; 23: 55–60
- Howie D W, Vernon-Roberts B. Long-term effects of intraarticular cobalt-chrome alloy wear particles in rats. J Arthroplasty 1988; 3: 327–36
- Howie D W, et al. The long-term wear of retrieved McKee-Farrar metal-on-metal total hip prostheses. J Arthroplasty 2005; 20: 350–7
- Jacobs J J, et al. Cobalt and chromium concentrations in patients with metal on metal total hip replacements. Clin Orthop 1996, 329: S256–63
- Jacobs J J, et al. Metal release in patients who have had a primary total hip arthroplasty. A prospective, controlled, longitudinal study. J Bone Joint Surg (Am) 1998; 80: 1447–58
- Jakobsen S S, et al. Cobalt-chromium-molybdenum alloy causes metal accumulation and metallothionein up-regu-lation in rat liver and kidney. Basic Clin Pharmacol Toxicol 2007; 101: 441–6
- Kadoya Y, et al. Wear and osteolysis in total joint replacements. Acta Orthop Scand 1998, Suppl 278: 1–16
- Keegan G M, et al. Orthopaedic metals and their potential toxicity in the arthroplasty patient: A review of current knowledge and future strategies. J Bone Joint Surg (Br) 2007; 89: 567–73
- Kobayashi A, et al. The size and shape of particulate polyethylene wear debris in total joint replacements. Proc Inst Mech Eng [H] 1997a; 211: 11–5
- Kobayashi A, et al. Number of polyethylene particles and osteolysis in total joint replacements. A quantitative study using a tissue-digestion method. J Bone Joint Surg (Br) 1997b; 79: 844–8
- Kudo O, et al. Interleukin-6 and interleukin-11 support human osteoclast formation by a RANKL-independent mechanism. Bone 2003; 32: 1–7
- Ladon D, et al. Changes in metal levels and chromosome aberrations in the peripheral blood of patients after metal-on-metal hip arthroplasty. J Arthroplasty 2004; 19: 78–83
- Lhotka C, et al. Four-year study of cobalt and chromium blood levels in patients managed with two different metal-on-metal total hip replacements. J Orthop Res 2003; 21: 189–95
- Lidgren L. Chronic inflammation, joint replacement and malignant lymphoma. J Bone Joint Surg (Br) 2008; 90: 7–10
- Lilikakis A K, et al. Hydroxyapatite-coated femoral implant in metal-on-metal resurfacing hip arthroplasty: minimum of two years follow-up. Orthop Clin North Am 2005; 36: 215–22, ix
- Lundborg M, et al. Phagolysosomal pH and dissolution of cobalt oxide particles by alveolar macrophages. Environ Health Perspect 1992; 97: 153–7
- Mabilleau G, et al. Influence of fluoride, hydrogen peroxide and lactic acid on the corrosion resistance of commercially pure titanium. Acta Biomater 2006; 2: 121–9
- MacDonald S J, et al. Metal-on-metal versus polyethylene in hip arthroplasty: a randomized clinical trial. Clin Orthop 2003, 406: 282–96
- MacQuarrie R A, et al. Wear-particle-induced osteoclast osteolysis: the role of particulates and mechanical strain. J Biomed Mater Res B Appl Biomater 2004; 69: 104–12
- Madan S, et al. Recurrent intrapelvic cyst complicating metal-on-metal cemented total hip arthroplasty. Arch Orthop Trauma Surg 2000; 120: 508–10
- Maloney W J, et al. Fixation, polyethylene wear, and pelvic osteolysis in primary total hip replacement. Clin Orthop 1999, 369: 157–64
- Margevicius K J, et al. Isolation and characterization of debris in membranes around total joint prostheses. J Bone Joint Surg (Am) 1994; 76: 1664–75
- Marker M, et al. Metal-on-metal hip implants: do they impair renal function in the long-term? A 10-year follow-up study. Arch Orthop Trauma Surg 2007; 128: 915–9
- Marnett L J. Lipid peroxidation-DNA damage by malondialdehyde. Mutat Res 1999; 424: 83–95
- McKay G C, et al. Interactions of orthopaedic metals with an immortalized rat osteoblast cell line. Biomaterials 1996; 17: 1339–44
- McKellop H, et al. In vivo wear of three types of metal on metal hip prostheses during two decades of use. Clin Orthop 1996, 329: S128–40
- Mont M A, et al. Resurfacing hip arthroplasty: comparison of a minimally invasive versus standard approach. Clin Orthop Relat Res 2005; 441: 125–31
- Mont M A, et al. Use of metal-on-metal total hip resurfacing for the treatment of osteonecrosis of the femoral head. J Bone Joint Surg (Am) 2006; 88(Suppl 3)90–7
- Morais S, et al. In vitro biomineralization by osteoblast-like cells. I. Retardation of tissue mineralization by metal salts. Biomaterials 1998; 19: 13–21
- National joint registry England and Wales annual report. http:www.njrventre.org.uk/Public/prev_annual_reports.htm 2008
- Nichols K G, Puleo D A. Effect of metal ions on the formation and function of osteoclastic cells in vitro. J Biomed Mater Res 1997; 35: 265–71
- Nishii T, et al. Five-year results of metal-on-metal resurfacing arthroplasty in Asian patients. J Arthroplasty 2007; 22: 176–83
- The Norwegian Arthroplasty Register. http://www.hauke-land.no/nrl 2007
- Pandit H, et al. Pseudotumours associated with metal-on- metal hip resurfacings. J Bone Joint Surg (Br) 2008; 90: 847–51
- Papageorgiou I, et al. The effect of nano- and micron-sized particles of cobalt-chromium alloy on human fibroblasts in vitro. Biomaterials 2007; 28: 2946–58
- Park Y S, et al. Early osteolysis following second-generation metal-on-metal hip replacement. J Bone Joint Surg (Am) 2005; 87: 1515–21
- Qian Z M, et al. Targeted drug delivery via the transferrin receptor-mediated endocytosis pathway. Pharmacol Rev 2002; 54: 561–87
- Rae T. The macrophage response to implant materials. Crit Rev Biocompatibility 1986; 2: 97–126
- Rasquinha V J, et al. Serum metal levels and bearing surfaces in total hip arthroplasty. J Arthroplasty 2006; 21: 47–52
- Revell M P, et al. Metal-on-metal hip resurfacing in osteonecrosis of the femoral head. J Bone Joint Surg (Am) 2006; 88(Suppl 3)98–103
- Robey P, Boskey A. Extracellular matrix and biomineralization. Primer on the metabolic bone diseases and disorders of mineral metabolism, J Lian, S Goldring. American Society for Bone and Mineral Research, Washington DC 2006; 12–9
- Rousselle A V, et al. Influence of metal ion solutions on rabbit osteoclast activities in vitro. Histol Histopathol 2002; 17: 1025–32
- Savarino L, et al. Ion release in patients with metal-on-metal hip bearings in total joint replacement: a comparison with metal-on-polyethylene bearings. J Biomed Mater Res 2002; 63: 467–74
- Schmalzried T P, et al. Periprosthetic bone loss in total hip arthroplasty. Polyethylene wear debris and the concept of the effective joint space. J Bone Joint Surg (Am) 1992; 74: 849–63
- Shanbhag A S, et al. Composition and morphology of wear debris in failed uncemented total hip replacement. J Bone Joint Surg (Br) 1994; 76: 60–7
- Shimmin A, et al. Metal-on-metal hip resurfacing arthroplasty. J Bone Joint Surg (Am) 2008; 90: 637–54
- Shimmin A J, Back D. Femoral neck fractures following Birmingham hip resurfacing: a national review of 50 cases. J Bone Joint Surg (Br) 2005; 87: 463–4
- Shimmin A J, et al. Complications associated with hip resurfacing arthroplasty. Orthop Clin North Am 2005; 36: 18793, ix
- Sieber H P, et al. Analysis of 118 second-generation metal-on-metal retrieved hip implants. J Bone Joint Surg (Br) 1999; 81: 46–50
- Sochart D H. Relationship of acetabular wear to osteolysis and loosening in total hip arthroplasty. Clin Orthop 1999, 363: 135–50
- Steffen R T, et al. The five-year results of the Birmingham Hip Resurfacing arthroplasty: An independent series. J Bone Joint Surg (Br) 2008; 90: 436–41
- Sun H, et al. Transferrin as a metal ion mediator. Chem Rev 1999; 99: 2817–42
- Swedish Hip Arthroplasty Register. http://www.jru.orthop.gu.se 2006
- Toms A P, et al. MRI of early symptomatic metal-on-metal total hip arthroplasty: a retrospective review of radiological findings in 20 hips. Clin Radiol 2008; 63: 49–58
- Torti S V, Torti F M. Iron and ferritin in inflammation and cancer. Adv Inorg Biochem 1994; 10: 119–37
- Treacy R B, et al. Birmingham hip resurfacing arthroplasty. A minimum follow-up of five years. J Bone Joint Surg (Br) 2005; 87: 167–70
- Vendittoli P A, et al. Chromium and cobalt ion release following the Durom high carbon content, forged metal-on-metal surface replacement of the hip. J Bone Joint Surg (Br) 2007; 89: 441–8
- Virginio C, et al. Effects of divalent cations, protons and calmidazolium at the rat P2X7 receptor. Neuropharmacology 1997; 36: 1285–94
- Wang J Y, et al. Prosthetic metals impair murine immune response and cytokine release in vivo and in vitro. J Orthop Res 1997; 15: 688–99
- Willert H G, et al. Metal-on-metal bearings and hypersensitivity in patients with artificial hip joints. A clinical and histomorphological study. J Bone Joint Surg (Am) 2005; 87: 28–36
- Witkiewicz-Kucharczyk A, Bal W. Damage of zinc fingers in DNA repair proteins, a novel molecular mechanism in carcinogenesis. Toxicol Lett 2006; 162: 29–42
- Witzleb W C, et al. Neo-capsule tissue reactions in metal-on-metal hip arthroplasty. Acta Orthop 2007; 78: 211–20
- Wolf T, et al. Molecular interaction of different chromium species with nucleotides and nucleic acids. Carcinogenesis 1989; 10: 655–9
- Ziaee H, et al. Transplacental transfer of cobalt and chro mium in patients with metal-on-metal hip arthroplasty: a controlled study. J Bone Joint Surg (Br) 2007; 89: 301–5