Abstract
Hexagonal β-CuSCN hollow microspheres with average diameter 5 µm were successfully synthesised on a large scale via a decomposed reaction of the Cu(II)-dithizonate complex (Cu(HDZ)2). Examination of the produced microspheres as a function of the reaction time showed that the surface topography of the microspheres changed from a ragged surface to a smooth one. The synthetic products have been characterised by X-ray powder diffraction patterns, microscopy image and scanning electron microscopy. The optical properties were also investigated by the room temperature ultraviolet–visible absorption spectroscopy. Then, based on the observation, a possible formation process of hollow microspheres was proposed.
Keywords:
1. Introduction
Recently, one of the major technological challenges in materials research is the self-assembly of tiny nanobuilding units into larger geometrical architectures because control of the nucleation and growth of nanomaterials is difficult Citation1. Self-assembled microstructures have been attracting growing interest because of their highly specific morphologies, novel properties and great application potential in many fields Citation2. Among the resulting specific architectures, hollow structures with nanometre to micrometre dimensions, due to their high surface area, low material density and surface permeability Citation3, are highly demanded for applications in optical, electronic, magnetic, catalytic and sensoring devices Citation4–8. Therefore, it is attractive to further explore this field, which has been an intensive and hot research topic.
It is seen that β-CuSCN is a p-type semiconductor (band gap ∼3.6 eV), possessing three-dimensional polymer-like structures constituted by layers of close-packed CuSCN units Citation9. Therefore, it is thought to be the most promising substance in filling the pores of porous film due to its stability and possibility Citation10. Nowadays, β-CuSCN has been a good candidate material for the realisation of dye-sensitised solid-state photovoltaic cells Citation11–13 and it has been widely used as a window for solar cells Citation14, a catalyst for the synthesis of organic compounds Citation15, and a material for the synthesis of polymer electrolytes Citation16. So far, various routes have been reported to synthesise CuSCN, such as deposition technique Citation17, microcrystal growth technology Citation18, alumina template process Citation19 and solution reaction Citation20. However, the methods used for the synthesis of β-CuSCN nano and micropowders with special shapes are few in number. In this article, we present a facile method for the synthesis of β-CuSCN powder with the hollow spherical microstructure via a solvothermal treatment of dithizone and CuCl2 · 2H2O at a relatively low temperature. In this solvothermal process, neither physical templates nor catalysts are required. Then, based on microscope image and scanning electron microscopy (SEM), a possible formation mechanism of the hollow microstructures was proposed.
2. Experimental sections
2.1. Chemicals and synthesis
All the chemicals are analytical pure reagents (AR) and used without further treatment.
In a typical synthesis, 0.308 g of dithizone (1.2 mmol) was dissolved in 45 mL benzene under constant stirring to form a green solution (S1). In this method, 0.242 g of CuCl2 · 2H2O (1.4 mmol) was dissolved in appropriate amounts of distilled water and then transferred into the above solution (S1) to form a dark red solution (S2) after stirring for 30 min. Subsequently, the water phase was removed from S2 and the organic phase was collected and transferred into the 50 mL Teflon-lined stainless steel autoclave, up to 80% of its whole capacity. At last, the autoclave was sealed and maintained at 180°C for 24 h. After the reaction was finished, the autoclave was allowed to cool down to room temperature naturally. The grey-black precipitate was filtered and washed successively with distilled water and ethanol to remove the residual reactants. After that, the obtained sample was dried in vacuum at 60°C for 4 h and collected for characterisation.
2.2. Characterisation
X-ray powder diffraction (XRD) pattern of the product was performed with a D/max-2200 PC (Japan) X-ray diffractometer with Cu-Kα radiation (λ = 1.5418 Å). The surface morphology and the particle size of the as-prepared products were examined by environmental SEM (Quanta 200 Philips-FEI Co., Holland, 20 KV). FT-IR spectra was recorded on a Vector-22 Fourier Transmission Infrared spectrometer with wave numbers in the range 400–4000 cm−1. The ultraviolet–visible (UV–vis) absorption was recorded on a UV–vis spectrophotometer (DR 5000, Hach Company) with wavelength ranging from 190 to 1100 nm.
3. Results and discussion
The phase structure and the purity of the as-prepared products were characterised by XRD. shows the typical XRD pattern of the as-prepared β-CuSCN sample with the hollow spherical microstructure. All the diffraction peaks in the pattern can be indexed as the pure hexagonal phase of β-CuSCN with the lattice constants a = 3.857 and c = 16.449 Å (JCPDS card No. 29-0581). No characteristic XRD peaks arising from possible impurities, such as sulphur, metallic oxides or any other sulphides can be detected, indicating that the obtained products are pure.
The morphology and the particle size of β-CuSCN were investigated by the microscopic and the SEM images. The overall morphology of the samples, as shown in , indicates that there exist a great deal of relatively uniform microspheres with average diameter 5 µm. Some hollow microspheres are broken when ultrasonication was applied (). The fragments of the cracked one are present in . The cracked hollow sphere has a wall thickness of 1 µm, which proves its hollow structure. shows the microscopic image of the uniform hollow spheres, which are in agreement with the above SEM results. The inner cavity of the microspheres is clearly revealed by the unchanged contrast difference between the centre and edge, further confirming their hollow structures.
Figure 2. (Colour online) (a) Low-magnification SEM image of the sample; (b) high-magnification SEM image of some fragments of hollow spheres; (c) high-magnification SEM image of a typical individual broken sphere; and (d) the microscopic image (×1000).
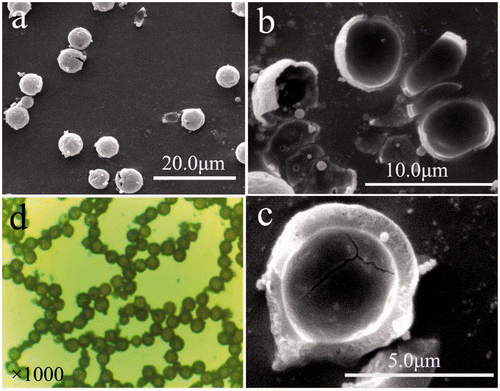
It is well known that dithizone (H2DZ) is a good linker ligand and a reducing agent, which can form M(HDZ)2 with M2+ under some conditions. Therefore, the Cu(HDZ)2 complex can be formulated as Citation21:
To confirm the formation of the Cu-dithizonate complex, the S2 solution was extracted and purified Citation22. Then, it was characterised by FT-IR spectrum ().
Figure 3. The FT-IR spectrum of the dithizone and the complex: (a) the dithizone and (b) the as-prepared complex.
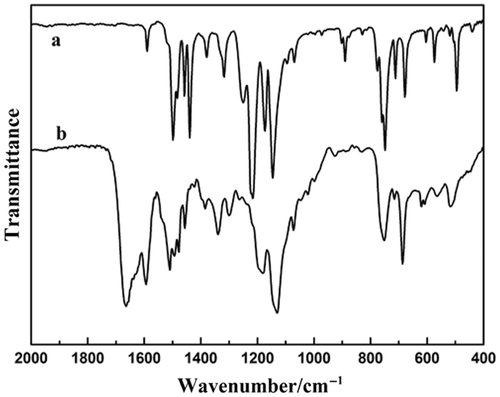
From , it is found that there are some changes ranging from 1100 to 1600 cm−1 between the as-prepared complex () and the pure dithizone (). Obviously, compared to the complex, the stretching vibration frequency of C=S of the pure dithizone shifted extremely from 1216 and 1146 cm−1 to 1179 and 1130 cm−1, while the strong absorption peaks of N–C at 1379 and 1317 cm−1 in the benzene ring of the dithizone were shifted to 1334 and 1298 cm−1. The decreased C=S stretching vibration frequencies imply that the dithizone coordinates with metal ion using its sulphur atom. This result is well in agreement with that reported Citation23.
The reaction in the present system is based on the formation of the Cu(HDZ)2 complex followed by thermal decomposition of these complexes to obtain the final product. The whole reaction process can also be expressed as:
To understand the formation mechanism of the spherical structures, we carried out the time-dependent experiments. The intermediate products at different reaction periods were used as the samples for the SEM characterisation () to track the formation of the hollow microspheres. In the initial stage of the intermediate products collected after 5 h, an examination shows that there are a large number of small particles. These small particles are interconnected to form the irregular and larger hollow particles (). With an increase in the reaction time (8 h), the sphere-like structure is gradually formed. But the surfaces of these spheres are not smooth and there are some caves and irregular small particles on them. Interestingly, some broken spheres were also found in . These intermediates suggest that the hollow spherical structures have already appeared in this stage (). By further prolonging the reaction time to 11 h (), we find that the nanoparticles on the surfaces almost disappear and the surfaces become smoother. The caves on the surfaces have been stuffed further by the particles and become small. As the solvothermal process is proceeding to 24 h, the hollow microspheres formed. Their surfaces are smooth and the caves disappear ().
Figure 4. SEM images of β-CuSCN hollow spheres prepared at different reaction stages: (a) 5; (b) 8; (c) 11; and (d) 24 h.
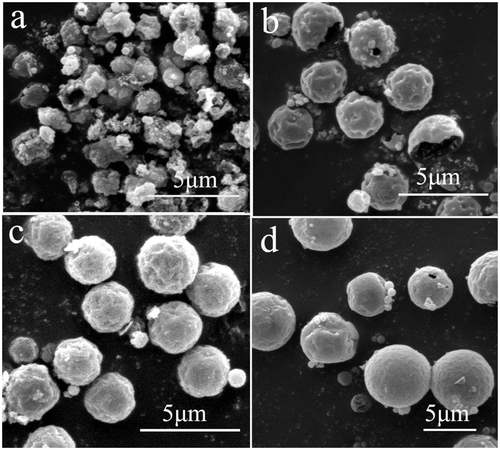
On the basis of the experiment results, the tentative formation mechanism of hollow spheres might be proposed. Similar to the fabrication of CuS core–shell structure Citation24, in this study, a small amount of water might play an important role in formatting hollow spheres. First, the Cu(HDZ)2 complex is formed and dissolved in benzene when the aqueous solution of copper ions is added into the benzene solution of dithizone. And the small amount of water remaining in the precursor solution might disperse and form small microspheres in the benzene–water mixture. When the temperature reaches 180°C, the Cu(HDZ)2 complex begins to decompose and form the CuSCN nanoparticles. Driven by the minimisation of interfacial energy, these nanoparticles may aggregate and self-assemble around the surfaces of the water. With the increment of the reaction time, the shells become thicker and denser through the oriented aggregation and their surfaces become smoother. Finally, the dispersed hollow spheres are obtained after the water is removed by washing and drying. One experimental fact described below seems to support such a formation mechanism. After the solvent was evaporated from the S2 solution under reduced pressure, the as-made precursor was placed in a vacuum oven at 60°C to remove the water further, and then the dehydrated precursor was dispersed in benzene under similar conditions established for CuSCN microspheres. The results show that only some particles appear although the pure hexagonal CuSCN is formed. Obviously, the existence of a small amount of water in the precursor is crucial for the formation of the hollow CuSCN micorspheres and the whole possible formation process might be simply depicted and described as in . In addition, we investigated the influence of temperature and solvent on the growth of CuSCN hollow spheres. We did the experiments using the typical synthesis route at different temperatures. It was found that almost no as-expected product could be found when the temperature was below 180°C, while at 180°C, CuSCN hollow spheres were easily obtained as the major products. The possible reason might be that dithizone could not decompose under a relatively low temperature. In our experiments, several solvents were also selected to synthesise CuSCN such as ethylendiamine and water, and no as-expected products appeared, which indicates that the solvent benzene is the ideal solvent for synthesis of the pure phase CuSCN.
The UV–vis absorption of hollow CuSCN spheres was also measured. shows the absorption spectrum of the sample dispersed in ethanol by sonication. A peak centred at 260 nm (4.1 eV) is observed. The absorption spectrum shows an obvious blue shift compared to that of bulk β-CuSCN (∼3.6 eV) Citation25.
4. Conclusions
In summary, we have successfully synthesised hollow CuSCN microspheres from a Cu(HDZ)2 precursor. The results show that the key factor to the formation of the hollow microspheres is the small amount of water remaining in the precursor solution and a possible growing mechanism of the hollow β-CuSCN microspheres has been proposed. It is known that dithizone is often used as a ligand to coordinate with some metal ions for preparing metal sulphide materials. However, in this route, only CuSCN could be obtained via a different thermal decomposition of the complex. It is expected that this method can be extended to prepare other hollow thiocyanate materials.
Acknowledgements
The authors appreciate the financial support from the Scientific Research Planning Program of the Education Department of Shaanxi Province (11JK0602), the Scientific Research Planning Program of Shaanxi Province of China (2011K07-13), the Scientific Research Planning Program of Yulin City and the Graduate Innovation Fund of Shaanxi University of Science and Technology.
This article was originally published with errors. This version has been corrected. P lease see Erratum (http://dx.doi.org/10.1080/17458080.2013.829704).
References
- Zhang , Z P , Shao , X Q , Yu , H D , Wang , Y B and Han , M Y . 2005 . Morphosynthesis and ornamentation of 3D dendritic nanoarchitectures . Chem. Mater. , 17 : 332 – 336 . doi: 10.1021/cm048436r
- Chen , L Y , Zhang , Z D and Wang , W Z . 2008 . Self-assembled porous 3D flowerlike -In2S3 structures: Synthesis, characterization, and optical properties . J. Phys. Chem. C , 112 : 4117 – 4123 . doi: 10.1021/jp710074h
- Jiang , P , Bertone , J F and Colvin , V L . 2001 . A lost-wax approach to monodisperse colloids and their crystals . Science , 291 : 453 – 457 . doi: 10.1126/science.291.5503.453
- Li , X P , Gao , Y N , Yu , L and Zheng , L Q . 2010 . Template-free synthesis of CdS hollow nanospheres based on an ionic liquid assisted hydrothermal process and their application in photocatalysis . J. Solid State Chem. , 183 : 1423 – 1432 . doi: 10.1016/j.jssc.2010.04.001
- Zhu , J and Qian , X F . 2010 . From 2-D CuO nanosheets to 3-D hollow nanospheres: interface-assisted synthesis, surface photovoltage properties and photocatalytic activity . J. Solid State Chem. , 183 : 1632 – 1639 . doi: 10.1016/j.jssc.2010.05.015
- Qu , X F , Yao , Q Z , Zhou , G T , Fu , S Q and Huang , J L . 2010 . Formation of hollow magnetite microspheres and their evolution into durian-like architectures . J. Phys. Chem. C , 114 : 8734 – 8740 . doi: 10.1021/jp912278r
- Liu , Q Y , Guo , X H , Li , Y and Shen , W J . 2009 . Synthesis of hollow co structures with netlike framework . Langmuir , 25 : 6425 – 6430 . doi: 10.1021/la900024e
- Weinzier , D , Lind , A and Kunz , W . 2009 . Hollow SiO2 microspheres produced by coating yeast cells . Cryst. Growth Des. , 9 : 2318 – 2323 . doi: 10.1021/cg801143e
- Gao , X D , Li , X M , Yu , W D , Qiu , J J and Gan , X Y . 2008 . Room-temperature deposition of nanocrystalline CuSCN film by the modified successive ionic layer adsorption and reaction method . Thin Solid Films , 517 : 554 – 559 . doi: 10.1016/j.tsf.2008.06.077
- Wu , W B , Jin , Z G , Hua , Z , Fu , Y N and Qiu , J J . 2005 . Growth mechanisms of CuSCN films electrodeposited on ITO in EDTA-chelated coppor(II) and KSCN aqueous solution . Electrochim. Acta. , 50 : 2343 – 2349 . doi: 10.1016/j.electacta.2004.10.048
- O’Regan , B , Schwartz , D T , Zakeeruddin , S M and Grtzel , M . 2000 . Electrodeposited nanocomposite n–p heterojunctions for solid-state dye-sensitized photovoltaics . Adv. Mater. , 12 : 1263 – 1266 . doi: 10.1002/1521-4095(200009)12:17<1263::AID-ADMA1263>3.0.CO;2-T
- Perera , V PS , Pitigala , P KDDP , Jayaweera , P VV , Bandaranayake , K MP and Tennakone , K . 2003 . Dye-sensitized solid-state photovoltaic cells based on dye multilayer-semiconductor nanostructures . J. Phys. Chem. B , 107 : 13758 – 13761 . doi: 10.1021/jp0348979
- Tennakone , K , Kumara , G RRA , Kottegoda , I RM , Perera , V PS and Weerasundara , P SRS . 1998 . Sensitization of nano-porous films of TiO2 with santalin (red sandalwood pigment) and construction of dye- sensitized solid-state photovoltaic cells . J. Photochem. Photobiol. A Chem. , 117 : 137 – 142 . doi: 10.1016/S1010-6030(98)00344-X
- Sankapal , B R , Goncalves , E , Ennaoui , A and Ch. Lux-Steiner , M . 2004 . Wide band gap p-type windows by CBD and SILAR methods . Thin Solid Films , 451–452 : 128 – 132 . doi: 10.1016/j.tsf.2003.11.002
- Zhang , H Q , Marin , V , Fijten , M WM and Schubert , U S . 2004 . High-throughput experimentation in atom transfer radical polymerization: A general approach toward a directed design and understanding of optimal catalytic systems . J. Polym. Sci., Part A: Polym Chem. , 42 : 1876 – 1885 . doi: 10.1002/pola.20027
- Sindhu , K S , Sekhon , S S , Hashmi , S A and Chandra , S . 1993 . Studies on poly(ethylene oxide)-CuSCN polymer electrolytes . Eur. Polym. J. , 29 : 779 – 782 . doi: 10.1016/0014-3057(93)90327-C
- Zhuge , F W , Gao , X D , Li , X M and Gan , X Y . 2009 . Microstructure and optical properties of CuSCN thin film deposited by Successive Ionic Layer Adsorption and Reaction (SILAR) method . J. Inorg. Mater. , 24 : 8 – 12 . doi: 10.3724/SP.J.1077.2009.00008
- Bringley , J F , Eachus , R S and Marchetti , A P . 2002 . Aqueous phase self-assembly of nanoscale p−n heterojunctions . J. Phys. Chem. B , 106 : 5346 – 5350 . doi: 10.1021/jp020024n
- Tacconi , N R and Rajeshwar , K . 2002 . Semiconductor nanostructures in an alumina template matrix: micro-versus macro-scale photoelectrochemical behavior . Electrochim. Acta , 47 : 2603 – 2613 . doi: 10.1016/S0013-4686(02)00121-4
- Yang , M and Li , J J . 2009 . Synthesis and characterizations of monodispersed nanospheres of β-CuSCN using CuO as the precursor . J. Dispersion Sci. Technol. , 30 : 361 – 364 . doi: 10.1080/01932690802548122
- Zhang , W Q , Yang , Q , Xu , L Q , Yu , W C and Qian , Y T . 2005 . Growth of PbS crystals from nanocubes to eight-horn-shaped dendrites through a complex synthetic route . Mater. Lett. , 59 : 3383 – 3388 . doi: 10.1016/j.matlet.2004.09.065
- Cholak , J , Hubbard , D M and Burkey , R E . 1948 . Microdetermination of lead in biological material with dithizone extraction at high pH . Anal. Chem. , 20 : 671 – 678 . doi: 10.1021/ac60019a026
- Merlwether , L S , Breitner , E C and Sloan , C L . 1965 . The photochromism of metal dithizonates . J. Am. Chem. Soc. , 87 : 4441 – 4448 . doi: 10.1021/ja00948a006
- Chen , Y B , Chen , L and Wu , L M . 2008 . Water-induced thermolytic formation of homogeneous core-shell CuS microspheres and their shape retention on desulfurization . Cryst Growth Des. , 8 : 2736 – 2740 . doi: 10.1021/cg700838s
- Tennakone , K , Jayatissa , A H , Fernando , C AN , Wickramanayake , S , Punchihewa , S , Weerasena , L K and Premasiri , W DR . 1987 . Semiconducting and photoelectrochemical properties of n- and p-type β-CuCNS . Phys. Status Solidi A , 103 : 491 – 497 . doi: 10.1002/pssa.2211030220