Abstract
This work aimed to study cobalt nanoparticles (Co-NPs) preparation using three different methods in order to evaluate the effect of synthesis variables that can influence the nanoparticle size distribution and particle shape. The synthesised nanoparticles were characterised by Transmission Electron Microscopy. The first synthesis employed decomposition of Co2(CO)8, at high temperatures. This procedure resulted in spherical nanoparticles with low size distribution. The size of Co-NPs could be tuned by modification of precursor/surfactant, nevertheless the stirring and injection time influenced the size distribution. Using polyol process, at high temperatures, it was produced undefined-shape nanoparticles. This result suggests that the solution composition, i.e. the amount of trioctylphosphine and oleic acid was not suitable to control both size and shape of nanoparticles. Finally, the method based on reduction with NaBH4 resulted spherical nanoparticles with tiny sizes, indicating that in this case a variation on amount of reductant would be more efficient on the particle size control than a variation in concentration of oleic acid. These results indicated that, for each method, a different variable exists for the control of the distribution size and the shape of the formed particles.
1. Introduction
There is a growing interest in organising materials on the nanometer scale for fundamental investigations or novel technological applications Citation1. Nanoscale and nanoparticle materials are expected to exhibit chemical and physical properties different from bulk materials or single atoms Citation2. These materials have significant potential as higher activity and selectivity catalysts to a range of reactions, including hydrogenations, enantioselective hydrogenations, hydrosilylations, hydropyrolysis and hydrogenolysis, oxidation of CO and CO/H2, oxidative acetoxylation, Suzuki and Heck-Type couplings and cycloaddition reactions Citation3. Especially, cobalt nanoparticles (Co-NPs) can be used to obtain catalysts for the Fischer–Tropsch synthesis (FTS). In this way, it is possible to control the size, shape and metallic dispersion, increasing the catalytic performance in comparison to conventional catalysts. According to Bezemer et al. Citation4, the particle size has a large influence in the behaviour of cobalt-based Fischer–Tropsch catalysts. It was observed that cobalt catalysts for FTS with average cobalt particle size above the optimum value of 6–8 nm exhibit lower activity and smaller particles display both a lower activity and a lower selectivity.
The chemical synthesis of Co-NPs can be usually accomplished by reduction of metallic salt Citation5–9, reverse micelles Citation2,Citation10,Citation11, or thermal decomposition of an organometallic precursor Citation12–16. The first step of the synthesis involves the precursor reduction followed by the nucleation, forming an aggregate of atoms. After this, the particle growth occurs. The metal growth is controlled by an organic molecule (surfactant or passivator). Then, each nanoparticle in a sample consists of an inorganic crystalline core surrounded by an organic monolayer Citation17. Through adjustment of the temperature, injection time and precursor/surfactant ratio Citation14 or oleic acid/trioctylphosphine (TOP) Citation9, the size of nanoparticles can be controlled.
Therefore, this work aims to study Co-NPs preparation using polyol method, thermal decomposition of an organometallic and reduction of cobalt salt by borohydride in order to evaluate the effect of synthesis variables that can influence the nanoparticle size distribution and particle shape.
2. Methods
The first method selected for nanoparticles production was based on the reduction of cobalt salt by a polyalcohol (polyol) Citation8. In brief, 1.0 g cobalt acetate and 1.5 mL oleic acid were mixed in 40 mL diphenyl ether (DPE) and heated to 200°C under N2 atmosphere. A volume of 1.0 mL of TOP was added and the mixture was heated to 250°C. Subsequently, the reducing solvent (4.0 g 1,2-dodecanediol dissolved in 10 mL DPE), at 80°C, was injected into the mixture. The mixture was held at 250°C for 30 min until the reduction was complete and then it was cooled to room temperature. Ethanol was used to precipitate Co nanoparticles. The solution was centrifuged (3000 rpm) and the nanoparticles were redispersed in a solution of 94% octane, 5% octhanol and 1% oleic acid.
The second method studied was described by Puntes and Krishnan Citation2, based on the decomposition of an organometallic. A typical synthesis consisted of heating 100 mg of TOP oxide to 80°C/30 min under vacuum. It was injected with 14.4 mL of 1,2-dichlorobenzene (DCB) and oleic acid (OA) and the mixture was heated to 180°C under N2 atmosphere. Then, 0.54 g Co2(CO)8 diluted in 3.0 mL DCB was rapidly injected into the solution under vigorous agitation. The variation of amount of oleic acid was adopted to control the particle size, using the OA/Co2(CO)8 molar ratio of 0.2, 0.4 and 0.8 Citation16 to prepare CoG, CoM and CoP nanoparticles, which refer to large, medium and small nanoparticles, respectively. The flask was kept at 180°C for 15 min and cooled to room temperature. Ethanol was used for nanoparticles precipitation. The solution was centrifuged (3000 rpm) and the nanoparticles were redispersed in a solution of 94% octane, 5% octhanol and 1% oleic acid.
The last method studied was proposed by Zhao et al. Citation6, based on the reduction of salt metallic by borohydride. The synthesis consists of the addition of a mixture of NABH4 and ethanol to a solution of cobalt acetate, ethanol and oleic acid in a drop-like manner, at room temperature. The same OA/precursor ratio of the second method was adopted for CoG nanoparticles preparation (0.2). The molar ratio of the mixture was 1 Co(CH3COO)2 : 6 ethanol : 0.2 OA : 2 NaBH4. Finally, the solution was centrifuged (3000 rpm) and the nanoparticles were redispersed in a solution of 94% octane, 5% octhanol and 1% oleic acid.
High-resolution transmission electron microscopy (HRTEM) was done in a JEOL JEM 3010 URP equipment operating at 300 kV (1.7 Å point resolution). transmission electron microscopy (TEM) samples were prepared by diluting the colloid of Co-NPs in hexane and dropping it onto carbon film supported on copper grid. The data were analysed using Gatan DigitalMicrograph and Microcal Origin 7.5 software. The interplanar distances observed in the HRTEM images were measured using the Size Meter 1.1 software and these values were applied to the Bragg's Law in order to get the angle 2θ that refers to the particle structure.
3. Results and discussion
TEM images of Co-NPs prepared by the first method Citation8 are shown in . As it can be observed, Co-NPs presented undefined shape and size, with some agglomerations indicating that the nanoparticles were not well dispersed. This result is similar to that obtained by Wu et al. Citation8. Then, it was not possible to obtain the histogram of size distribution for this method. The polyol method is a slow process, which takes more time for nucleation and growth of nanoparticles due to slow reaction rate and low monomer concentration. Therefore, the shape control of Co-NPs by polyol process is more difficult than it is with other process.
Besides, according to Lisiecki Citation18, the best control of the particle shape is obtained by combining the strategy of the surfactant-based template and the selective adsorption of salts. In this way, composition of the solution greatly affects the morphology of the products and the surfactant acts as a kind of shape director Citation19. Using polyol process, the oleic acid is chemisorbed as a carboxylate onto the Co-NPs, and the two oxygen atoms in the carboxylate are coordinated symmetrically to the Co atoms Citation8. Due to this fact, the addition of oleic acid makes facets on the surfaces of Co-NPs Citation20.
It should also be taken in consideration that the TOP acts as a selective absorber, altering the relative growth rates of different faces of the crystals. The crystal structures of Co, hexagonal close packed, face-centred cubic (fcc) and epsilon (ε), are close in energy, suggesting that the surfactant adhesion or modest variation in temperature affects the crystal shape Citation21. In the case of hexagonal cobalt, the primary particles are platelets and their agglomeration takes place through a stacking mode that resembles a columnar mesophase Citation22. Therefore, the amount of TOP added to the synthesis has an important role in the shape control of the prepared Co-NPs, and the unsatisfactory results obtained here suggest a necessity of optimisation of the amount of TOP and oleic acid to improve this method. Then, the synthesis presented by Wu et al. Citation8 should be supplemented, starting from a higher TOP/precursor molar ratio to reach a better control over the shape and size of the formed Co-NPs.
TEM and HRTEM images and the histograms of size distribution of Co-NPs prepared by the second method Citation2 showed that the formed nanoparticles are spherical, with uniform shape (). For all samples, were observed the interplanar distances by HRTEM images () of about 2.17 Å. These d spacings correspond to the metallic ε-Co structure formed Citation5, with reflections (220), which is typical of the synthesis based on the decomposition of organometallic at high temperature. According to , the average nanoparticle sizes observed were 7.2 ± 1.7, 7.1 ± 1.0 and 6.2 ± 1.0 nm to CoG, CoM and CoP nanoparticles, respectively.
Figure 2. TEM of Co-NPs prepared by the decomposition of cobalt carbonyl: TEM images of (a) CoP, (b) CoM and (c) CoG and HRTEM images of (d) CoP, (e) CoM and (f) CoG.
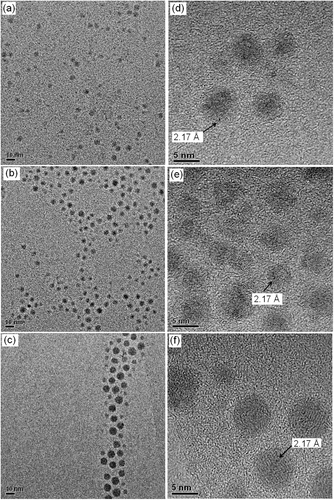
Figure 3. Histogram of size distribution of Co-NPs prepared by the decomposition of cobalt carbonyl: (a) CoP, (b) CoM and (c) CoG.
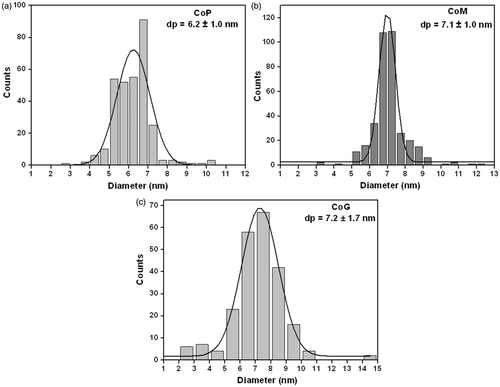
Beyond the close sizes to each other, CoG and CoP presented a lightly large size distribution, whereas the sample CoM presented narrower one. According to Puntes et al. Citation14, the decomposition and nucleation occurs instantaneously upon precursor injection. The lifetime of atoms in solution is short leading to the simultaneous formation of many small metal clusters (nuclei) Citation23. Unfortunately, since decomposition occurs very rapidly the resulting clusters have irregular sizes.
However, control of the bath temperature and the surfactant composition modifies the strength of the metallic particle–organic molecule bonding. By controlling the precursor/surfactant ratio, the reaction temperature, the injection time and the size of the spherical particles can be controlled Citation14. On the other hand, the growth of the particles, and hence their final size, is determined by the diffusion of precursor in solution and the driving forces between the growing clusters Citation24. In other words, the vigorous stirring and very rapid injection are crucial to control the size and shape of the nanoparticles formed. The closes sizes and lightly large distributions obtained by this method can be attributed to small stirring variations and to time/way of injection. In spite of this, the carbonyl decomposition procedure showed to be very effective and is cited in the literature Citation25 as scalable to large quantities for the commercial production of magnetic fluids. Then, the variation of precursor/surfactant molar ratio seems to be adequate for the size control of nanoparticles in this method, but the system must be well formulated and the operator must be agile enough to perform the injection directly into the vortex of the mixture stirred extremely quickly to control spherical shape and the size of the nanoparticles formed.
TEM and HRTEM images and the histogram of size distribution of Co-NPs prepared by the third method, based on reduction of precursor by sodium borohydride, are presented in . As it can be seen, the amount of oleic acid added was not enough to produce CoG nanoparticles, since the distribution size was 3.64 ± 0.64 nm, indicating that it is not possible to control the particle size only by the variation of the amount of oleic acid. As also observed when the method by Puntes and Krishnan Citation2 was employed, one can observe by HRTEM image interplanar distances of about 2.17 Å (), corresponding to the metallic ε-Co structure formed Citation5.
Figure 4. (a) TEM, (b) HRTEM images and (c) histogram of size distribution of Co-NPs prepared by the method of reduction by borohydride.
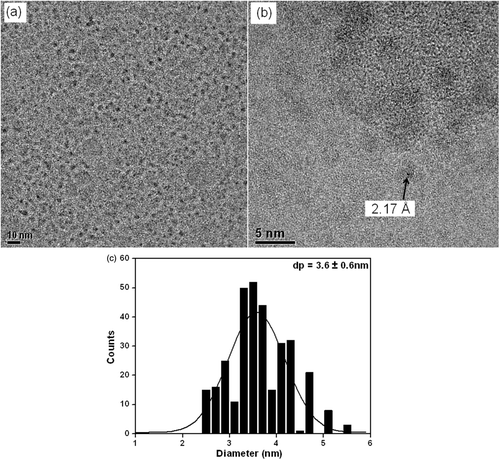
According to Zhao et al. Citation6, it is important to perform the reduction in a nonaqueous medium in order to get pure magnetic metal nanoparticles (e.g. fcc Co). In aqueous solution the primary products is Co2B, while in nonaqueous media the primary product is Co, followed by the decomposition of B2H6 in B and H2. The formation of these products is not affected by overall
/
ratio Citation26. However, the particle size depends on the
/metal ratio, pH and degree of mixing Citation27. Then, the amount of reducing agent is one of the key parameters in controlling the size distribution of nanocrystals. At high concentration of NaBH4, a strong reducing agent, cobalt nanocrystals has a very defined-shape, but was smaller Citation28. As in the method proposed by Zhao et al. Citation6 it was not cited the importance of the amount of reducing agent to control the size of particles formed, it should be stressed here that the amount of reducing agent is more important than the precursor/surfactant ratio. Then, it would be more efficient to variate the NaBH4/Co(CH3COO)2 ratio than the oleic acid/Co(CH3COO)2 ratio in order to control the particle size of the nanoparticles formed. This result is opposite that would be expected from the synthesis by decomposition of an organometallic.
4. Conclusion
In this work, it was demonstrated that Co-NPs can be obtained by chemical methods in an efficient way, with low size distribution, if some synthesis parameters are well controlled. The method based on the decomposition of cobalt carbonyl at high temperatures is the most appropriate to size and shape control by the variation in the ratio precursor/surfactant. However, a precise control can be obtained with more vigorous stirring and more rapid injection of the precursor. On the other hand, the composition of solution for the other two methods tested is the most important factor in the particle size control. For the polyol process, an adjustment in the amount of TOP and oleic acid can favour the formation of spherical nanoparticles, while in the method based on the reduction by sodium borohydride the amount of reducing agent influences the size of the formed particles significantly. In a general way, it can be concluded that the formation of Co-NPs is extremely sensitive to the synthesis conditions. Therefore, before using a synthesis method described in the literature, one should observe the real influence of synthesis conditions such as temperature, stirring and composition of the mixture for an effective control of size distribution and particle shape.
Acknowledgements
The authors thank LME-LNLS (Campinas-SP-Brazil) for HRTEM images and CNPq/Brazil for financial support.
References
- Bao , Y , Beerman , M and Krishnan , KM . 2003 . Letter to the editor: Controlled self-assembly of colloidal cobalt nanocrystals . J. Magn. Magn. Mater. , 266 : L245 – L249 . doi: 10.1016/S0304-8853(03)00649-8
- Puntes , VF and Krishnan , KM . 2001 . Synthesis, structural order and magnetic behavior of self-assembled ε-Co nanocrystal arrays . IEEE Trans. Magn. , 37 ( 4 ) : 2210 – 2212 . doi: 10.1109/20.951126
- Aiken , JD III and Finke , RG . 1999 . A review of modern transition-metal nanoclusters: Their synthesis, characterization, and applications in catalysis . J. Mol. Catal. A Chem. , 145 : 1 – 44 . doi: 10.1016/S1381-1169(99)00098-9
- Bezemer , GL , Bitter , JH , Kuipers , HPCE , Oosterbeek , H , Holewijn , JE , Xu , X , Kapteijn , F , van Dillen , AJ and de Jong , KP . 2006 . Cobalt particle size effects in the Fischer–Tropsch reaction studied with carbon nanofiber supported catalysts . J. Am. Chem. Soc. , 128 ( 12 ) : 3956 – 3964 . doi: 10.1021/ja058282w
- Sun , S and Murray , CB . 1999 . Synthesis of monodisperse cobalt nanocrystals and their assembly into magnetic superlattices (invited) . J. Appl. Phys. , 85 ( 8 ) : 4325 – 4330 . doi: 10.1063/1.370357
- Zhao , Y-W , Zheng , RK , Zhang , XX and Xiao , JQ . 2003 . A simple method to prepare uniform cobalt nanoparticles . IEEE Trans. Magn. , 39 ( 5 ) : 2764 – 2766 . doi: 10.1109/TMAG.2003.815592
- Kobayashi , YM , Horie , M , Konno , M , Rodriguez-Gonzalez , B and Liz-Marzan , LM . 2003 . Preparation and properties of silica-coated cobalt nanoparticles . J. Phys. Chem. B , 107 ( 30 ) : 7420 – 7425 . doi: 10.1021/jp027759c
- Wu , N , Fu , L , Su , M , Aslam , M , Wong , KC and Dravid , VP . 2004 . Interaction of fatty acid monolayers with cobalt nanoparticles . Nano Lett. , 4 ( 2 ) : 383 – 386 . doi: 10.1021/nl035139x
- Su , YK , Shen , CM , Yang , TZ , Yang , HT , Gao , HJ and Li , HL . 2005 . The dependence of Co nanoparticles sizes on the ratio of surfactants and the influence of different crystal sizes on magnetic properties . Appl. Phys. A , 81 : 569 – 572 . doi: 10.1007/s00339-004-2713-z
- Martínez , A and Prieto , G . 2007 . Breaking the dispersion-reducibility dependence in oxide-supported cobalt nanoparticles . J. Catal. , 245 : 470 – 476 . doi: 10.1016/j.jcat.2006.11.002
- Martínez , A and Prieto , G . 2007 . The key role of support surface tuning during the preparation of catalysts from reverse micellar-synthesized metal nanoparticles . Catal. Commun. , 8 : 1479 – 1486 . doi: 10.1016/j.catcom.2006.12.025
- Osuna , J , Decaro , D , Amiens , C , Chaudret , B , Snoeck , E , Respaud , M , Broto , JM and Fert , A . 1996 . Synthesis, characterization, and magnetic properties of cobalt nanoparticles from an organometallic precursor . J. Phys. Chem. , 100 ( 35 ) : 14571 – 14574 . doi: 10.1021/jp961086e
- Dassenoy , F , Casanove , MJ , Lecante , P , Verelst , M , Snoeck , E , Mosset , A , Ely , TO , Amiens , C and Chaudret , B . 2000 . Experimental evidence of structural evolution in ultrafine cobalt particles stabilized in different polymers – from a polytetrahedral arrangement to the hexagonal structure . J. Chem. Phys. , 112 ( 18 ) : 8137 – 8145 . doi: 10.1063/1.481414
- Puntes , VF , Krishnan , KM and Alivisatos , AP . 2001 . Synthesis, self-assembly, and magnetic behavior of a two-dimensional superlattice of single-crystal ε-Co nanoparticles . Appl. Phys. Lett. , 78 ( 15 ) : 2187 – 2189 . doi: 10.1063/1.1362333
- Puntes , VF , Krishnan , K and Alivisatos , AP . 2002 . Synthesis of colloidal cobalt nanoparticles with controlled size and shapes . Top. Catal. , 19 ( 2 ) : 145 – 148 . doi: 10.1023/A:1015252904412
- Ribeiro , RU , Liberatori , JWC , Winnishofer , H , Bueno , JMC , Zanchet , D and Zanchet , D . 2009 . Colloidal Co nanoparticles supported on SiO2: Synthesis, characterization and catalytic properties for steam reforming of ethanol . Appl. Catal. B Environ. , 91 : 670 – 678 . doi: 10.1016/j.apcatb.2009.07.009
- Murray , CB , Sun , S , Gaschler , W , Doyle , H , Betley , TA and Kagan , CR . 2001 . Colloidal synthesis of nanocrystals and nanocrystal superlattices . IBM J. Res. Dev. , 45 ( 1 ) : 47 – 56 . doi: 10.1147/rd.451.0047
- Lisiecki , I . 2005 . Size, shape, and structural control of metallic nanocrystals . J. Phys. Chem. B , 109 ( 25 ) : 12231 – 12244 . doi: 10.1021/jp058018p
- Wang , C , Fang , J , He , J and O’Connor , CJ . 2003 . Synthesis of one-dimensional magnetic Co nanoparticles in a novel solution system . J. Colloid Interf. Sci. , 259 : 411 – 413 . doi: 10.1016/S0021-9797(02)00182-0
- Cha , SI , Mo , CH , Kim , KT and Hong , SH . 2005 . Ferromagnetic cobalt nanodots, nanorices, nanowires and nanoflowers by polyol process . J. Mater. Res. , 20 ( 8 ) : 2148 – 2153 . doi: 10.1557/JMR.2005.0267
- Puntes , VF , Zanchet , D , Erdonmez , CK and Alivisatos , AP . 2002 . Synthesis of hcp-Co nanodisks . J. Am. Chem. Soc. , 124 ( 43 ) : 12874 – 12880 . doi: 10.1021/ja027262g
- Chakroune , N , Viau , G , Ricolleau , C , Fiévet-Vincent , F and Fiévet , F . 2003 . Cobalt-based anisotropic particles prepared by the polyol process . J. Mater. Chem. , 13 : 312 – 318 . doi: 10.1039/b209383a
- Wilcoxon , JP and Abrams , BL . 2006 . Synthesis, structure and properties of metal nanoclusters . Chem. Soc. Rev. , 35 : 1162 – 1194 . doi: 10.1039/b517312b
- Rotstein , HG and Tannenbaum , R . 2002 . Cluster coagulation and growth by surface interactions with polymers . J. Phys. Chem. B , 106 : 146 – 151 . doi: 10.1021/jp012784o
- Murray , CB , Sun , S , Doyle , H and Betley , T . 2001 . Monodisperse 3d transition-metal (Co, Ni, Fe) nanoparticles and their assembly into nanoparticle superlattices . MRS Bull , : 985 – 991 . doi: 10.1557/mrs2001.254
- Glavee , GN , Klabunde , KJ , Sorensen , CM and Hadjipanayis , GC . 1993 . Sodium borohydride reduction of cobalt ions in nonaqueous media. Formation of ultrafine particles (nanoscale) of cobalt metal . Inorg. Chem. , 32 : 474 – 477 . doi: 10.1021/ic00056a022
- Glavee , GN , Klabunde , KJ , Sorensen , CM and Hadjipanayis , GC . 1993 . Borohydride reduction of cobalt ions in water. Chemistry leading to nanoscale metal, boride, or borate particles . Langmuir , 8 : 771 – 773 . doi: 10.1021/la00039a008
- Lisiecki , I and Pileni , MP . 2003 . Synthesis of well-defined and low size distribution cobalt nanocrystals: The limited influence of reverse micelles . Langmuir , 19 : 9486 – 9489 . doi: 10.1021/la0301386