Abstract
ZnS nanoparticles have been synthesised at low temperature by short reaction (80°C for 2 h) via wet chemical route. The nanoparticles were stabilised using hexamethylenetetramine (HMTA) as surfactant in aqueous solution. The average particle size calculated from X-ray diffraction studies was about 4 nm with cubic zincblende structure. The presence of HMTA in the synthesised ZnS nanoparticles was confirmed by FTIR studies. A significant blue shift was observed in the optical absorption band edge for the ZnS nanoparticles as compared to the bulk, indicating a strong quantum confinement. The room temperature photoluminescence (PL) spectrum showed a broad green emission peak at around 502 nm. The photocatalytic property of HMTA-stabilised ZnS nanoparticles were investigated on the decolourisation of methylene blue aqueous solution under UV light irradiation.
1. Introduction
Semiconductor nanoparticles have attracted much interest in recent years owing to their remarkable electronic, optical and photocatalytic properties due to quantum confinement effect Citation1,Citation2. Preparation and characterisation of semiconductor nanoparticles, nanofibres and nanorods have gained increased interest. Nevertheless, control over the morphology and crystal size of nanostructured materials is a new challenge to the materials scientists Citation3. Wide band gap II–VI semiconductors are expected to be the novel materials for the optoelectronic devices. Among which, ZnS is an important II–VI compound semiconductor with a wide direct band gap energy Citation4. To date, several conventional methods have been employed to synthesise II–VI semiconducting nanoparticles Citation5. However, it is well known that group II–VI materials form defects and inter-diffuse at higher temperature (above 500°C). Therefore, the synthesis of these materials at low temperature and short duration time provides attractive advantages, among which the surfactant-assisted technique has attracted much attention. It uses the organic or inorganic reagent to act as a passivator to prevent the coalescence of nanoparticles Citation6.
Even though surfactant-assisted technique is an effective pathway for the formation of nanoparticles, most of the commercial surfactant employed are hazardous chemical substances. Hence, it is necessary to adopt a more eco-friendly route for the fabrication of nanoparticles. Hexamethylenetetramine (HMTA), a non-toxic surfactant, has already been used as a stabilising (capping) agent for the preparation of ZnO and ZnS nanoparticles by hydrothermal method Citation7–9. In the present study, a wet chemical method has been optimised for the synthesis of ZnS nanoparticles at low temperature with short reaction time (80°C for 2 h) using HMTA as surfactant in aqueous solution. This synthetic route is simple and cost-effective for the preparation of semiconductor nanoparticles for optoelectronic and environmental applications. In order to understand the growth kinetics of ZnS nanoparticles, their structural and optical properties have been studied. The photocatalytic behaviour of ZnS nanoparticles has also been evaluated using methylene blue (MB) as a model organic compound.
2. Experimental
2.1. Synthesis of HMTA-stabilised ZnS nanoparticles
Zinc acetate dihydrate ((CH3COO)2Zn · 2H2O), thiourea (H2NCSNH2) and HMTA (C6H12N4), all analytical grade reagents were used without further purification. Deionised water was used throughout the experiments. For the synthesis of ZnS nanoparticles at low temperature and short reaction time, an appropriate amount of zinc acetate dihydrate along with 0.5 M of HMTA were added into 50 mL of double-distilled water and stirred for few minutes to get a clear solution. The resulting solution was added into 50 mL of aqueous solution containing certain amount of thiourea. Zinc and sulphur sources were taken in equimolar ratio. Under constant stirring, the final mixture was heated at 80°C for 2 h to get a precipitate. Then the resulting mixture was filtered and washed several times with double-distilled water and alcohol to remove possible impurities. The final product was dried at room temperature for 24 h and collected for characterisation studies. The flowchart for the wet chemical synthesis of HMTA-stabilised ZnS nanoparticles is shown in .
2.2. Characterisation studies
The powder X-ray diffraction (XRD) pattern was recorded using Siemens D5005 X-ray powder diffractometer with Cu-Kα radiation (λ = 1.5418 Å). FTIR spectra were recorded using Perkin Elmer RX1 Model FTIR spectrophotometer by KBr pellet method in the range between 4000 and 400 cm−1. Diffuse reflectance UV–Vis spectra (DRS-UV) of the prepared powder samples were recorded at room temperature using Shimadzu UV-2101 apparatus. TEM analysis was performed using JEOL 3010 TEM to study the morphology, structure, size and selected area electron diffraction (SAED) pattern of the prepared ZnS sample. Photoluminescence (PL) measurements were carried out at room temperature with an excitation wavelength of 310 nm using Hitachi F-2500 luminescence spectrometer. The photocatalytic behaviour of the prepared sample was examined using MB as a model organic compound using Perkin Elmer UV–Vis spectrophotometer at room temperature.
2.3. Photodegradation studies
To demonstrate the potential applicability of HMTA-capped ZnS nanoparticles in environmental remediation, its photocatalytic activity was studied using UV light. MB was used as a test contaminant, since it has been extensively used as an indicator for the photocatalytic activities owing to its absorption peaks in the visible range Citation10,Citation11. Photocatalytic degradation experiments were carried out by adding 0.075 g of HMTA-stabilised ZnS sample into a quartz photoreactor containing 80 mL of a 10 mg L−1 MB aqueous solution. After stirring for 30 min in dark in order to reach the adsorption equilibrium, the mixture was irradiated with a Hg lamp (300 W) at room temperature. The residual MB in the aqueous solution was analysed by checking the absorbance at 663 nm in the UV–Vis absorption spectra.
To determine the percentage of degradation of MB, the samples were collected at regular intervals (for every 30 min), filtered, centrifuged to remove the nanophotocatalyst particles that exist as undissolved particles in the sample and studied using UV–Vis absorption. Photodegradation studies were performed by monitoring the absorbance of the dye solution at a wavelength of 663 nm.
The degradation percentage of the dye in the presence and absence of ZnS nanoparticles can be calculated from the following equation Citation12:
3. Results and discussion
3.1. Structural properties
Powder XRD pattern of HMTA-stabilised ZnS nanoparticles is shown in . By comparing with the data from JCPDS (File No. 05-0566), all the diffraction peaks can be indexed to cubic zinc blende structure with lattice constants a = b = c = 5.40 Å Citation13. The d-spacing with corresponding (hkl) planes for the prepared ZnS samples are (111) 3.1253 Å, (220) 1.9096 Å, (311) 1.6331 Å and a = 5.4103 Å, V = 158.36 Å3. These values are consistent with the standard cubic phase of ZnS Citation14. The average crystallite size of about 4 nm was estimated according to the line width analysis of the strong intense diffraction peak based on the Scherrer's formula
3.2. TEM analysis
TEM images of HMTA-stabilised ZnS sample are shown in . It is observed that the HMTA-stabilised ZnS particles possess spherical morphology. The structure of ZnS nanoparticles was revealed by the SAED pattern (). The SAED pattern shows a set of broad rings due to the random orientation of the crystallites, corresponding to diffraction from different planes of the nanocrystallites. The SAED pattern exhibit three rings corresponding to (111), (220) and (311) planes, respectively, which is in agreement with the cubic phase of ZnS Citation15.
3.3. FTIR spectral analysis
The FTIR transmission spectra of (a) HMTA and (b) HMTA-stabilised ZnS nanoparticles are shown in . It reveals that all the absorption peaks of HMTA () match with those reported in the literature Citation16. The spectrum () reveals the C–H stretching vibration bands at 2920 and 2863 cm−1, the C–H bending modes at 1487 cm−1 and the relatively weak methylene rocking vibration at 690 cm−1. The vibration at 690 cm−1 is the characteristic of a minimum of four methyl groups, (CH2)4 in a row and assigned to the methylene rocking vibration. Hence, it is concluded that the long alkyl chain does exist in HMTA Citation17. A peak due to the stretching vibrations of C–H bonds in tertiary amines of HMTA at 1391 and 1006 cm−1was observed in the vibrational spectra Citation18. The absorption peaks at 3312 and 3489 cm−1 are assigned to O–H stretching.
For the HMTA-stabilised ZnS nanoparticles (), the C–H bonding and O–H stretching vibrations are revealed by the absorption peaks at 2900, 2815, 1557, 1400, 1021 and 3462 cm−1, respectively. The peak at around 619 cm−1 is assigned to Zn–S stretching vibration Citation19. Thus, it is confirmed from the FTIR studies that, when the ZnS nanoparticles are encapsulated by the stabilising agent (HMTA), a shift in the absorption peak is observed, indicating that the capping process is successful Citation20.
3.4. Optical absorption studies
The DRS-UV of HMTA-stabilised ZnS nanoparticles are shown in . Generally, the wavelength of maximum exciton absorption (λ max) would decrease as the size of the nanoparticles decreases, as a consequence of quantum confinement of the photogenerated electron–hole carriers Citation21. It can be seen that the prepared sample is fairly blue-shifted from the absorption edge of the bulk ZnS (345 nm) Citation22. The blue shift in the absorption spectra is attributed to the quantum confinement of charge carriers. The absorption edge of the prepared sample is also sharp due to the relatively narrow size distribution of the synthesised particles. When the size of HMTA-stabilised ZnS nanoparticles decreased, the band gap energy increased, which in turn led to higher redox potential in the system. Therefore, the nanoscale ZnS is expected to have higher photocatalytic activity than its bulk Citation12.
The optical energy band gap has been calculated by using the Tauc relation Citation23,
3.5. PL studies
PL spectrum of HMTA-stabilised ZnS nanoparticles was recorded at room temperature with an excitation wavelength (λ exc) of 310 nm, as shown in . Denzler et al. Citation25 reported that different kinds of lattice defects like sulphur or zinc vacancies or interstitials may give rise to fluorescence emission at different positions and produce a broad spectrum. Broadening of the emission peak could be attributed to both size distribution and increase in the surface state owing to the increase in surface to volume ratio. It is observed that the PL spectra of HMTA-stabilised ZnS nanoparticles exhibit strong trap-state green emission at around 502 nm. The green emission could be attributed to some self-activated defect centres related to Zn-vacancies Citation26.
3.6. Photocatalytic degradation studies
The photocatalytic degradation of organic matter (pollutants) in aqueous solution was initiated by UV light irradiation to excite an electron from the valence band (VB) to the conduction band of the ZnS nanoparticles resulting in the formation of photogenerated electron–hole pair. The highly oxidative holes on VB not only directly decompose the dye molecules adsorbed on the surface of ZnS particles, but also oxidise H2O or OH− to form OH• with high activity and indirectly degrade the dye molecules in aqueous solution. In addition, the electrons may then react with species adsorbed on the surface, yielding radicals such as as a result of the presence of hydroxyl groups, water and oxygen at the surface of the ZnS particles. These radicals then react with the surrounding organic dye molecules, leading to volatile degradation by-products or entire mineralisation into CO2, H2O and mineral acids.
The proposed mechanism for the degradation of organic dyes using HMTA-capped ZnS nanoparticles as photocatalyst is given as Citation27
In order to study the photodegradation kinetics of MB, a comparative experiment was carried out in the absence and presence of HMTA-stabilised ZnS nanoparticles under UV light irradiation. The results are illustrated in . It is observed that under identical conditions with exposure to UV light irradiation, MB degrades effectively in the presence of HMTA-stabilised ZnS nanoparticles. Thus, HMTA-stabilised ZnS nanoparticles showed better degradation efficiency because it possess higher surface area to volume ratio and thus allows greater photon absorption on the photocatalyst surface Citation2,Citation12. The degradation of MB in the presence of HMTA-stabilised ZnS nanoparticles follows first-order kinetics Citation28.
Figure 8. Photocatalytic degradation of MB in the (a) absence and (b) presence of nanophotocatalyst.
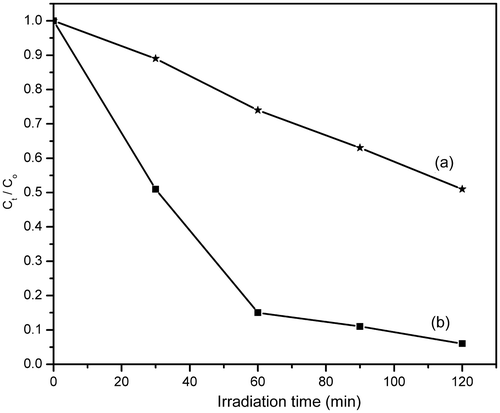
shows the degradation percentage of MB with irradiation time in the absence and presence of HMTA-stabilised ZnS nanoparticles. The degradation efficiency of MB in aqueous solution in the presence of HMTA-stabilised ZnS nanoparticles reaches 95% after 120 min of UV light irradiation, while the comparative degradation efficiency in the absence of HMTA-stabilised ZnS nanoparticles is only 49% for the same irradiation time. These results show that the as-prepared HMTA-stabilised ZnS nanoparticles exhibit strong photocatalytic activity with potential application in the degradation of MB and other organic compounds (dyes).
4. Conclusion
ZnS nanoparticles have been synthesised at low temperature within a short reaction time by a simple wet chemical method using HMTA as surfactant in aqueous solution. XRD results reveal that the synthesised nanoparticles exhibit cubic zinc blende structure with an average grain size of about 4 nm. Formation of HMTA-capped ZnS nanoparticles were confirmed by FTIR studies. A significant blue shift in the absorption band edge was observed for the HMTA-stabilised ZnS nanoparticles compared to the bulk ZnS, and the optical band gap energy is estimated to be around 4.98 eV. The PL spectra of HMTA-stabilised ZnS nanoparticles exhibit a strong-green emission peak at around 502 nm due to some self-activated defect centres related to Zn-vacancies. The photocatalytic investigation shows that the degradation efficiency of organic pollutants can be doubled in the presence of HMTA-stabilised ZnS nanoparticles.
Acknowledgements
The authors wish to thank Dr M. Kandaswamy, Department of Inorganic Chemistry, University of Madras, Guindy Campus, Chennai, for providing the FTIR facility. The authors are very much thankful to Dr K. Pandian, Department of Inorganic Chemistry, University of Madras, Guindy Campus, Chennai, for extending the facilities for photocatalytic measurements. TEM facilities provided by Indian Institute of Technology, Mumbai, are greatly acknowledged. The authors wish to thank Dr A. Pandurangan, Department of Chemistry, Anna University, Chennai, for DRS-UV measurements.
References
- Naskar , M K , Patra , A and Chatterjee , M . 2006 . Understanding the role of surfactants on the preparation of ZnS nanocrystals . J. Colloid Interf. Sci. , 297 : 271 – 275 .
- Hoffmann , M R , Martin , S T , Choi , W and Bahnemannt , D W . 1995 . Environmental applications of semiconductor photocatalysis . Chem. Rev. , 95 : 69 – 96 .
- Shiang , J J , Kadavanich , A V , Grubbs , R K and Alivisatos , A P . 1995 . Symmetry of annealed wurtzite CdSe nanocrystals: Assignemnt to the C3v point group . J. Phys. Chem. , 99 : 17417 – 17422 .
- Senthilkumaar , S and Thamizselvi , R . 2008 . Synthesis and characterization of one dimensional ZnS nanorods . Synth. React. Inorg. Metal Org. Nano Metal Chem. , 38 : 710 – 715 .
- Vadivelmurugan , A , Kale , B B , Kulkarni , A V , Kunde , L B and Saaminathan , V . 2005 . Novel approach to control CdS morphology by simple micro-wave solvothermal method . J. Mater. Sci. Mater. Elect. , 16 : 295 – 299 .
- Mu , J , Gu , D and Gu , X . 2005 . Synthesis of water-soluble coprecipated CdxZn(1−x)S nanoparticles . J. Disper. Sci. Tech. , 26 : 193 – 195 .
- Ismail , A A , El-Midany , A , Abdel-Aal , E A and El-Shall , H . 2005 . Application of statistical design to optimize the preparation of ZnO nanoparticles via hydrothermal technique . Mater. Lett. , 59 : 1924 – 1928 .
- Vijaianand , K , Karlchinnu , M , Mohankumar , R , Mohan , R and Jayavel , R . 2009 . Formation of zinc sulfide nanoparticles in HMTA matrix . Appl. Surf. Sci. , 255 : 8879 – 8882 .
- Vijaianand , K , Karlchinnu , M , Mohankumar , R , Mohan , R and Jayavel , R . 2010 . Thermal stability and optical properties of HMTA capped zinc sulfide nanoparticles . J. Alloys Compd. , 496 : 665 – 668 .
- Wang , R , Xin , J H , Yang , Y , Liu , H , Xu , I and Hu , J . 2004 . The characteristics and photocatalytic activities of silver doped ZnO nanocrystallites . Appl. Surf. Sci. , 227 : 312 – 317 .
- Lachheb , L , Puzenat , E , Houas , A , Ksibi , M , Elslousi , E , Guillard , C and Hermman , J M . 2001 . Photocatalytic degradation pathway of methylene blue in water . Appl. Catal. B Environ. , 31 : 145 – 157 .
- Pouretedal , H R , Norozi , A , Keshavarz , M H and Semnani , A . 2008 . Nanoparticles of zinc sulfide doped with manganese, nickel and copper as nanophotocatalyst in the degradation of organic dyes . J. Hazard. Mater. , 162 : 674 – 681 .
- Chen , L , Shang , Y , Xu , J , Liu , H and Hu , Y . 2006 . Synthesis of ZnS nanospheres in microemulsion containing cationic Gemini surfactant . J. Disper. Sci. Tech. , 27 : 839 – 842 .
- Hoa , T Q , Vu , L V , Canh , T D and Long , N N . 2009 . Preparation of ZnS nanoparticles by hydrothermal method . J. Phys. Conf. Ser. , 187 : 012081 – 012086 .
- Gao , H J , Lu , C , Lu , X and Du , Y . 2007 . Aphen-functionalized nanoparticles-polymer fluorescent nanocomposites via ligand exchange and in situ bulk polymerization . J. Mater. Chem. , 17 : 4591 – 4597 .
- Zhang , Y , Wang , S , Qian , Y and Zhang , Z . 2006 . Complexing-reagent assisted synthesis of hollow CuO microspheres . Solid State Sci. , 8 : 462 – 466 .
- Chen , S and Liu , W . 1999 . Preparation and characterization of surface-coated ZnS nanoparticles . Langmuir , 15 : 8100 – 8104 .
- Sugunan , A , Warad , H C , Boman , M and Dutta , J . 2006 . Zinc oxide nanowires in chemical bath on seeded substrates: Role of hexamine . J. Sol-Gel Sci. Technol. , 39 : 49 – 56 .
- Remadevi , B S , Raveendran , R and Vaidyan , A V . 2007 . Synthesis and characterization of Mn2+ doped ZnS nanoparticles . Pramana , 68 : 679 – 687 .
- Sharma , M , Kumar , S and Pandey , O P . 2010 . Study of energy transfer from capping agents to intrinsic vacancies/defects in passivated ZnS nanoparticles . J. Nanopart. Res. , 12 : 2655 – 2666 .
- Khiew , P S , Radiman , S , Huang , N M , Ahmad , M S and Nadarajah , K . 2005 . Preparation and characterization of ZnS nanoparticles synthesized from chitosan laurate micellar solution . Mater. Lett. , 59 : 989 – 993 .
- Dutta , K , Manna , S and De , S K . 2009 . Optical and electrical characterizations of ZnS nanoparticles embedded in conducting polymer . Synth. Met. , 159 : 315 – 319 .
- Tauc , J . 1974 . Amorphous and Liquid Semiconductor , New York : Plenium Press .
- Jindal , Z and Verma , N K . 2008 . Photoluminescent properties of ZnS:Mn nanoparticles with in-built surfactant . J. Mater. Sci. , 43 : 6539 – 6545 .
- Denzler , D , Olschewski , M and Satter , K . 1998 . Luminescence studies of localized gap states in colloidal ZnS nanocrystals . J. Appl. Phys. , 84 : 2841 – 2845 .
- Kar , S , Biswas , S and Chaudhuri , S . 2005 . Catalytic growth and photoluminescence properties of ZnS nanowires . Nanotechnology , 16 : 737 – 740 .
- Aarthi , T , Narahari , P and Madras , G . 2007 . Photocatalytic degradation of Azure and Sudan dyes using nano TiO2 . J. Hazard. Mater. , 149 : 725 – 734 .
- Hu , J S , Ren , L L , Guo , Y G , Liang , H P , Cao , A M , Wan , L J and Bai , C L . 2005 . Mass production and high photocatalytic activity of ZnS nanoporous nanoparticles . Angew. Chem. Int. Ed. , 44 : 1269 – 1273 .