Abstract
This study describes a new and simple approach to produce multiple optical vortices (OVs) using three-dimensional polymeric photonic crystal (PhC). The crystals are fabricated by well-known inward-growing self-assembly technique using polystyrene micro-particles of particle sizes 990 and 1900 nm. This fabrication technique provides the cubic lattice arrangement to the spheres with the ⟨1 1 1⟩ plane parallel to the substrate. When a Gaussian beam from a He–Ne laser at 633 nm is diffracted through the crystal of particle size 990 nm, six bright spots along with a central spot are observed on the screen. Diffractions are observed up to third-order in the case of 1900 nm particle size PhC. Multiple OVs arise when vortex beam with topological charge 1 is diffracted through such a crystal. The diffraction pattern shows similar arrangement of bright spots as for the Gaussian beam except now the diffracted spots contain the property of incident OV, i.e. the central dark core. The diffraction is recorded using a charge coupling device camera.
1. Introduction
Propagation of electromagnetic waves in a medium containing periodically varying refractive index has attracted attention because of its wide application in optics and photonics. Such medium is known as photonic crystal (PhC). Depending upon the application, the PhC can be synthesised by top-down Citation1 or bottom-up Citation2 technique. A colloidal PhC which can be fabricated by the bottom-up self-assembly method contains periodically arranged micro-spheres in two-dimensional (2-D) or three-dimensional (3-D) geometry. The colloidal particles can be a polymer or an inorganic material. When a light beam with wavelength comparable to the lattice constant of the PhC diffract from crystal planes, the far-field diffraction pattern provides the information about the lattice arrangement in the crystal Citation3.
In the present case, 3-D polymeric PhCs are used to study the diffraction of optical vortex (OV) beam to achieve multiple vortex beams. An OV is a light beam whose phase rotates in a helical form around its axis of propagation; hence it has a helical wave front Citation4, as shown in . Such beams contain an azimuthal phase term eimϕ , where m is the topological charge of OV. Topological charge is an important factor; it provides information about the orbital angular momentum of the incident vortex beam. For a vortex of topological charge m, the beam contains mђ orbital angular momentum per photon. The transverse intensity cross-section of OV contains a bright ring with a dark core at the centre, shown in . The phase profile of OV is shown in Citation5. An OV can be generated by diffracting a Gaussian laser beam through a computer generated hologram (CGH), which is an interferogram obtained by theoretically interfering a Gaussian beam with a vortex beam Citation6. The interference profile looks like a fork pattern, which is shown in Citation5. This pattern is called CGH and it can be transferred to a holographic plate or to a spatial light modulator (SLM). When a laser beam (Gaussian beam) diffracts from the branch point of the fork pattern of the CGH, different orders of OVs generate in diffraction.
Figure 1. (a) Helical wave front (adopted from Padgett and Allen Citation4), (b) intensity profile, (c) phase profile and (d) characteristic interference pattern with a reference beam of an OV of topological charge 1.
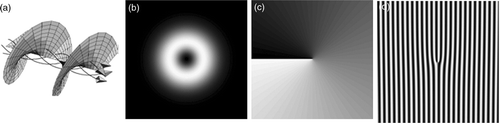
A number of groups have been studying the diffraction of OV through the photonic lattices to generate solitons Citation7–12. Terhalle et al. Citation7 reported the generation of multivortex solitons when vortex beam diffract from laser-induced photonic lattice in a strontium barium niobate crystal. Alexander et al. Citation11 used triangular photonic lattice to demonstrate the self-trapped states of light in the form of multivortex localised states. Neshev et al. Citation12 showed discrete vortex solitons in 2-D photonic lattice which was optically induced photorefractive crystal. In most of the cases, the lattice was induced in photorefractive material by illuminating it with a suitable interference fringe Citation7,Citation9,Citation10,Citation12.
Unlike the above cases, in this study self-assembled colloidal PhCs are used to explore the diffraction of OV. The PhCs are fabricated using polystyrene (PS) colloidal particles of sphere diameters 990 and 1900 nm by self-assembly technique Citation2. From scanning electron microscope (SEM) image of the crystal, it is found that the particles are arranged in a hexagonal closed pack form on the surface of the crystal. To study diffraction, the PhCs are first illuminated with Gaussian beam. First-order diffraction pattern is observed on the screen placed at a distance of 5 mm from the PS 990 nm PhC, while at the same screen, second- and third-order diffractions along with first-order are observed for PS 1900 nm PhC. In the next step, the OV with topological charge 1 is diffracted through PhCs and diffraction patterns are observed on the screen. The patterns are recorded using charge coupling device (CCD) camera.
2. Experimental set-up
Commercially available mono-dispersed PS micro-particles (from Bangs Laboratories, Inc.) with sphere diameters 990 and 1900 nm are used to synthesise PhCs by the inward-growing self-assembly method Citation2. In this method, an optimised volume of colloidal solution is dried on a horizontally placed glass substrate. Within 3–4 h, the particles get arranged in face-centred cubic lattice form. Multiple OVs are generated by diffracting a single vortex beam through the fabricated crystals.
is the schematic of the experimental set-up used to study the vortex diffraction from self-assembled PhCs. Light from an He–Ne laser at 633 nm is incident on the SLM which contains the fork pattern (). Different orders of OV generate and pass through the beam splitter (BS). An aperture (A) is used to choose the first-order OV, and it is then focussed with a microscopic objective lens (L1, 10×) on the PhC. The diffraction from the PhC is observed on a screen placed at 5 mm from the crystal. The pattern is imaged in the transmission mode of the crystal by using a lens (L2) and a CCD camera.
3. Results and discussion
shows the SEM images of the PS PhCs with sphere diameter 990 nm and 1900 nm, respectively. The hexagonal arrangement of colloidal particles on the surface of the PhC is clearly visible in both the images, this represents the ⟨1 1 1⟩ plane of the cubic lattice.
is the diffraction pattern of a Gaussian laser beam when it diffracts from the PS 990 nm PhC. Six bright spots surrounding the centre bright spot can be seen in the image. The six spots arise due to diffraction from the parallel planes of the crystal, containing hexagonal arrangement of PS particles. The intensity of all the spots is found to be nearly equal. is the diffraction pattern of an OV when it passes through the same PhC. One can notice that the intensity profile of the diffracted spots is different than the Gaussian beam diffraction. The characteristic dark area at the centre of a vortex is clearly visible in each spot. The diffraction of Gaussian beam from the PS 1900 nm PhC is shown in . Second- and third-order diffractions along with the first-order (inner six spots) are visible in the picture. In this case, the intensity of inner spots is found to be more than the outer spots. Also, the spots corresponding to higher diffraction order diverge more than the lower order. Multiple OV beams are seen when the OV diffracts through the crystal, as shown in . The dark centre of the spots is more clearly visible in the higher order diffraction. One can also notice the dark core of the central beam.
Figure 4. Diffraction pattern of: (a) Gaussian beam from PS 990 nm PhC, (b) OV from PS 990 nm PhC, (c) Gaussian beam from 1900 nm PhC and (d) OV from 1900 nm PhC.
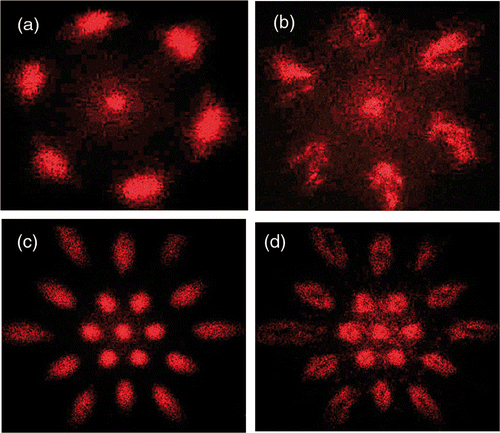
The solid and the dotted lines in are the intensity profiles of the spots arising due to the diffraction of Gaussian and vortex beams, respectively, in PS 990 nm PhC. A dip is observed (shown with arrow) in the dotted curve as compared to solid line, this represents the dark region where the light intensity reaches zero for OV beam. Similar effect is observed (shown with arrow) for the diffracted spot of OV beam (dotted line) compared to the Gaussian beam (solid line) for PS 1900 nm PhC, as shown in .
Figure 5. Intensity profile of the diffracted spot of the Gaussian beam (solid line) and the vortex beam (dotted line) in: (a) PS 990 nm PhC and (b) PS 1900 nm PhC.
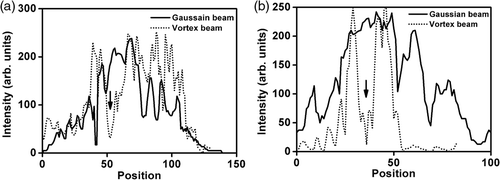
In conclusion, 3-D colloidal PhCs are suitable aperture to diffract an OV beam and to produce multiple OVs. Higher order diffractions can be observed in the PhC with large particle size. The generated vortices show similar arrangement as the colloidal particles in the PhCs. Hence, this is a simple way to multiply OVs for different applications.
References
- Matthias , S , Muller , F and Gosele , U . 2005 . Simple cubic three-dimensional photonic crystals based on macroporous silicon and anisotropic posttreatment . J. Appl. Phys. , 98 : 023524-1 – 023524-4 . doi: 10.1063/1.1993752
- Yan , Q , Zhou , Z and Zhao , X S . 2005 . Inward-growing self-assembly of colloidal crystal films on horizontal substrate . Langmuir , 21 : 3158 – 3164 . doi: 10.1021/la047337p
- Santamaria , F G , Lopez , J FG , Braun , P V and Lopez , C . 2005 . Optical diffraction and high-energy feature in three-dimensional photonic crystals . Phys. Rev. B , 71 : 195112-1 – 195112-5 . doi: 10.1103/PhysRevB.71.195112
- Padgett , M and Allen , L . 2000 . Light with a twist in its tail . Contemp. Phys. , 41 : 275 – 285 . doi: 10.1080/001075100750012777
- Kumar , A , Vaity , P and Singh , R P . 2010 . Diffraction characteristics of optical vortex passing through an aperture–iris diaphragm . Opt. Commun. , 283 : 4141 – 4145 . doi: 10.1016/j.optcom.2010.06.045
- Carpentier , A V , Michinel , H , Salgueiro , J R and Olivieri , D . 2008 . Making optical vortices with computer-generated holograms . Am. J. Phys. , 76 : 916 – 921 . doi: 10.1119/1.2955792
- Terhalle , B , Richter , T , Desyatnikov , A S , Neshev , D N , Krolikowski , W , Kaiser , F , Denz , C and Kivshar , Y S . 2008 . Observation of multivortex solitons in photonic lattices . Phys. Rev. Lett. , 101 : 031903-1 – 031903-4 . doi: 10.1103/PhysRevLett.101.013903
- Salgueiro , J R and Kivshar , Y S . 2009 . Optical vortex solitons and soliton clusters in photonic crystal fibres . Eur. Phys. J. Special Topics , 173 : 281 – 288 . doi: 10.1140/epjst/e2009-01080-0
- Chen , Z , Martin , H , Bezryadina , A , Neshev , D , Kivshar , Y S and Christodoulides , D N . 2005 . Experiments on Gaussian beams and vortices in optically induced photonic lattices . J. Opt. Soc. Am. B , 22 : 1395 – 1405 . doi: 10.1364/JOSAB.22.001395
- Yang , J , Makasyuk , I , Kevrekidis , P G , Martin , H , Malomed , B A , Frantzeskakis , D J and Chen , Z . 2005 . Necklace like solitons in optically induced photonic lattices . Phys. Rev. Lett. , 94 : 113902-1 – 113902-4 . doi: 10.1103/PhysRevLett.94.113902
- Alexander , T J , Desyatnikov , A S and Kivshar , Y S . 2007 . Multivortex solitons in triangular photonic lattices . Opt. Lett. , 32 : 1293 – 1295 . doi: 10.1364/OL.32.001293
- Neshev , D N , Alexander , T J , Ostrovskaya , E A , Kivshar , Y S , Martin , H , Makasyuk , I and Chen , Z . 2004 . Observation of discrete vortex solitons in optically induced photonic lattices . Phys. Rev. Lett. , 92 : 123903-1 – 123903-4 . doi: 10.1103/PhysRevLett.92.123903