Abstract
The wide use in various fields and the great potentials in biomedical applications of carbon nanotubes highlight the need to study their toxicity and biocompatibility for recent years. This work aimed to investigate the cytotoxicity of carbon nanotubes on human embryonic lung fibroblast cells and their inter-related affecting factors. Three carboxyl modified carbon nanotubes, short carboxyl single-walled carbon nanotubes (SWCNTs-COOH), short carboxyl double-walled carbon nanotubes (DWCNTs-COOH) and short carboxyl multi-walled carbon nanotubes (MWCNTs-COOH) were chosen as subjects for the evaluation of carbon nanotubes cytotoxity. Different concentrations of carboxyl carbon nanotubes were incubated with human embryonic lung fibroblast (HELF) cells for 48 h, respectively, and the electron microscopy was used to observe the cell growth and morphology. The results showed that MWCNTs-COOH, which had a better dispersion in water was much more cytotoxic than the other two carbon nanotubes. From Cell Counting Kit-8 assay and acridine orange staining, MWCNTs-COOH could inhibit the cell growth and induce cell apoptosis with a dose–effect relationship and oxidative stress may be one of the mechanisms.
1. Introduction
Carbon nanotubes (CNTs), with their unique mechanical, physical and chemical properties Citation1,Citation2, have been the hotspot research in the chemistry, physics, biology and materials areas and show a good prospect in biomedical fields Citation3–7. Serious concerns have been raised about the positive and negative impacts of nano-materials including carbon nanotubes on the environment and human/animals. Past researches have proved that CNTs could enter human/animal bodies through the skin or permeate lung–blood barrier in a way of simple diffusion or penetration, and distribute and deposit in main organs of bodies Citation8–10.
The cellular biological effects of nanomaterials are the basis for comprehending their biological effects (positive and negative). As for carbon nanotubes, it has been reported that they exhibited certain cytotoxicity and could cause a variety of cell apoptosis and ultrastructural changes of cells Citation11–14. Besides their own physical and chemical properties, there are many other factors that may affect the toxicity of carbon nanotubes such as concentration, length, diameter, dispersion, surface modification, layers of grapheme, size, specific surface area, etc. Citation13,Citation15–18. Although previous research about various factors that may affect toxicity of carbon nanotubes has made great progress, due to the diversity of the toxicity evaluation methods, cell lines models, the preparation and purification of carbon nanotubes and other possible reasons, the results of the affecting factors of carbon nanotubes cytotoxicity and their lack of comparability still remain controversial, and the proportion of each factor in the toxicity of carbon nanotubes need to be further studied.
For similar reasons, the mechanisms of carbon nanotubes cytotoxicity thus far also remain unclear. In general, there are several mechanisms for the explanation of carbon nanotubes cytotoxicity, such as the physical interaction between carbon nanotubes and cells, the oxidative stress induced by metallic impurities, the integrity disruption of the cell membranes caused by the cell uptake through the mobility of lipid bilayers Citation19–21.
Due to the poor solubility in water, the application of carbon nanotubes are subject to certain restrictions, and surface modification is an effective way to increase their dispersion stability and solubility Citation22,Citation23. So far, the study of negative effects of carbon nanotubes mainly focused on the in vitro and in vivo toxicity of pristine carbon nanotubes, while relatively little attention was given to the research on the biological effects of water-soluble carbon nanotubes. In this work, in order to better understand water-soluble carbon nanotubes cytotoxicity and inter-related factors, human embryonic lung fibroblast (HELF) cells served as research target, we investigated the interaction between three carboxyl modified carbon nanotubes and HELF cells by morphological observation, CCK-8 analysis and acridine orange (AO) staining. The possible mechanism was also discussed. Experimental results showed that dispersion in water played an important role in cytotoxicity of carbon nanotubes. The better the dispersion in water the carbon nanotubes had, the greater the toxicity they displayed. MWCNTs-COOH can destroy the membrane and nucleolus of HELF cells and oxidative stress may be one of the mechanisms.
2. Materials and methods
2.1. Materials
Three carbon nanotubes were purchased from Chengdu Organic Chemical Co. Ltd., Chinese Academy of Sciences (Sichuan, China). The HELF cells were obtained from China Center for Type Culture Collection (Wuhan, China). Penicillin/Streptomycin solution (10000 U penicillin/10 mg/mL streptomycin), Trypsin (with EDTA) and RPMI-1640 medium (with 2.05 mM L-Glutamine) were purchased from Hyclone (Life Technologies, USA). Foetal calf serum was purchased from Hangzhou Sijiqing Biological Engineering Materials Co., Ltd (Zhejiang, China). The 6- and 96-well cell culture plates were purchased from Corning Life Sciences (USA). Cell Counting Kit-8 was purchased from Dojindo Laboratories (Kumamoto, Japan). Commercial kits of glutathione peroxidase (GSH-Px), superoxide dismutase (SOD) and malondialdehyde (MDA) were purchased from Nanjing Jiancheng Bioengineering Institute (Nanjing, China). AO was purchased from Ameresco (USA). PBS buffer pre-mixed reagents (pH 7.2∼7.4) was obtained from Zhongshan Goldenbridge Biotechnology Co. Ltd (Beijing, China). All solutions were prepared with 18.3 MΩ distilled water (Millipore, USA).
2.2. Instruments
CO2 incubator (Forma Scientific, USA) was used for the cell culture. Inverted microscope (Olympus IX70 112, Japan) was used to observe the cell morphology. JY 92-II Ultrasonic cell disruption apparatus (Ningbo Xinyi Biotechnology Co. Ltd, China) was used to disrupt the cells. UV-1101 spectrophotometer (Shimadzu, Japan) was used to determine SOD, GSH-Px activities and MDA contents. Microplate spectrophotometer (Thermo Labsystems, USA) was used to measure cell viability.
2.3. Preparation of carbon nanotubes suspensions
Three carbon nanotubes suspensions were obtained using ultrasonic method. Each carbon nanotubes (0.0050 g) were mixed with PBS solution (5 mL), and then sterilized at 121°C for 30 min. The resulting nanotubes suspensions were stored in a 4°C refrigerator and dispersed by ultrasound for 30 min before using.
2.4. Cell culture and CCK-8 assay
The HELF cells were cultured in RPMI-1640 medium supplemented with 10% foetal calf serum and 1% Penicillin/Streptomycin solution, at 37°C in 5% CO2 humidified incubator. The medium was exchanged every other day and the cells were passaged every 4 days. Different concentrations of carbon nanotubes were added into the 6- or 96-well cell culture plates and HELF cells without CNTs were used as control. The cell growth and apoptosis were observed by electron microscopy to evaluate the cytotoxicity of different carbon nanotubes. Cell Counting Kit-8 was applied to evaluate the effect of carbon nanotubes on HELF cells viability. The cell viability was calculated by the following formula: cell viability (%) = (optical density [OD] of the treated cells−OD without cells)/(OD of non-treated cells−OD without cells).
2.5. AO staining
The HELF cells were seeded to a 6-well plate pre-placed cover slip on the bottom of each well and then incubated with different concentrations of carbon nanotubes for 48 h. Aspirate the culture medium, add 1 mL PBS to each well and then rinse the wells twice. Aspirate the PBS solutions and add 1 mL of 95% ethanol to each well. After 15 min, aspirate the ethanol and let the wells air-dry. Add sufficient amount of 0.01% AO to the cover slips, stain for 10 min and then rinse the wells with 1 mL PBS twice. Place a drop of PBS in the center of the microscope slide, take the treated cover slip over the slide and then observe the cells with a blue–violet excitation filter.
2.6. Determination of SOD and GSH-Px activities and MDA contents
Aspirate the culture medium, add 1 mL PBS to each well and then rinse the wells twice. Use a rubber cell scraper to scrape the cells gently from the plates and disrupt the cells by ultrasonic cell disruption apparatus. The determination of SOD and GSH-Px activities and MDA contents was carried out according to the instructions of the kits.
3. Results and discussion
In order to understand the factors affecting carbon nanotubes cytotoxicity, the interaction between HELF cells and the three hydroxyl carbon nanotubes with different structural characteristics was investigated.
3.1. Changes of HELF cells morphology after exposure to MWCNTs-COOH
showed the changes of HELF cells morphology after exposure to different concentrations of MWCNTs-COOH.
Figure 1. Changes of HELF cells morphology after exposure to MWCNTs-COOH. Concentrations of MWCNTs-COOH: (a) 0 µg/mL, (b) 1.25 µg/mL, (c) 2.5 µg/mL, (d) 10.0 µg/mL, (e) 30.0 µg/mL, (f) 50.0 µg/mL.
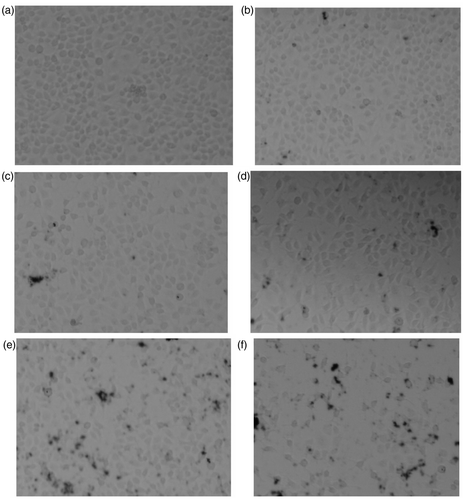
After 48 h of incubation, the HELF cells of control group showed ovoid and polygonal in mosaic arrangement (). No significant changes of cell morphology were observed when exposed to 1.25 and 2.5 µg/mL MWCNTs-COOH. When the concentration of MWCNTs-COOH was increased to 10.0 and 30.0 µg/mL, cell necrosis occurred and dead cells appeared in the culture medium. Pseudopods of some cells were prolonged, more pseudopods appeared from some cells and the cell gap was increased. When the concentration of MWCNTs-COOH was increased to 50.0 µg/mL, the number of dead cells increased significantly, and the morphology of living cells was further changed and even showed irregular shapes. The results showed that 10.0 µg/mL of MWCNTs-COOH showed toxicity on HELF cells with a dose–effect relation.
3.2. Changes of HELF cells’ morphology after exposure to DWCNTs-COOH
From it can be seen that most of the DWCNTs-COOH gathered and floated in the cell culture medium. After 48 h of incubation, no significant changes of cell morphology were observed when exposed to DWCNTs-COOH in the range of 0–50 µg/mL, indicating that MWCNTs-COOH at concentrations of 0–50 µg/mL showed no apparent toxicity to HELF cells.
3.3. Changes of HELF cells morphology after exposure to SWCNTs-COOH
From , similar to DWCNTs-COOH, SWCNTs-COOH also gathered and floated in the cell culture medium, and no significant changes of cell morphology were observed when exposed to SWCNTs-COOH in the range of 0–50 µg/mL after 48 h of incubation with SWCNTs-COOH. The results indicated SWCNTs-COOH at concentrations of 0–50 ug/mL also showed no apparent toxicity to HELF cells.
3.4. Discussion of cytotoxicity of three carboxyl carbon nanotubes
The structural characteristics of three carboxyl carbon nanotubes used in this work were described in . Their inner diameters (OD), carboxyl contents (–COOH content) and lengths were basically at the same levels and the biggest difference among them was the layers of graphene sheets. Since the carboxyl carbon nanotubes were dispersed in PBS solution, the pH values of them were close to each other.
Table 1. The structural characteristics of three carboxyl carbon nanotubes.
From Figures , it can be seen that the aggregation in water of three carboxyl carbon nanotubes was in the order of MWCNTs-COOH < DWCNTs-COOH ≈ SWCNTs-COOH, while at the same concentrations (0–50 µg/mL), the toxicity to HELF cells of them was in the order of MWCNTs-COOH > DWCNTs-COOH ≈ SWCNTs-COOH. They differed greatly in aggregation even if their differences in OD, -COOH content and length were not significant. According to the above results, we speculated that aggregation played an important role in the cytotoxicity of carboxyl carbon nanotubes. The smaller the aggregation, the greater the carbon nanotubes cytotoxicity.
There maybe two reasons for the results. First, the carbon nanotubes, which had a lower aggregation in aqueous solution could easily contact the cells and thus showed certain cytotoxicity, suggesting that physical interaction between carbon nanotubes and cell membrane may lead to the cell death. Second, when different carbon nanotubes were added into the cell culture mediums, most of the carbon nanotubes, which had a higher aggregation in aqueous solution aggregated and floated in the cell culture medium, whereas the concentration of those, which could directly contact the cells decreased, and thus a lower cytotoxicity was demonstrated.
In addition, the mechanisms of exogenous substances entering into cells mainly include energy-dependent endocytosis and energy-independent passive diffusion, the latter of which is closely related to the size of the exogenous substances. The smaller the size of exogenous substance is, the more easily passive diffusion occurs. In this work, carboxyl carbon nanotubes with a lower aggregation exhibited significant cytotoxicity, suggesting its way of entering cells was related to the size, and thus we presumed that passive diffusion may be one of the ways of carbon nanotubes entering the cells.
There is a conflict between previous report Citation17 and our experimental results on the effect of aggregation on the carbon nanotubes cytotoxicity, which may be due to different methods used or others.
3.5. The cell viability measured by Cell Counting Kit-8 (CCK-8)
As an upgrade alternative for the evaluation of cell viability, compared with the regular MTT assay, the CCK-8 assay has the advantage of higher sensitivity, better solubility and stability, and it can avoid the occasional false-positive phenomenon caused by the absorption of formazan to carbon nanotubes. According to , among three carboxyl carbon nanotubes, the MWCNTs-COOH exhibit significant toxicity to HELF cells. To further analyze the toxic effects of MWCNTs-COOH on HELF cells, we used the CCK-8 assay to study the change of cell viability, and the results were shown in .
showed the changes of HELF cells viability after incubated with MWCNTs-COOH for 48 h. With the increasing concentrations of MWCNTs-COOH in the culture medium, the cell viability decreased, especially in the range of 0–50 µg/mL, there was a linear relationship between them. When the MWCNTs-COOH concentration was increased to 50 µg/mL, the cell viability was reduced to about 12% of the control group. With further increasing concentrations of MWCNTs-COOH, the cell viability showed relatively slow decrease. The results indicated that MWCNTs-COOH could inhibit the cell growth and induce cell apoptosis.
3.6. AO staining of HELF cells
AO is a widely used vital dye and can stain both live and dead cells. It can permeate cells and bind DNA and RNA to give two fluorescences of green and red, from which we could get some information of cell apoptosis caused by exogenous substances. In this work, after incubation with different concentrations of MWCNTs-COOH for 48 h, the fluorescence staining in HELF cells are shown in .
Figure 5. Changes of HELF cells morphology (dyed by AO) after exposure to MWCNTs-COOH. Concentrations of MWCNTs-COOH: (a) 0 µg/mL, (b) 5.0 µg/mL, (c) 20.0 µg/mL, (d) 50.0 µg/mL, (e) 100.0 µg/mL, (f) 200.0 µg/mL.
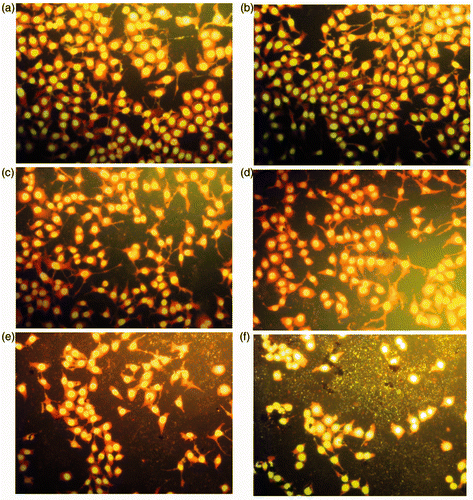
As can be seen from , after AO staining, the nuclei of HELF cells emitted bright green fluorescence and the cytoplasm emitted bright red fluorescence. The cell morphology of control group () was integrity, and the nuclei were round. With the increasing concentrations of MWCNTs-COOH in the culture medium, the number of cells, which were capable of emitting fluorescent decreased, indicating that the number of dead cells gradually increased.
In addition, when the concentration of MWCNTs-COOH increased to 100.0 µg/mL, nuclear chromatin condensation could be observed, and the fluorescent fragments appeared around the cells, indicating the cell membranes and nuclei were destroyed and the DNAs leaked. It was prompted that MWCNTs-COOH might penetrate the cells and even the nuclei.
3.7. Determination of SOD and GSH-Px activities and MDA levels in cells
The SOD and GSH-Px activities and malondialdehyde (MDA) content were commonly used indicators of oxidative stress. The SOD activity can indirectly reflect the level of the body's/cell's ability to scavenge oxygen-free radicals. GSH-Px activity can be used to assess the extent of oxygen-free radical-induced lipid peroxidation. MDA is an important indicator which can reflect the extent of free radical-induced lipid peroxidation and damage to bodies/cells.
Previous researches have proved that some carbon nanotubes could cause oxidative injury in many cells. To verify if oxidative stress was included in the mechanisms of MWCNTs-COOH cytotoxicity, after incubated with different concentrations of MWCNTs-COOH, the changes of SOD and GSH-Px activities and the contents of MDA in cells were determined and the results are shown in .
Table 2. The levels of SOD, GSH-Px and MDA in cells ( x ± s, n = 6).
As can be seen from , with the increasing concentrations of MWCNTs-COOH in culture medium, the SOD, GSH-Px activities of cells had a trend of decrease and the MDA contents increased gradually. Compared with the control group, especially when the concentrations of MWCNTs-COOH were 50,100 and 200 µg/mL, the SOD and GSH-Px activities decreased significantly with a dose–response relationship. Meanwhile, the content of MDA increased significantly with a dose–response relationship. The results showed that oxidative stress might play an important role in the MWCNTs-COOH cytotoxicity.
4. Conclusions
In short, among the three carboxyl carbon nanotubes, MWCNTs-COOH which had a better dispersion in aqueous solution showed more significant cytotoxicity, suggesting that dispersion and toxicity should be both considered in the biomedical applications of carbon nanotubes. MWCNTs-COOH can inhibit the cell growth and induce cell apoptosis with a dose–response relationship. In the process of cell apoptosis, the chromatin condensation occurred and the cell membrane and nucleus were destroyed. MWCNTs-COOH might enter the nuclei, and oxidative stress might be one of the mechanisms of its cytotoxicity.
So far, researches on carbon nanotubes toxicity still remain controversial. Due to the many factors that may affect the toxicity of carbon nanotubes, it is important for researchers to choose types of carbon nanotubes. They should choose sufficient number of different structures of carbon nanotubes to guarantee that they can rule out other factors and study only one single factor how to affect the carbon nanotube toxicity. In addition, in order to ensure the accuracy of the results, different toxicity testing methods should be combined for mutual authentication.
As this work concentrated on the cell damage induced by extracellular concentrations of carbon nanotube, the information about interaction inside cells and the MWCNT-COOH location in the cells was obtained mainly through speculating from experimental results, and intuitionistic observation is lacking. Further studies, including more types of cells and carbon nanotubes, more research indicators and methods are needed to clarify the carbon nanotubes’ cellular biological effects.
Acknowledgements
The authors acknowledge the considerable support of China Post-doctoral Science Foundation funded project (20090461214) and Innovation Fund for the Post-Doctoral Program of Shandong Province (200902015).
References
- O’Connell , M J , Bachilo , S M and Huffman , C B . 2002 . Band gap fluorescence from individual single-walled carbon nanotubes . Science , 297 : 593 – 596 .
- Dai , H . 2002 . Carbon nanotubes: Opportunities and challenges . Surface Science , 500 : 218 – 241 .
- Bianco , A , Kostarelos , K , Partidos , C D and Prato , M . 2005 . Biomedical applications of functionalised carbon nanotubes . Chem. Commun. , : 571 – 577 .
- Kam , N WS , O’Connell , M J and Wisdom , J A . 2005 . Carbon nanotubes as multifunctional biological transporters and near-infrared agents for selective cancer cell destruction . PNAS , 102 : 11600 – 11605 .
- Gao , L , Nie , L and Wang , T . 2006 . Carbon nanotube delivery of the gfp geneinto mammalian cells . Chembiochem. , 7 : 239 – 241 .
- Veetil , J V and Ye , K . 2007 . Development of immunosensors using carbon nanotubes . Biotechnol. Prog. , 23 : 517 – 531 .
- Feazell , R P , Nakayama-Ratchford , N , Dai , H and Lippard , S J . 2007 . Soluble single-walled carbon nanotubes as longboat delivery systems for platinum(IV) anticancer drug design . J. Am. Chem. Soc. , 129 : 8438 – 8439 .
- Mitchell , L A , Gao , J , Wal , R V , Gigliotti , A , Burchiel , S W and McDonald , J D . 2007 . Pulmonary and systemic immune response to inhaled multiwalled carbon nanotubes . Toxicol. Sci. , 100 : 203 – 214 .
- Deng , X , Jia , G , Wang , H , Sun , H , Wang , X , Yang , S , Wang , T and Liu , Y . 2007 . Translocation and fate of multi-walled carbon nanotubes in vivo . Carbon , 45 : 1419 – 1424 .
- Bai , Y H , Zhang , Y , Zhang , J P , Mu , Q X , Zhang , W D , Butch , E R , Snyder , S E and Yan , B . 2010 . Repeated carbon nanotube administrations in male mice cause reversible testis damage without affecting fertility . Nat. Nanotechnol. , 5 : 683 – 689 .
- Tsukahara , T and Haniu , H . 2011 . Cellular cytotoxic response induced by highly purified multi-wall carbon nanotube in human lung cells . Mol. Cell. Biochem. , 352 ( 1–2 ) : 57 – 63 .
- Jia , G , Wang , H F , Yan , L , Wang , X , Pei , R J , Yan , T and Zhao , Y L . 2005 . Cytotoxicity of carbon nanomaterials: Single-wall nanotube, multi-wall nanotube, and fullerene . Environ. Sci. Technol. , 39 : 1378 – 1383 .
- Manna , S K , Sarkar , S , Barr , J , Wise , K , Barrera , E V , Jejelowo , O , Rice-Ficht , A C and Ramesh , G T . 2005 . Single-walled carbon nanotube induces oxidative stress and activates nuclear transcription factor kappa B in human keratinocytes . Nano Lett. , 5 : 1676 – 1684 .
- Monteiro-Riviere , N A , Nemanich , R J , Inman , A O , Wang , Y Y and Riviere , J E . 2005 . Multi-walled carbon nanotube interactions with human epidermal keratinocytes . Toxicol. Lett. , 155 : 377 – 384 .
- Bottini , M , Bruckner , S , Nika , K , Bottini , N , Bellucci , S , Magrini , A , Bergamaschi , A and Mustelin , T . 2006 . Multi-walled carbon nanotubes induce T lymphocyte apoptosis . Toxicol. Lett. , 160 : 121 – 126 .
- Becker , M L , Fagan , J A and Gallant , N D . 2007 . Length-dependent uptake of DNA-wrapped single-walled carbonnanotubes . Adv. Mater. , 19 : 939 – 945 .
- Wick , P , Manser , P , Limbach , L K , Dettlaff-Weglikowska , U , Krumeich , F , Roth , S , Stark , W J and Bruinink , A . 2007 . The degree and kind of agglomeration affect carbon nanctube cytotoxicity . Toxcol. Lett. , 168 : 12l – 13l .
- Yan , L , Zhao , F , Li , S , Hu , Z and Zhao , Y . 2011 . Low-toxic and safe nanomaterials by surface-chemical design, carbon nanotubes, fullerenes, metallofullerenes, and graphenes . Nanoscale , 3 : 362 – 382 .
- Pulskamp , K , Diabat , E S and Krug , H F . 2007 . Carbon nanctubes show no sign of acute toxicity but induce intracellular reactive oxygen species in dependence on contaminants . Toxicol. Lett. , 168 : 58 – 74 .
- Garibaldi , S , Brunelli , C , Bavastrello , V , Ghigliotti , G and Nicolini , C . 2006 . Carbon nanotube biocompatibility with cardiac muscle cells . Nanotechnology , 17 : 391 – 397 .
- Nimmagadda , A , Thurston , K , Nollert , M U and McFetridge , P S . 2006 . Chemical modification of SWNT alters in vitro cell-SWNT interactions . J. Biomed. Mater. Res. A , 76 ( 3 ) : 614 – 625 .
- Lee , J , Kim , M , Hong , C K and Shim , S E . 2007 . Measurement of the dispersion stability of pristine and surface-modified multiwalled carbon nanotubes in various nonpolar and polar solvents . Meas. Sci. Technol. , 18 : 3707 – 3712 .
- Iijima , M and Kamiya , H . 2009 . Surface modification for improving the stability of nanoparticles in liquid media . KONA Powder Particle J. , 27 : 119 – 129 .