Abstract
Titanium dioxide nanocrystals are prepared by a hydrolysis process of Titanium tetraisopropoxide. Nano crystals of various sizes are obtained after annealing from 300 to 450°C. Raman scattering was employed to investigate the evolution of the anatase phase in the nano crystals during annealing. X-ray diffraction, transmission electron microscopy and dynamic light scattering studies were done to supplement the Raman scattering results on particle size. Phonon confinement and non-stoichiometry effects are responsible for the blue shift and broadening of the lowest-frequency Eg Raman mode.
1. Introduction
Nanophase materials have been attracting great interest for several years because of their unique mechanical, electrical, optical, biological and magnetic properties [Citation1]. The properties of nanophase materials are strongly related to their structure, therefore the structure of nanophase materials is studied on a variety of length scales in order to develop an understanding of the structure–property relations [Citation2]. Titanium dioxide (TiO2) is a main component of paints, pigments, a variety of glass products, biomedical implants and in cosmetics [Citation3,Citation4]. TiO2 exists in three natural phases. They are anatase, rutile, and brookite. Anatase is tetragonal () with two formula units per unit cell and six Raman active modes (A
1
g
+ 2B
1
g
+ 3Eg
), whereas rutile ∼tetragonal,
) has two units and four Raman active modes (A
1
g
+ B
1
g
+ B
2
g
+ Eg
). Brookite is orthorhombic (
), has eight formula units per unit cell and shows 36 Raman active modes (9A
1
g + 9B
1
g + 9B
2
g + 9B
3
g
) [Citation5]. The phonons are confined in nanocrystals. In the bulk material Raman modes obey the fundamental selection rule
= 0, where
is the wave vector of the scattered phonon. In finite domains this selection rule is relaxed. All phonon modes with
π/d, where d is the dimension of the crystalline domine, contribute to the Raman intensity [Citation6]. An important property of nanocrystals is the appearance of surface phonon modes. In the frequency range between bulk longitudinal optical phonon frequency, ω
LO transverse optical mode frequency, ω
TO a new mode know as surface phonon mode, appears in the nanocrystals, which is absent in corresponding bulk material [Citation7].
Fabrication techniques and the processing conditions have a strong influence on the microstructures and electronic and vibrational properties of the materials. Changes in the Raman spectra TiO2 nanocrystals were related to non-stoichiometry, surface pressure and the phonon confinement effect [Citation8–11]. In this study, we investigate the Raman spectra of TiO2 nanocrystals at various annealing temperatures and particle sizes in order to get a better understanding of the lattice vibration characters in ultrafine particle TiO2 systems. Particle size were also studied by X-ray diffraction (XRD), transmission electron microscopy (TEM) and dynamic light scattering (DLS) to confirm the results of Raman scattering.
2. Experimental procedure
2.1. Synthesis of TiO2 nanoparticles
A 7.4 ml of titanium tetraisopropoxide was added, drop by drop, to 30 ml of 1 M HNO3 aqueous solution, and then agitated for 2 h to give a transparent sol, in which 2.0 g TiO2 are contained. The pH of the colloidal solution was adjusted to pH 3, with the addition of 1 M NaOH solution after dilution of the colloid with 100 ml water, resulting in a turbid TiO2 colloid. The suspension was agitated at room temperature, centrifuged and then washed with distilled water. The isolated TiO2 was dried for 1 h at 100°C in air. The resulting powder was then calcinated at 300, 350, 400, 450°C for 3 h [Citation12,Citation13].
The micro-Raman spectra were recorded at room temperature using a laser Raman confocal microprobe (LabRam HR 800). A He–Ne laser (λ = 633 nm) was used as the excitation source with an output power of 17 mW and was focused to a spot of 1 µm. Before measurement, the system was calibrated using the 521 cm−1 Raman line of a silicon (Si) wafer. The confocal hole of the spectrograph was 100 µmin diameter. All Raman spectra were recorded in the back scattering geometry. Powder XRD (Seifert, JSO-DE BYEFLEX 2002, Germany) was utilised to identify the crystalline phase composition. The morphology and crystal structure of the product were observed by TEM. The instrument was JEOL 2000Fx-II operated at 200 kV, high resolution, analytical TEM with a W-source and a point–point resolution of 2 Å. Particle size distribution is analysed by Zeta sizer Nano S90 series.
3. Results and discussion
Raman spectra of TiO2 samples annealed at different temperatures are given in . In the spectra of all TiO2 samples the dominant Raman modes can be assigned to the Raman active modes of the anatase crystal 144 (Eg (1)), 197 (Eg (2)), 399 (B 1 g (1)), 519 (combination of A 1 g and B 1 g (2) that cannot be resolved at room temperature) and 639 cm−1 (Eg (3)) [Citation14]. The dependence of the shape of the Raman peaks from the crystal dimension may have various explanations. A pressure effect on the grains, induced by the surrounding grains or by the surface tension, has been often invoked in similar cases. This mechanism usually gives large frequency shifts and, moreover, Raman measurements as a function of the external pressure show a behaviour, for some of the peaks, which is opposite that observed here. Therefore, we believe that the changes in the Raman line widths have a different origin. A possible cause of the Raman line broadening could be a non-stoichiometry of the sample i.e. an oxygen deficiency or the disorder induced by minor phases, which in titania samples obtained with other preparation methods play an important role. In our case, the thermal treatments are performed in air and the non-stoichiometry is expected to be negligible. In addition, Raman and XRD measurements suggest that only the anatase phase is present [Citation15].
Therefore, we attempt to explain the observed line broadening with the breakdown of the phonon momentum selection rule q = 0, specific of the Raman scattering in ordered systems. In the case of crystals of very small size, this rule is no longer valid as the phonons are confined in space and all the phonons over the Brillouin zone will contribute to the first order Raman spectra. The weight of the off-center phonons increases as the crystal size decreases and the phonon dispersion causes an asymmetrical broadening and the shift of the Raman peaks. A simple confinement model may then be used to calculate the shape of the 144 cm−1 mode of the anatase at various nano crystal sizes. For spherical nano crystals and first-order scattering, the Raman intensity I(ω) can be written as
By taking a spherical Brillouin zone and an isotropic dispersion curve, the dispersion relationship for the Eg Raman mode in the anatase phase could be expressed as [Citation16]
shows the Raman intensity calculated using phonon confinement model for different crystal sizes (diameter 6, 6.5, 7, 8.25 and 11 nm). As the crystallite size decreases, the frequency and line width of the Eg Raman peak blue shift and increase, respectively. At the crystallite size of 6 nm, the Raman peak exhibits a significant asymmetric broadening. Obviously, the calculated results based on the phonon confinement model are in qualitative agreement with our experimental data. The main Eg Raman modes of the TiO2 nano crystals annealed at various temperatures are displayed in . The broadening of the peak becomes important only for the smallest crystals, when the confinement involves the phonons far from the zone center. When the size is <3–4 nm the model cannot be used, as details of the dispersion curve and of the shape of the Brillouin zone becomes critical. The calculated broadening and blue shift of the Raman peak fairly describe those observed in nano crystals with different sizes. This indicates that the phonon confinement is the dominant mechanism responsible for the blue shift of the Raman peak in small-sized nano crystals, and the non-stoichiometry has only a little influence on the blue shift.
Figure 2. (Colour online) Raman intensity calculated using phonon confinement model for different crystal sizes.
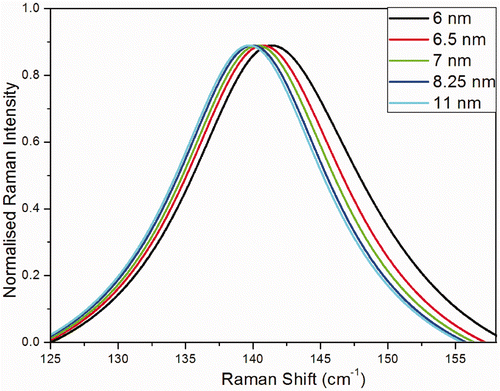
Figure 3. (Colour online) Raman modes Eg of the TiO2 nanoparticles annealed at various temperatures.
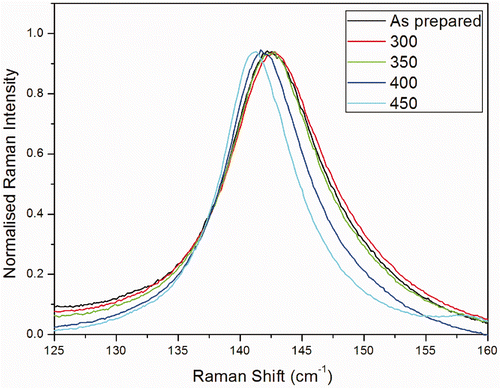
TiO2 samples were annealed at various temperatures for 3 h. shows the X-ray diffraction patterns for TiO2 as a function of annealing temperature. The diffraction peaks corresponds to anatase phase. From 300 to 450°C only peaks related to anatase structure were evident. Existence of strong crystalline peaks at 2θ values of 25.20°, 37.80°, 48.04°, 53.890° and 62.68° corresponding to the crystal planes of (101), (004), (200), (105) and (204) indicates the formation of anatase TiO2 (JCPDS card No. 21-1272). As the annealing temperature increases above 300°C the intensity of (101) peak increases and the line width of the same decreases. This indicates that after annealing, the crystallinity of the sample becomes better and the crystallite size increases.
Figure 4. XRD images of TiO2 nanoparticles annealed at different temperatures: (a) as prepared; (b) annealed at 300°C; (c) annealed at 350°C; (d) annealed at 400°C and (e) annealed at 450°C.
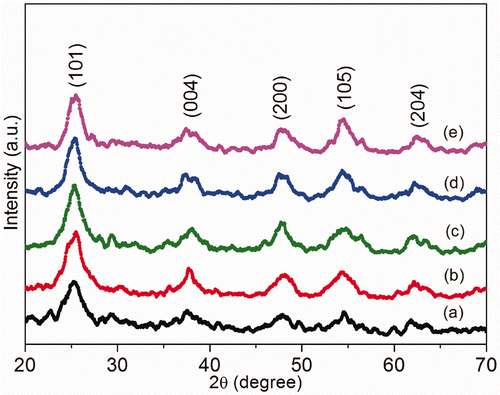
The TEM images of TiO2 nanoparticles annealed for 3 h at different temperature are given in . TEM images confirm the formation of spherical particles with uniform particle size. It is clearly seen that as the annealing temperature increases the average particle size increases. The increase in the average particle size is very little up to about 450°C. This agrees well with the results of Siegel et al. [Citation17]. Grains with much agglomeration are clearly observable from the images of low temperature heat treated samples. The particle size of TiO2 nanoparticles varies from 6 to 12 nm. The SAED analyses confirmed the formation of tetragonal structure of TiO2. The particle size calculated from TEM agrees well with the particle size calculated in Raman spectroscopy.
The particle size distribution is shown in . For the as prepared TiO2, the size of particle is around 7 nm (60%). It is interesting that with the temperature rising, the size of particles increases. The size is around 8 nm (70%) at 300°C, while that is around 9 nm (75%) at °C, around 10 nm (78%) at 400°C and around 12 nm (80%) at 450°C. All the samples have a narrow size distribution.
Figure 6. Particle size distributions of TiO2 nanoparticles annealed at different temperatures analysed by DLS.
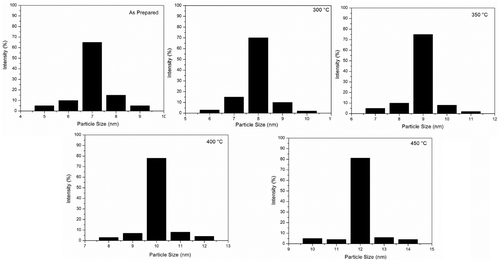
Raman spectroscopy analysis shows that the TiO2 nano crystals contain only tetragonal anatase phases. The evolution of the anatase phase in theTiO2 nano crystals can be well characterised by the change of the lowest-frequency Eg Raman mode with annealing treatment. Phonon confinement has been found to be the main cause of the broadening and the blue shift of the main Raman peak in TiO2 powders of pure anatase nano crystals. The particle size analysis by XRD, TEM and DLS is in agreement with the Raman spectroscopy analysis.
4. Conclusion
TiO2 nanocrystals have been synthesised through chemical route. XRD, TEM, and Raman spectroscopy results confirm a single-phase anatase TiO2 nano crystal. The evolution of the anatase phase in the TiO2 nano crystals can be well characterised by the change of the lowest-frequency Eg Raman mode with annealing treatment. The phonon confinement has been found to be the main cause of the broadening and the blue shift of the main Raman peak in TiO2 powders of pure anatase nano crystals. A combined mechanism involving phonon confinement and non-stoichiometry effects is proposed to well explain the blue shift and broadening of the lowest-frequency Eg Raman mode in TiO2 nano crystals. The knowledge of the phonon dispersion curve in anatase could help in a more quantitative correlation between the band shape and the crystals size. The sizes of the particles obtained from TEM, particle size distribution and Raman scattering are consistent.
Acknowledgements
S. Ramasamy, CSIR Emeritus Scientist, acknowledges the financial support given to carry out this work under CSIR Emeritus Scientist Scheme number 21(0714)/08/EMR-II dated 28-04-2008 G. Devanand Venkatasubbu, CSIR-SRF, acknowledges the financial support given to him by CSIR. The authors V.S. and V.R. are thankful to UGC-UPE programme of Madurai Kamaraj University for the Micro Raman facility. The authors are grateful to Dr. G.S. Avadhani, Department of Materials Engineering, Indian Institute of Science, Bangalore, India for his help to do TEM analysis.
References
- Gleiter , H . 1989 . Nanocrystalline materials . Prog. Mater. Sci. , 33 : 223 – 315 .
- Siegel , RW . 1994 . What do we really know about the atomic-scale structures of nanophase materials? . J. Phys. Chem. Solids. , 55 : 1097 – 1106 .
- Venz , PA , Kloprogge , JT and Frost , RL . 2000 . Chemically modified titania hydrolysates: Physical properties . Langmuir , 16 : 4962 – 4968 .
- Murugan , AV , Samuel , V and Ravi , V . 2006 . Synthesis of nanocrystalline anatase TiO2 by microwave hydrothermal method . Mater. Lett. , 60 : 479 – 480 .
- Lottici , PP , Bersani , D , Braghini , M and Montenero , A . 1993 . Raman scattering characterization of gel-derived titania glass . J. Mater. Sci. , 28 : 177 – 183 .
- Cambell , IH and Fauchet , PM . 1986 . The effects of microcrystal size and shape on the one phonon Raman spectra of crystalline semiconductors . Solid State Comm. , 58 : 739 – 741 .
- Fuchs , R and Kliewer , KL . 1968 . Optical modes of vibration in an ionic crystal sphere . Opt. Soc. Am. , 58 : 319 – 330 .
- Ma , WH , Lu , Z and Zhang , MS . 1998 . Investigation of structural transformations in nanophase titanium dioxide by Raman spectroscopy . Appl. Phys. A , 66 : 621 – 627 .
- Parker , JC and Siegel , RW . 1990 . Raman microprobe study of nanophase TiO2 and oxidation induced spectral changes . J. Mater. Res. , 5 : 1246 – 1252 .
- Parker , JC and Siegel , RW . 1990 . Calibration of Raman spectrum to the oxygen stoichiometry of nanophase TiO2 . Appl. Phys. Lett. , 57 : 943 – 945 .
- Bersani , D , Lottici , PP and Ding , XZ . 1998 . Phonon confinement effects in thee Raman scattering by TiO2 nanocrystals . Appl. Phys. Lett. , 72 : 73 – 75 .
- Robert , D and Weber , JV . 1999 . Titanium dioxide synthesis by sol–gel methods and evaluation of their photocatalytic activity . J. Mat. Sci. Lett. , 18 : 97 – 98 .
- Manivannan , A , Glaspell , G and Dutta , P . 2008 . Synthesis of nanocrystalline TiO2 particles and their structural characteristic . J. Clust. Sci. , 19 : 391 – 399 .
- Ma , W , Lu , Z and Zhang , M . 1998 . Investigation of structural transformations in nanophase titanium dioxide by Raman spectroscopy . Appl. Phys. A. , 66 : 621 – 627 .
- Zhang , WF , He , YL , Zhang , MS , Yin , Z and Chen , Q . 2000 . Raman scattering study on anatase TiO2 nanocrystals . J. Phys. D: Appl. Phys. , 33 : 912 – 916 .
- Golubovic , A , Scepanovic , M , Kremenovic , A , Askrabic , S , Berec , V , Dohcevic-Mitrovic , Z and Popovic , ZV . 2009 . Raman study of the variation in anatase structure of TiO2 nanopowders due to the changes of sol–gel synthesis conditions . J. Sol-Gel. Sci. Technol. , 49 : 311 – 319 .
- Siegel , RW , Ramasamy , S , Hahn , H , Zongquan , LI , Ting , LU and Gronsky , R . 1988 . Synthesis, characterization, and properties of nanophase TiO2 . J. Mat. Res. , 3 : 1367 – 1372 .