Abstract
Biosynthesised iron oxide nanoparticles (INPs) less than 100 nm were used to decolourise the textile dye, Direct Orange S by the process of ultra sonication. The parameters tested for the decolourisation of the Direct Orange S dye were the INP concentration (0.2–1g/l), pH (3–11) and H2O2 concentration (2–8 ml/l). The rate of decolourisation process was performed by both stirrer and ultra sonicator method using the first-order kinetics ln(c/c0). The percentage of dye removal for the optimum conditions such as INP, 1g/l (87.2%); pH, 9 (85.5%) and H2O2 concentration 8 ml/l (86.5%) were observed. The Direct Orange S dye was degraded efficiently by sonolysis (60 min) than by the magnetic stirrer method (120 min). High-performance liquid chromatography results showed that the peak for Direct Orange S dye was not present when the dye sample was treated by either H2O2 + INP or INP alone. Use of biosynthesised iron NPs in Direct Orange S dye (azo dye) decolourisation by a simple non-toxic Fenton reaction is a safe and novel approach. Industrialisation of this technique will be an economical way to decolorise the textile dyes present in water systems.
1. Introduction
Nanotechnology works with materials, devices and other structures with at least one-dimensional size from 1 to 100 nanometres.[Citation1,2] Generally, nanotechnology is the application of nanoscience to a broad set of emerging manufacturing technologies, which control and manipulate material at the level of atoms and molecules. Synthesis of nanoparticles has become a major focus nowadays like using chemicals,[Citation3,4] plant phytochemicals [Citation5,6] and microbial metabolites.[Citation7] Metal oxide nanoparticles (NPs) are extensively used in a considerable number of applications in food, material, chemical and biological sciences.[Citation8] Nowadays, metal oxide NPs are under experiments for textile dye decolourisation because the generation rate of hydroxyl radical using surface reaction is much slower than using dissolved iron ion. Furthermore, nanoscale iron is expected to be useful in the remediation processes.[Citation9]
The textile mills daily discharge millions of litres of untreated effluents in the form of wastewater into public drains that eventually empty into rivers. Most of them are recalcitrant in nature, especially azo dyes. The stability and the xenobiotic nature of reactive azo dyes makes them recalcitrant; hence, they are not totally degraded by conventional wastewater treatment processes that involve light, chemicals or activated sludge.[Citation10] The dyes are therefore released into the environment, in the form of coloured wastewater. This can lead to acute effects on exposed organisms due to the toxicity of the dyes; phytoplanktons form abnormal colouration and cause reduction in photosynthesis because of the absorbance of light that enters the water.[Citation11,12] The presence of unnatural colours is aesthetically unpleasant and tends to be associated with contamination. Without an adequate treatment, these dyes will remain in the environment for an extended period of time.[Citation13] There is a reasonable urgency for developing a treatment process which decolourises the coloured water efficiently and more economically.
Several methods were adapted for the reduction of azo dyes to achieve decolourisation. These include physiochemical methods [Citation14] such as filtration, specific coagulation, use of activated carbon, chemical flocculation, etc. Biotreatment offers a cheaper and environmentally friendlier alternative for colour removal in textile effluents.[Citation15,16] Several studies have also reported that oxidation of organic compounds using Fenton's reagent (H2O2, Fe2+) is found to be efficient in the decolourisation of organic compounds like azo dyes.[Citation17–20] One such nanoscale iron particle was used as an iron catalyst in a Fenton-like process to enhance the activation of the catalyst and surface reaction. Apart from this, sonolysis can also chemically oxidise organic pollutants. Chemical reactions using ultrasound in aqueous medium has been investigated by several researchers.[Citation21–23] Higher ultrasound frequencies are especially more favourable for the generation of hydroxyl radicals, possibly due to their faster production rates.[Citation24–26]
Due to the high surface area, good absorbing capacity and a nature of catalyst, the biologically synthesised iron oxide NP (INP) was used in the textile dye removal from the water system. Hence, in this present investigation, an attempt was made to decolourise the Direct Orange S azo dye using biologically synthesised nano iron catalyst.
2. Materials and methods
2.1. Chemicals
All the chemicals used in this study were AR grade and obtained from Himedia Laboratories Pvt. Ltd., India. The commercially available dye Direct Orange S was obtained from a dyeing unit, Uraiyur, Trichy (Dt), India. Required concentrations of H2O2 and dye solutions were prepared by dissolving them in distilled water. pH of the solutions was adjusted by adding 1N HCl or 1N NaOH. For high-performance liquid chromatography (HPLC) analysis, HPLC-grade methanol was used and phosphate buffer (pH 6.7) was prepared using Milli-Q water.
The INP was prepared using potato extract as starch template with ferrous sulphate heptahydrate (FeSO4·7H2O) as precursor. The synthesis and characterisation methods were previously published [Citation5] which represents the size (29–40 nm) and morphology (spherical) of the biosynthesised INPs.
2.2. Optimisation of INP, H2O2 and pH
Optimisation tests were carried out using varying concentrations of INP (0.2–1 g/l) and H2O2 (2–8 ml/l) to the fixed Direct Orange S dye concentration (10 mg/l). Control tests were also performed. The effect of pH was also studied at different values of 3, 5, 7, 9 and 11. With the optimised conditions of INP, H2O2 and pH, synchronised decolourisation of Direct Orange S was performed with magnetic stirrer (REMI Equipments, Mumbai, India) and ultra sonicator (Misonix, Medi Spec Pvt. Ltd., USA). The conditions for stirrer and sonication were 1000 X rpm and 30 KHz using ultrasonic power of 135 W and aqueous temperature of 26 ± 1°C, respectively. Ultraviolet–visible (UV–visible) spectrophotometer (BioTek, Synergy HT) readings of the samples were taken at 411 nm for every 15 min. The total decolourisation of dye was observed within 60 min by sonolysis and 120 min using magnetic stirrer.
2.3. Chromatography
The dye samples were decolourised with (INP + H2O2) and without H2O2 (INP alone) by sonolysis. HPLC measurements were performed on a photodiode array detector with Nucleosil C18 (250 × 4.6 mm) 100 Å 5 μm columns (Shimadzu, USA). A mobile phase consisting of 70:30 (methanol: 10 mM phosphate buffer), pH 6.7, was utilised at a flow rate of 0.5 mL/min for analytical separation, and detection was performed at 411 nm.
3. Results
3.1. Optimisation of INP concentration
In the present study, it was observed that as the particle loading concentration increases from 0.2 to 1.0 g/l, the decolourisation of the dye also increases. The maximum dye removal or decolourisation occurred at INP concentration of 1g/l because of the increased surface area of NPs, which provides more active site for binding. The complete colour removal was observed visually, while the UV spectral observation shows 87.2% ().
3.2. Optimisation of H2O2 concentration
To provide a strong oxidising condition sequential (2–8 ml/l) addition of H2O2 was carried out. In the present study, the optimised H2O2 value was observed at a concentration of 8 ml/l giving the highest percentage dye removal of 89% in UV spectral observation, whereas it showed complete (100%) colour removal visually ().
3.3. Optimisation of pH
The present investigation showed an increasing pattern of decolourisation between pH 3 and 7 and the optimised value was obtained at pH 9, showing dye removal percentage of 79%, whereas at higher pH 11, the decolourisation was less (). As the pH increased, the number of negatively charged surface sites on the iron oxide (INP) also increased. This favours the adsorption of dye cations due to electrostatic attraction. Hence, the decolourisation of the dye increases.
3.4. Decolourisation and kinetics study with or without H2O2
The very purpose of this study is to find out the effectiveness of the NPs in decolourisation which is given by calculating the rate of decolourisation. The decolourisation follows the first-order kinetics and best fits it [Citation27]
The kinetic study was observed for Orange S dye decolourisation with H2O2 (H2O2 + INP) in the dye solution and without H2O2 by sonolysis. The results inferred that the INP and H2O2 combination is more effective with rate constant ‘k’ (slope) values of k(obs) 30 min = 0.00397, k(obs) 60 min = 0.00424, whereas the rate of decolourisation without H2O2 in dye solution was much lesser at 60 min ().
3.5. Comparison between stirrer and sonicator
With the optimised conditions of INP (1 g/l), H2O2 (8 ml/l) and pH (9), the decolourisation of Direct Orange S dye was performed with both stirrer and sonicator. The complete dye decolourisation occurred in 120 min in the case of stirrer, whereas in the case of sonicator the complete decolourisation was observed in 60 min. The clear solution of decolourised sample was observed visually ((a)–3(c)).
Figure 3. (a) Control dye sample (b) and (c) decolourisation of Direct Orange S dye (10 mg/l) with INP (1 g/l) and H2O2 (8 ml/l) at pH 9 using stirrer and ultra sonicator, respectively.
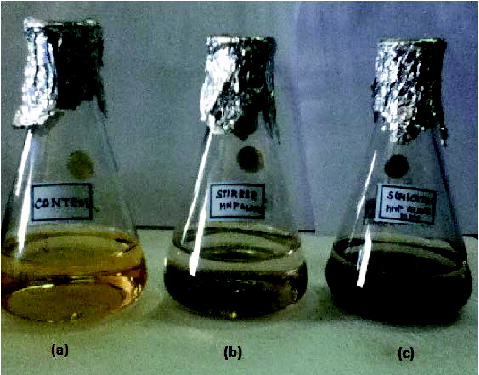
clearly shows that sonolysis was found to be more effective with rate constant ‘k’ (slope) values of k(obs) 30 min = 0.00397, k(obs) 60 min = 0.00424 than that of the stirrer [k(obs) 30 min = 0.002881, k(obs) 60 min = 0.00258 and k(obs) 120 min = 0.0026]. The extreme pressure and temperature conditions of sonolysis generated more number of (free radicals) •OH radicals which initiates the decolourisation reaction through oxidation. The scission of water caused by implosion of cavitations bubbles under this condition also results in pyrolysis of the organic compounds and volatile pollutants.
3.6. High-performance liquid chromatography analysis
With the objective of evaluating not only the colour removal by the photo-Fenton reaction but also the degree of mineralisation. The samples of dye decolourisation process performed with and without H2O2 by sonolysis were analysed using HPLC. The control sample without INP and H2O2 was also analysed. The peak obtained for Orange S dye was found to be 10.251 at Rt 12 min (). The absence of peak responsible for Orange S dye was noted in both INP + H2O2 () and INP alone, i.e. without H2O2 treated dye () solutions. The sample that does not have H2O2 for its advanced oxidation process showed less percentage of decolourisation.
3.7. Total dissolved solids
The total dissolved solids (TDS) of the Orange S dye water was analysed before and after treatment with the INP.[Citation28] The TDS value for Orange S dye was higher (2680 mg/L) than the standard limits (2100 mg/L) of ISI 2490–1981, whereas after treatment with INP, the TDS value was reduced to 1760 mg/L. Hence, it was confirmed that the INPs have the ability to decolourise the Direct Orange S dye at a significant level.
4. Discussion
The loading of the catalyst INP is an important factor that can greatly influence the Fenton reaction. Higher catalyst loading can lead to a faster rate of decolourisation of the organic compound, i.e. Direct Orange S textile dye. But on reaching a level of saturation, the decolourisation is completely slowed down or even stopped.[Citation29]
Decolourisation of organic compounds increases as the concentration of H2O2 increases, because H2O2 concentration is directly related to the number of OH radicals generated in the Fenton reaction. Initial H2O2 concentration plays an essential role in oxidation process. It should be added at an optimal concentration to achieve the best degradation. Addition of H2O2 exceeding the optimal concentration did not improve the respective maximum degradation.[Citation30]
Several studies have shown that decolourisation of organic compounds is greatly influenced by pH value.[Citation31–33] It can be explained by scavenging effect, where the decolourisation increases as pH of the sample is increased up to the optimal value and on further increase, i.e. at higher pH the formation of OH radicals becomes slow because of the hydrolysis of Fe2+ and the precipitation of FeOH from the solution. According to the literature, the optimal pH range for colour removal of dye was pH 3–11,[Citation34] the same was followed in this study and obtained the optimum value as 9 for Direct Orange S dye removal.
Many articles have proved that the •OH radical ions are the principal source for Fenton oxidation reactions; these ions get adsorbed on the active sites present on surface of the iron NPs.[Citation35,36] This work evidenced the decolourisation of an azo dye (Direct Orange S) using economically effective INP based on the Fenton oxidation within 60 min. Sonolysis enhances the decolourisation rate further by increasing the catalytic activity of the semiconductor catalyst. This could occur through decrease in size of the catalyst and disaggregation of particles due to the effect of sonication leading to an increase in surface area and thus the catalytic performance. The combination of both advanced oxidation (by H2O2) and sonolysis can increase the amount of reactive radical species inducing oxidation of the substrate and the decolourisation of intermediates and can be responsible for the highly observed decolourisation rate of the dye.[Citation37] From the above results, it can be inferred that sonolysis gives better decolourisation in lesser time (60 min) when compared to stirrer.
Further to confirm the absence of Direct Orange S dye in the treated water sample, HPLC plays as a key technique which was an evident to analyse the colour removal property of NPs during oxidation processes (). Using this sonolysis method, decolourisation of Direct Orange S dye achieved as high as 87% after 60 min with the optimised conditions of pH(9), INP (1g/l) and dye concentrations(10mg/l). This method will be an easy, economical and eco-friendly water remediation process. The methodology can be adapted for large-scale use in industries in association with textile effluent treatment plants. The treated water can be reused for the same purpose of dyeing or any other cleaning purposes. The magnetic NPs used in this textile dye removal from colourant water may be recollected and recycled from the treated water by applying a magnetic field and thus metal (iron) incorporation along with the treated water may be avoided.
Acknowledgements
The authors wish to record their thanks to UGC Research Fellowship in Sciences for Meritorious students (RFSMS), UGC-BSR Faculty Fellow (BFF), UGC, DST-FIST and MoE&F for financial support and Instrumentation facilities. S. Karthikeyeni is grateful to University Grants Commission for the award of UGC Research Fellowship in Sciences for Meritorious Students (RFSMS) for the financial support.
References
- Brumfiel G. Nanotechnology: a little knowledge. Nature. 2003;424:246–248.
- Hassan MHA. Small things and big changes in the developing world. Science. 2005;309:65–66.
- Siva Vijayakumar T, Karthikeyeni S, Vasanth S, Arul Ganesh, Bupesh G, Ramesh R, Manimegalai M, Subramanian P. Synthesis of Ag:ZnO nanocomposites by pulse mode ultrasonication and its properties. J Nanosci. Forthcoming 2013.
- Ramesh R, Arun S, Rajagopal G, Subramanian P. Synthesis of silicon dioxide (SiO2) nanoparticles by sol-gel method. In: Umadevi M, Parimaladevi R, Geetha N, Geetha TS, editors. Proceedings of the International Conference on Nanomaterials and Applications; 2012 Feb 28–29; New Delhi, India. New Delhi: Excel India Publishers; 2012.
- Karthikeyeni S, Siva Vijayakumar T, Vasanth S, Arul Ganesh, Manimegalai M, Subramanian P. Biosynthesis of iron oxide nanoparticles and its haematological effects on fresh water fish Oreochromis mossambicus. J Acad Indus Res. 2013;10:645–649.
- Vignesh V, Felix Anbarasi K, Karthikeyeni S, Sathiyanarayanan G, Subramanian P, Thirumurugan R. A superficial phyto-assisted synthesis of silver nanoparticles and their assessment on hematological and biochemical parameters in Labeo rohita (Hamilton, 1822). Colloids Surf A: Physicochem Eng Aspects. 2013. http://dx.doi.org/10.1016/j.colsurfa.2013.04.011
- Sathiyanarayanan G, Kiran GS, Selvin J. Synthesis of silver nanoparticles by polysaccharide bioflocculant produced from marine Bacillus subtilis MSBN17. Colloids Surf B 2013;102:13–20.
- Aitken RJ, Chaudhry MQ, Boxall ABA, Hull M. Manufacture and use of nanomaterials: current status in the UK and global trends. Occup Med. 2006;56:300–306.
- Zhang W. Nanoscalebiron particles for environmental remediation: an overview. J Nanopart Res. 2003;5:323–332.
- Chung KT, Cerniglia CE. Mutagenicity of azo dyes: structure activity relationship. Mutation Research 1992;277:201–220.
- Duran N, Esposito E. Potential applications of oxidative enzymes and phenoloxidase-like compounds in wastewater and soil treatment: a review. Appl Cat B: Environ. 2000;28:83–99.
- Mester T, Tien M. Oxidative mechanism of ligninolytic enzymes involved in the decolorization of environmental pollutants. Intern Biodeterioration and Biodecolorization 2000;46:51–59.
- Olukanni OD, Osuntoki AA, Gbenle GO. Textile effluent biodecolorization potentials of textile effluent-adapted and non-adapted bacteria. Africal J Biotech. 2006;5:1980–1984.
- Droste RL. Theroy and practice of water and wastewater treatment. Singapore: John Wiley & Sons, Inc; 2004.
- Sureshkumar R, Beris A. Effect of artificial stress diffusivity on the stability of numerical calculations and the flow dynamics of time-dependent viscoelastic flows. J Non-Newton Fluid Mech. 1995;60:53–80.
- Togo CA, Mutambanengwe CCZ, Whiteley CG. Decolourisation and decolorization of textile dyes using a sulphate reducing bacteria (SRB) – biodigester microflora co-culture. African J Biotech. 2008;7(2):114–121.
- Tzedakis T, Savall A, Clifton MJ. The electrochemical regeneration of Fenton's reagent in the hydroxylation of aromatic substrates: batch and continuous processes. J Appl Electrochem. 1989;19:911–921.
- Oturan MA, Pinson J. Polyhydroxylation of salicylic acid by electrochemically generated OH· radicals. N J Chem. 1992;16:705–710.
- Chou S, Huang YH, Lee SN, Huang GH, Huang C. Treatment of high strength hexamine-containing wastewater by electro-Fenton method. Water Res. 1999;33:751–759.
- Aaron JJ, Oturan MA. New photochemical and electrochemical methods for the decolorization of pesticides in aqueous media. Environmental applications. Tr J Chem. 2001;25:509–520.
- Hart EJ, Fischer CH, Henglein A. Sonolysis of hydrocarbons in aqueous solution. Radiat Phys Chem. 1990;36:511–516.
- Serpone N, Terzian R, Colarusso P, Minero C, Pelizzetti E, Hidaka H. Sonochemical oxidation of phenol and three of its intermediate products in aqueous media: catechol, hydroquinone and benzoquinone. Kinetic and mechanistic aspects. Res Chem Intermed. 1992;18:183–202.
- Serpone N, Colarusso P. Sonochemistry I. Effects of ultrasounds on heterogeneous chemical reactions – a useful tool to generate radicals and to examine reaction mechanisms. Res Chem Intermed. 1994;20:635.
- Colarusso P, Serpone N. Sonochemistry II. Effects of ultrasounds on homogenous chemical reactions and in environmental detoxifications. Res Chem Intermed. 1996;22:61–89.
- Hua I, Hoffmann M. Optimization of ultrasonic irradiation as an advanced oxidation technology. Environ Sci Technol. 1997;31:2237–2243.
- Entezari MH, Kruus P. Effect of frequency on sonochemical reactions II. Temperature and intensity effects. Ultrason Sonochem. 1996;3:19–24.
- Ameta G, Vaishnav P, Malkani RK, Ameta SC. Sonolytic, photocatalytic and sonophotocatalytic decolorization of toluidine blue. J Ind Council Chem. 2009;26:100–105.
- APHA. Standard methods for the examination of water and wastewater. 17th ed. Washington, DC: American Public Health Association; 1989.
- Feng J, Hu X, Yue PL. Novel bentonite clay-based Fe-nanocomposite as a heterogeneous catalyst for photo-Fenton discoloration and mineralization of Orange II. Environ Sci Technol. 2004;38:269–275.
- Movahedyan H, Seid Mohammadi AM, Assadi A. Comparison of different advanced oxidation processes degrading p-chlorophenol in aqueous solution. Iran J Environ Health Sci Eng. 2009;6–3:153–160.
- Kavitha SK, Palanisamy PN. Photocatalytic and Sonophotocatalytic decolorization of reactive red 120 using dye sensitized TiO2 under visible light. Int J Civil and Environ Eng. 2011;3:1.
- Sabhi S, Kiwi J. Decolorization of 2,4-Dichlorophenol by immobilized iron catalysts. Elsevier Science Wat Res. 2001;35:1994–2002.
- Rauf MA, Salman Ashraf S. Fundamental principles and application of heterogeneous photocatalytic decolorization of dyes in solution. Chem Eng J. 2009;151:10–18.
- Dindarloo Inaloo K, Naddafi K, Mesdaghinia AR, Nasseri S, Nabizadeh Nodehi R, Rahimi A. Optimization of operational parameters for decolorization and degradation of c. i. reactive blue 29 by ozone. Iran J Environ Health Sci Eng. 2011;8(3):227–234.
- Lim H, Lee J, Jin S, Kim J, Yoon J, Hyeon T. Highly active heterogeneous Fenton catalyst using iron oxide nanoparticles immobilized in alumina coated mesoporous silica. The Royal Society of Chemistry, Chem Commum. 2006;463–465. doi: 10.1039/b513517f
- Fernandez J, Dhananjeyan MR, Kiwi J, Senuma Y, Hilborn J. Evidence for Fenton photoassisted processes mediated by encapsulated Fe ions at biocompatible pH values. J Phys Chem B. 2000;104:5298–5301.
- Erena Z, Inceb NH. Sonolytic and sonocatalytic decolorization of azo dyes by low and high frequency ultrasound. J Hazard Mat. 2010;177:1019–1024.