Abstract
Catalytic activities of sonochemically prepared Au-core/Pd-shell-structured bimetallic nanoparticles (NPs) immobilised on TiO2 were evaluated. Comparing with the mixture of monometallic Au and Pd NPs on TiO2, core/shell-immobilised catalysts exhibited higher activities for the partial reduction of nitrobenzene (NB) to aniline (AN), suggesting that the synergistic effect originating from the core/shell structure enhanced the catalytic activities. In the case of high Au/Pd ratios, where the Pd-shell thickness was calculated to be 0.5 nm or lower, infrared spectroscopic measurements of adsorbed CO showed that the Au cores were successfully covered with Pd shells. It was found that a thin Pd shell of one layer or two layers of Pd atoms effectively catalysed the reduction of NB under ambient temperature, whereas the formation of AN was not confirmed on monometallic Au NP-immobilised catalysts.
1. Introduction
Bimetallic nanoparticles (NPs) have received considerable attention due to their unique properties which are different from pristine characteristics of the constituent monometallic NPs. Among bimetallic NPs, Au–Pd NPs have attracted much attention mainly in the field of catalysts.[Citation1–3] The synergistic effects of the addition of Au to Pd in enhancing the overall catalytic activity, selectivity and stability of the catalyst have been of great interest. Goodman et al. [Citation4] investigated the effect of the addition of Au using acetoxylation of ethylene and concluded that the critical reaction site for vinyl acetate synthesis consisted of two non-contiguous Pd monomers suitably spaced by Au. Guczi et al. [Citation5] reported the slight synergetic effect of Au/Pd alloy in the CO oxidation catalysed by Au/Pd bimetallic NPs immobilised on TiO2. The synergistic effects were not observed when SiO2 was used instead of TiO2. The direct synthesis of hydrogen peroxide [Citation6,7] and solvent-free oxidation of primary alcohols, which were catalysed by Au/Pd bimetallic NPs,[Citation8,9] were also reported.
The dependences of the catalytic activities on the Au/Pd ratios of the bimetallic NPs also have been extensively studied. Marx et al. [Citation10] investigated oxidation of benzyl alcohol and reported that admixing Pd to Au resulted in a continuous increase of selectivity to benzaldehyde reaching a maximum with catalysts containing Au/Pd ratio of 1/9. According to Sarkany et al., Au/Pd molar ratio of 20/80 in core/shell-structured NPs exhibited the highest catalytic activity for the hydrogenation of acetylene.[Citation3] Ishihara et al. [Citation11] also reported that bimetallic NPs of Au/Pd ratio of 19/81 immobilised on rutile TiO2 showed superior performance for hydrogen peroxide synthesis by hydrogen oxidation with O2. It is interesting that the optimum ratios are comparable irrespective of the reaction systems. Enhancements of the catalytic activities by the addition of Au have been discussed based on the stability, geometric structures of the catalysts, etc. Among them, the distribution of constituent Au and Pd atoms across a bimetallic NP is thought to be one of the important factors determining catalytic properties. Hosseini et al. [Citation12] reported that the higher activity of Pd(shell)–Au(core)/TiO2 was obtained for toluene and propene oxidation compared to those of Au(shell)–Pd(core) and Pd–Au(alloy).
The authors have been studying the preparation of the noble metal NPs by the effect of ultrasound and reported the sonochemical preparation of Au-core/Pd-shell bimetallic NPs. [Citation13,14] When an aqueous solution containing Au ions, Pd ions and surfactants such as sodium dodecylsulfate (SDS) was sonicated, noble metal ions were reduced in a stepwise fashion, first Au, and then Pd ions, to form the bimetallic NPs. The structure of the formed NPs which consisted of Au core and Pd shell was originated from this reduction sequence.
Recently, the authors reported that photocatalytic hydrogen evolution was enhanced by immobilising Au/Pd bimetallic NPs on TiO2 and that the photocatalytic activity including its wavelength dependence was tunable by changing the inner nanostructure of the bimetallic NPs.[Citation15] In the present study, the catalytic activities of Au/Pd bimetallic NP-immobilised TiO2 without light illumination were evaluated by employing partial reduction of nitrobenzene (NB) as a model reaction. By immobilising NPs with different Au-core/Pd-shell dimensions and evaluating their catalytic activities, the correlation between the inner structure and the synergistic effect coming from the structures was explored.
2. Experimental procedure
2.1. Materials
All reagents including noble metal complexes (Na2PdCl4, HAuCl4) and polyethyleneglycol monostearate (n ⋍ 40, PEG-MS) were purchased from Wako pure chemicals and used without further purifications. Aeroxide TiO2 P-25 powder was obtained from Nippon Aerosil.
2.2. Sonochemical preparation and immobilisation of the bimetallic NPs
The samples were fabricated in two steps, the sonochemical preparation of the core/shell NPs and the sonochemical immobilisation of the NPs on TiO2. Typically, 50 mL of an aqueous solution of the appropriate noble metal complexes and PEG-MS (0.4 mM) was prepared. Au/Pd ratios were 0/1.0, 0.25/0.75, 0.50/0.50, 0.75/0.25 and 1.0/0 (mM/mM). To investigate the effects of Pd-shell thickness on the catalytic activities, NPs were also prepared from 0.25/0, 0.25/0.40 and 0.25/3.0 (mM/mM). The aqueous solution was purged with Ar gas (>99.999% purity), which was suitable to obtain greater sonochemical effects,[Citation16] and then irradiated with ultrasound using a multiwave ultrasonic generator (KAIJO, TA-4021, 200 kHz, 6 W cm−2) connected to a lead zirconate titanate (PZT) oscillator. Sonication was carried out in a temperature-controlled water bath at 20°C. It was reported that the reducing agents required for the reduction of noble metal ions were generated in situ by the effects of the ultrasound.[Citation17,18] Namely, some organic compounds such as alcohols, surfactants or water-soluble polymers included in the sonicated system were decomposed by the cavitational collapse to form reactive radicals.[Citation16] Some of these radicals reduced noble metal ions in the solution to form NPs.
The progress of the sonochemical reduction was monitored by the improved colorimetric determination of the residual Au and Pd ions. Addition of saturated NaBr solution to the sample solution containing the ionic species of noble metals and reduced metallic particles makes the particles aggregated by the salting-out effect of sodium cations, and it becomes feasible to remove them by filtration. Noble metal ions in the filtrate become bromo complexes through the coordination of bromide ions to noble metal ions via a ligand exchange reaction, which have relatively large absorption coefficients in the near-ultraviolet region. As a result of this, remaining metal ions are colorimetrically determined without interference of scattering by the formed NPs. The details are described in our previous papers.[Citation13,Citation19] The sonication time was 30 min, which was enough to complete the reduction of all the noble metal ions. With the progress of the reduction, a yellowish colour of the solution originating from the noble metal complex changed to the characteristic colour of noble metal NPs depending on the Au/Pd ratio: when the ratio was high, the colour of the sonicated solution was reddish, and when low, the colour was dark brown.
Subsequently, TiO2 powder (0.64 g) was added into the solution and the sonication was prolonged for 30 min in air. The sonicated TiO2 powder was collected by filtration, rinsed and dried. The TiO2 powder took up the colour of the noble metal NPs and the filtrate was colourless transparent, which did not contain any noble metal species. This supports that all noble metal NPs are successfully immobilised on the TiO2. This sonochemical immobilisation of the prepared Au/Pd NPs is considered to come from the shock wave generated during the collapse of cavitation bubbles, which induces the inter-particle collisions with a high velocity.[Citation20] Details of the sonochemical immobilisation of noble metal NPs on TiO2 surface were previously reported elsewhere.[Citation21] Mixtures of monometallic Au and Pd NPs, which were separately prepared by the sonochemical method, were also successfully immobilised on TiO2 by the same protocol.[Citation15] It is impossible to prepare these mixture samples by ‘1 step method’, where the sample solution containing all materials is sonicated at once.
2.3. TEM observation
The morphologies and nanostructures of the catalysts were observed by a scanning transmission electron microscopy (STEM, FEI Titan 80-300 operated at 300 kV) equipped with a high-angle annular dark-field (HAADF) detector. A beam convergence angle of 10 mrad and a detector angle higher than 60 mrad were used for HAADF-STEM imaging. Sizes of more than 200 particles were measured on electron micrographs to obtain average sizes and its standard deviations.
2.4. IR spectra measurements of CO adsorbed on noble metal NPs
Infrared (IR) spectrometric experiments were performed in diffuse reflection geometry with an Fourier transform infrared (FTIR) spectrophotometer equipped with a liquid-nitrogen-cooled mercury cadmium telluride (MCT) detector (FT/IR-6100, Jasco) and a diffuse reflectance measurement unit (DR 600 Ci, Jasco). About 0.02 g of the specimen charged in the sample tray was placed in the sample chamber equipped with a KBr window and heated at 80°C under vacuum (lower than 10 Pa) for 1 h to remove the impurities on the sample surface. After the background spectra acquirement, the sample was cooled to 13 ± 3°C and CO of 20 ± 1 kPa was introduced 1 h before the spectra measurements. All spectra were recorded with a resolution of 4 cm−1 and 64 times of scanning after evacuation.
2.5. Evaluation of the catalytic activity
The catalytic activities were investigated by the partial reduction of NB under the reaction condition reported by Lu et al. [Citation22] The sonochemically prepared catalysts of 0.01 g were added to 30 mL of ethanol containing 0.3 mmol of NB. The reduction of NB was conducted at 25°C under 1 atm of hydrogen. The solution was periodically sampled and a trace of the catalysts in the sample solution was removed by centrifugation prior to the high performance liquid chromatography (HPLC) analyses. HPLC analyses were conducted by Shimadzu LC-2010AHT monitoring 254 nm using ODS-3 column (GL science, i.d. 4.6 mm × 150 mm length) and water/acetonitrile (50/50) as mobile phase with a constant flow rate of 1 mL min−1. The progress of the reaction was monitored by the amounts of formed aniline (AN) and consumed NB measured by HPLC. The catalytic activities are denoted as turnover frequency (TOF), obtained by the following equation:
(1)
As mentioned above, all noble metal ions are reduced and immobilised on TiO2. Therefore, it can be regarded that the amount of noble metals immobilised on TiO2 is equal to the amount of noble metal ions added in the catalyst preparation. In the evaluation of TiO2 alone, the formation of AN was not confirmed, indicating that the active site for NB reduction was the immobilised noble metal NPs.
3. Results and discussion
3.1. Characterisation of Au/Pd bimetallic NPs immobilised on TiO2
HAADF-STEM images of Au/Pd bimetallic NPs immobilised on TiO2 are shown in . The average sizes and the standard deviations of the immobilised NPs are also shown in the figure. Because the Z contrast is proportional to the square of the atomic number of the observed material in HAADF images,[Citation23] small particles with strong contrast are assignable to Au and/or Pd, and TiO2 particles appear with relatively weak contrast in spite of their large size. Au monometallic NPs ( (a)) are the largest with broad size distribution. By addition of Pd, the sizes became smaller and the distribution became narrower. This size reduction and narrowing of size distribution were formerly reported in the preparation of bimetallic NPs by alcoholic reduction method.[Citation24,25] In addition, it was also reported that the spontaneous formation of core/shell-structured bimetallic NPs by simply mixing two kinds of noble metal NP dispersions,[Citation26], and suggested that the thermal equilibrium might govern the uniformity observed during the alloying process. The present authors also reported the size reduction and the narrowing of size distribution in the sonochemically prepared Au-core/Pd-shell bimetallic NP dispersions in the presence of an SDS as stabiliser.[Citation13]
Figure 1. HAADF-STEM images of (a) Au 1.0/Pd 0, (b) Au 0.75/Pd 0.25, (c) Au 0.50/Pd 0.50, (d) Au 0.25/Pd 0.75 and (e) Au 0/Pd 1.0 bimetallic NP-immobilised TiO2.
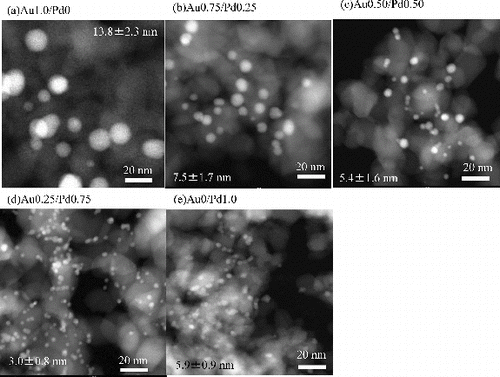
shows magnified HAADF-STEM images and energy dispersive X-ray spectroscopy (EDS) results. EDS analyses exhibited each bimetallic NP immobilised on TiO2 consisted of Au and Pd. However, the ratios of the signal intensities from each particle dispersed from stoichiometric values due to very low intensity of EDS signals. The insets in the STEM images in are the intensity profiles along the lines which intersect NPs. In the cases of Au 0.50/Pd 0.50 and Au 0.25/Pd 0.75, the crooked profiles of the image intensities demonstrate the core/shell structures of the immobilised NPs because the profiles show that the components of the particles discontinuously change at the points denoted by arrows in . However, in the case of Au 0.75/Pd 0.25, the crooked profile in HAADF signal was ambiguous in spite of its multiple twined structure, which is composed of several segments to form icosahedrons, decahedrons or a truncated structure.[Citation27] Au-core diameters and Pd-shell thickness calculated with the assumption that the bimetallic NPs consist of a spherical Au core and Pd shell with uniform thickness are also shown in the table, indicating that the Pd shell of the Au 0.75/Pd 0.25 NPs is very thin, 0.3 nm which corresponds to one layer or two layers of Pd atoms. This might suggest the incomplete Au-core coverage with Pd shell. However, Pd monometallic NPs directly immobilised on TiO2 or Pd islands on Au core were not observed by HAADF-STEM observation with EDS mappings.
Table 1. Structures of immobilised Au/Pd bimetallic NPs. Insets are the image intensity profiles along the indicated lines.
In order to investigate the Pd coverage over Au core, IR spectra of CO adsorbed on Pd were measured. FTIR spectra of CO adsorbed on the samples are displayed in (a). Characteristic absorption bands are observed except for Au/Pd = 1.0/0. Absorption bands at ∼1920, ∼1986 and ∼2088 cm−1 are assignable to CO on threefold hollow Pd (111) sites, CO on bridge sites and CO linearly bonded to Pd atoms, respectively.[Citation28,29] The intensity of the above band increases with the increment of Pd ratio and reaches the maximum at Au 0.25/Pd 0.75. In the case of Au 0.75/Pd 0.25, where the calculated Pd-shell thickness was only 0.3 nm, adsorption bands coming from CO are confirmed. The relationship between the peak areas of adsorbed CO in the IR spectra and the total surface areas of immobilised NPs is shown in (b). The total surface area was calculated using the following equation:
(2)
Figure 2. (a) IR spectra of CO adsorbed on Au/Pd bimetallic NP-immobilised TiO2 and (b) the relationship between the surface areas of the immobilised NPs and the peak areas of adsorbed CO.
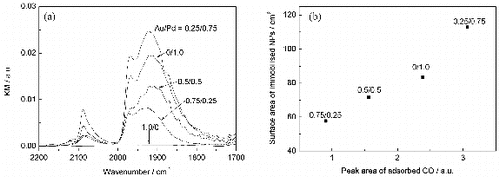
In the present case, the amount of noble metals immobilised on TiO2 is equal to the amount of noble metal ions added in the catalyst preparation. The mass of an immobilised NP was calculated using the average diameters observed by TEM. The calculations were conducted with the assumptions that the NPs were spherical and their densities were the same as the bulk metal and that the bimetallic NPs consisted of spherical Au core and Pd shell with uniform thickness. The surface area of an immobilised NP was derived from the average size observed by TEM. The linear correlation in (b) supports that the surfaces of the bimetallic NPs are covered with Pd even in the case of Au/Pd = 0.75/0.25. The results of TEM observations and IR analyses show that the surfaces of Au/Pd bimetallic NPs are covered with Pd shells.
3.2. Evaluation of catalytic activities
The catalytic activities (TOF) are shown in . The activity of Au 1.0/Pd 0 is the lowest, almost zero. With the increase of Pd ratio, the activities are enhanced and reach the maximum value when Au/Pd is 0.25/0.75. On the other hand, in the cases of the mixture of Au and Pd monometallic NPs immobilised on TiO2, the activities are monotonously increased with the Pd mol ratios. The Au/Pd ratio dependences are similar to those of the previously reported photocatalytic activities of Au/Pd bimetallic NPs immobilised on TiO2.[Citation15] When the Au/Pd ratio is the same, the activities of core/shell catalysts are superior to those of the mixture of monometallic NPs immobilised on TiO2. This synergistic effect is thought to be originated from the core/shell structure. The synergistic factors, which are defined as the ratios of TOF of core/shell to that of the mixture with the same Au/Pd ratio, are also shown in . The synergistic effect of Au 0.75/Pd 0.25 NPs is the highest 5.9, which is superior to the previously reported value.[Citation30] It is found that the Pd thin layer, which is calculated to be 0.3 nm thickness (see ) corresponding to only one layer or two layers of Pd atoms, can catalyse the reaction, even though Au monometallic core substantially has no activity. Additionally, by comparing with the activities of the mixture of monometallic Au and Pd NPs on TiO2, the synergistic effect is found to originate essentially from the core/shell structure, not from simple proximity effect of Au and Pd.
Figure 3. TOF (per Au + Pd) of (a) Au-core/Pd-shell bimetallic NPs and (b) mixture of monometallic Au and Pd NP-immobilised TiO2 (left axis) and (c) synergistic factor (histogram, right axis).
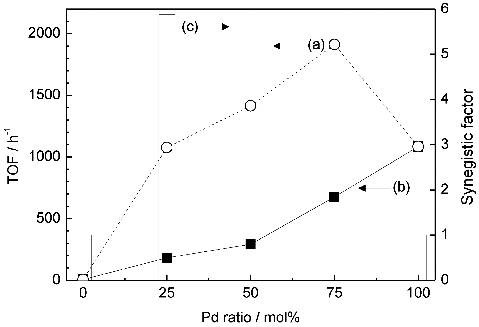
The contribution of this Pd thin shell is found to be pronounced by normalisation of the reaction rates by the total surface area calculated using Equation (2). The normalised catalytic activities are shown in . In the case of the mixtures of Au and Pd NP-immobilised TiO2, the rates are normalised by the surface area of both noble metal NPs. The normalised activities of core/shell NPs exceed those of Pd monometallic NPs and the mixture of monometallic NPs. These data support that the enhancement of the catalytic activities of core/shell particles is inherently attributable to core/shell structure, not to the large surface area of the bimetallic NPs.
Figure 4. Catalytic activities normalised by the surface area of immobilised (a) Au-core/Pd-shell bimetallic NPs and (b) mixture of monometallic Au and Pd NPs.
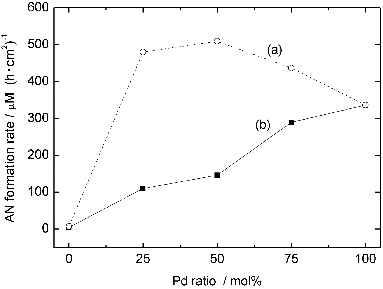
In order to evaluate the electron structure of the present samples, X-ray photoelectron spectroscopy (XPS) measurements were carried out with an electron spectrometer (Kratos AXIS-Ultra DLD, Shimadzu) equipped with monochromated Al Kα radiation at a base pressure of 3.0 × 10−7 Pa. shows XPS spectra of (a) Pd 3d and (b) Au 4f of the core/shell NP-immobilised TiO2. Peak positions of metallic states are shown in the figures. The Pd 3d 5/2 peak overlaps with the peak of Au 4d 5/2 at around 335 eV so that it is difficult to interpret the individual contributions to this peak. With the increase of Au/Pd ratio, the Pd 3d 3/2 peak shape becomes sharp and shifts to the direction of lower binding energy. On the other hand, the peaks of Au 4f shift to the direction of lower binding energy and their positions are almost same regardless of the Au/Pd ratio. The reduction state of Pd shell seems to be preferable for the reduction of NB. However, the detailed electron structure is not clear at the present time and will be reported in the future work.
Figure 5. (a) Pd 3d and (b) Au 4f XPS spectra of Au-core/Pd-shell bimetallic NPs immobilised on TiO2.
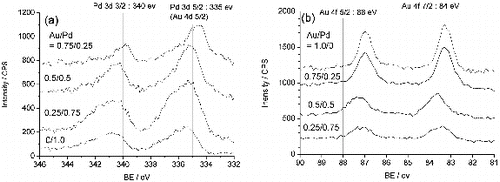
In order to investigate the dependence of the catalytic activities on the Pd-shell thickness, bimetallic NPs prepared from constant 0.25 mM of Au ions and various concentrations of Pd ions were immobilised on TiO2. The average diameter, Au-core diameter and Pd-shell thickness are summarised in . When Pd ion is 0.4 mM, the diameters of the immobilised core/shell NPs are smaller than those of monometallic Au particles obtained from 0.25 mM of Au ions. When Pd ion is 3.0 mM, the thickness of the Pd shell is 1.3 nm, but the assembly of some NPs on TiO2 is observed.
Table 2. HAADF-STEM images and the sizes of TiO2 immobilising Au/Pd bimetallic NPs prepared from 0.25 mM HAuCl4 and various concentrations of Na2PdCl4.
shows the dependence of catalytic activity on the Pd-shell thickness. The TOF reaches the maximum when the Pd shell is about 0.5 nm and descends with the increment of Pd-shell thickness. When AN formation rates are normalised by the surface area, the rate reaches the plateau when Pd shell is about 0.5 nm.
Figure 6. Dependences of catalytic activities on the Pd-shell thickness. (▪; left axis): normalised AN formation rate, (○; right axis): TOF.
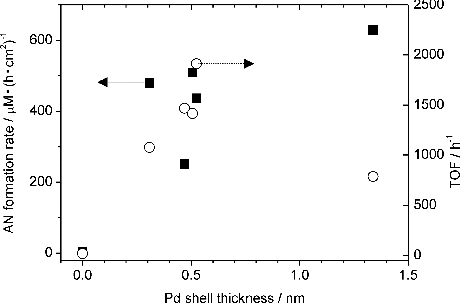
Two possibilities can be proposed to explain the catalytic activity declines when Pd shell becomes thick. First, we believe that the electronic interaction between Au and Pd enhances the catalytic activities of the Au-core/Pd-shell NPs.[Citation14] When the shell becomes thicker, the interaction is thought to be weaker.
Another factor might be alloying of the Pd thin layer with Au. Ding et al. investigated the atomic structures of Au/Pd bimetallic NPs in detail.[Citation31] They reported that when Au core was epitaxially covered with Pd shell, the strain between Au core and Pd shell was released in two different manners depending on the Pd-shell thickness. When the Pd shell was a monoatomic layer of Pd, the strain was released with Shockley partial dislocation accompanied with the formation of stacking faults. On the other hand, when the Pd shell became thicker (>2 nm), the diffusion of Au atoms into the Pd shell released the strain. Consequently, the shell consisted of Au–Pd alloy, not pure Pd. In the present study, the thickness of Pd shell is between monoatomic layer and 2 nm. Therefore, some of the Pd shells might be alloyed with Au coming from the core. This alloying is thought to contribute to the present descending of the activities above 0.5 nm of Pd-shell thickness.
On the other hand, we previously reported the structural change of Au-core/Pd-shell NPs immobilised on TiO2 by annealing. When the NPs were annealed at 400°C under hydrogen atmosphere, the multiple twin structure originated from the Au-core/Pd-shell structure was destroyed and changed into face-centred cubic structure, where Au and Pd atoms were homogeneously located across the particles.[Citation15,Citation32] In addition, significant size growth of the immobilised NPs by annealing was not observed. When evaluating the catalytic activity of the annealed catalyst for the partial reduction of NB, the TOF of the Au 0.25/Pd 0.75 on TiO2 drastically descended from 1911 to 593 h−1.
This suggests that the alloying of Pd shell with Au atoms diffusing from the Au core is attributable to the descent of the catalytic activities when the Pd-shell thickness grows. However, it is not easy to evaluate the degree of Pd-shell alloying. We are planning to conduct the structural analyses by extended X-ray absorption fine structure (EXAFS).
4. Conclusions
By sonochemical method, Au-core/Pd-shell NPs with various core/shell sizes were successfully immobilised on TiO2. The sizes of the Au/Pd NPs and the standard deviation became smaller with the increase of Pd ratio. The core/shell structures of the NPs were confirmed using HAADF-STEM observations and CO adsorption technique. The dependence of the inner structure, especially the Pd-shell thickness, on the catalytic activities for the partial reduction of NB was investigated. Even when the Pd shell was very thin, for example, 0.3 nm, the catalytic activities were dramatically enhanced comparing with monometallic Au and Pd NPs. The enhancement coming from the core/shell structure declined with the growth of Pd-shell thickness above 0.5 nm. This is probably due to low electronic interaction between Au and Pd and alloying effects of Pd shell with Au. Theoretical calculations of the electron structure dependence on alloying and Pd-shell thickness are under way.
Acknowledgements
The authors wish to thank Ms Y. Matsuda from IMR, Tohoku University for her helpful assistances in preparation of catalysts and evaluation of the catalytic activities and Ms K. Ohmura from IMR, Tohoku University for XPS analyses. The authors acknowledge the Grant-in-Aid for Young Scientists (A) (No. 22686074) from Japan Society for the Promotion of Science (JSPS) and the Grant-in-Aid for Scientific Research (A) (No. 22241023) from the Ministry of Education, Culture, Sports, Science and Technology (MEXT).
References
- Allison EG, Bond GC. The structure and catalytic properties of palladium-silver and palladium-gold alloys. Catal Rev. 1972;7:233–289.
- Villa A, Campione C, Prati L. Bimetallic gold/palladium catalysts for the selective liquid phase oxidation of glycerol. Catal Lett. 2007;115:133–136.
- Sarkany A, Hargittai P, Horvath A. Controlled synthesis of PDDA stabilized Au–Pd bimetallic nanostructures and their activity in hydrogenation of acetylene. Top Catal. 2007;46:121–128.
- Chen M, Kumar D, Yi C-W, Goodman DW. The promotional effect of gold in catalysis by palladium-gold. Science. 2005;310:291–293.
- Guczi L, Beck A, Horváth A, Koppány Z, Stefler G, Frey K, Sajó I, Geszti O, Bazin D, Lynch J. AuPd bimetallic nanoparticles on TiO2: XRD, TEM, in situ EXAFS studies and catalytic activity in CO oxidation. J Mol Catal A. 2003;204–205:545–552.
- Edwards JK, Solsona BE, Landon P, Carley AF, Herzing A, Kiely CJ, Hutchings GJ. Direct synthesis of hydrogen peroxide from H2 and O2 using TiO2-supported Au–Pd catalysts. J Catal. 2005;236:69–79.
- Landon P, Collier PJ, Carley AF, Chadwick D, Papworth AJ, Burrows A, Kiely CJ, Hutchings GJ. Direct synthesis of hydrogen peroxide from H2 and O2 using Pd and Au catalysts. Phys Chem Chem Phys. 2003;5:1917–1923.
- Li G, Enache DI, Edwards J, Carley AF, Knight DW, Hutchings GJ. Solvent-free oxidation of benzyl alcohol with oxygen using zeolite-supported Au and Au–Pd catalysts. Catal Lett. 2006;110:7–13.
- Enache DI, Edwards JK, Landon P, Solsona-Espriu B, Carley AF, Herzing AA, Watanabe M, Kiely CJ, Knight DW, Hutchings GJ. Solvent-free oxidation of primary alcohols to aldehydes using Au-Pd/TiO2 catalysts. Science. 2006;311:362–365.
- Marx S, Baiker A. Beneficial interaction of gold and palladium in bimetallic catalysts for the selective oxidation of benzyl alcohol. J Phys Chem C. 2009;113:6191–6201
- Ishihara T, Hata Y, Nomura Y, Kaneko K, Matsumoto H. Pd–Au bimetal supported on rutile–TiO2 for selective synthesis of hydrogen peroxide by oxidation of H2 with O2 under atmospheric pressure. Chem Lett. 2007;36:878–879.
- Hosseini M, Barakat T, Cousin R, Aboukaïs A, Su B-L, De Weireld G, Siffert S. Catalytic performance of core–shell and alloy Pd–Au nanoparticles for total oxidation of VOC: the effect of metal deposition. Appl Catal B: Environ. 2012;111–112:218–224.
- Mizukoshi Y, Okitsu K, Maeda Y, Yamamoto TA, Oshima R, Nagata Y. Sonochemical preparation of bimetallic nanoparticles of gold/palladium in aqueous solution. J Phys Chem B. 1997;101:7033–7037.
- Mizukoshi Y, Fujimoto T, Nagata Y, Oshima R, Maeda Y. Characterization and catalytic activity of core-shell structured gold/palladium bimetallic nanoparticles synthesized by the sonochemical method. J Phys Chem B. 2000;104:6028–6032.
- Mizukoshi Y, Sato K, Konno TJ, Masahashi N. Dependence of photocatalytic activities upon the structures of Au/Pd bimetallic nanoparticles immobilized on TiO2 surface. Appl Catal B: Environ. 2010;94:248–253.
- Riesz P. Free radical generation by ultrasound in aqueous solutions of volatile and non-volatile solutes. In: Advances in sonochemistry. Vol. 2, Mason TJ, editor. London: JAI Press; 1991. p. 23–64.
- Mizukoshi Y, Oshima R, Maeda Y, Nagata Y. Preparation of platinum nanoparticles by sonochemical reduction of the Pt(II) ion. Langmuir. 1999;15:2733–2737.
- Mizukoshi Y, Takagi E, Okuno H, Oshima R, Maeda Y, Nagata Y. Preparation of platinum nanoparticles by sonochemical reduction of the Pt(IV) ions: role of surfactants. Ultrason Sonochem. 2001;8:1–9.
- Mizukoshi Y, Okitsu K, Bandow H, Nagata Y, Maeda Y. Improved colorimetric determination of noble metal ions in multitudinous solution using sodium bromide or sodium iodide. Bunseki Kagaku. 1996;45:327–331.
- Doktycz SJ, Suslick K. Interparticle collisions driven by ultrasound. Science. 1990;247:1067–1069.
- Mizukoshi Y, Makise Y, Shuto T, Hu J, Tominaga A, Shironita S, Tanabe S. Immobilization of noble metal nanoparticles on the surface of TiO2 by the sonochemical method: photocatalytic production of hydrogen from an aqueous solution of ethanol. Ultrason Sonochem. 2007;14:387–392.
- Lu P, Teranishi T, Asakura K, Miyake M, Toshima N. Polymer-protected Ni/Pd bimetallic nano-clusters: preparation, characterization and catalysis for hydrogenation of nitrobenzene. J Phys Chem B. 1999;103:9673–9682.
- Pennycook SJ. Z-Contrast stem for materials science. Ultramicroscopy. 1989;30:58–69.
- Toshima N, Harada M, Yamazaki Y, Asakura K. Catalytic activity and structural analysis of polymer-protected Au-Pd bimetallic clusters prepared by the simultaneous reduction of HAuCl4 and PdCl2. J Phys Chem. 1992;96:9927–9933.
- Toshima N. Polymer-protected bimetallic clusters. Preparation and application to catalysis. J Macromol Sci Chem. 1990;A27:1225–1238.
- Toshima N, Kanemura M, Shiraishi Y, Koga Y. Spontaneous formation of core/shell bimetallic nanoparticles: a calorimetric study. J Phys Chem B. 2005;109:16326–16331.
- Dai Z-R, Sun S, Wang ZL. Shapes, multiple twins and surface structures of monodisperse FePt magnetic nanocrystals. Surf Sci. 2002;505:325–335.
- Borchert H, Jürgens B, Zielasek V, Rupprechter G, Giorgio S, Henry CR, Bäumer M. Pd nanoparticles with highly defined structure on MgO as model catalysts: an FTIR study of the interaction with CO, O2, and H2 under ambient conditions. J Catal. 2007;247:145–154.
- Bertarione S, Scarano D, Zecchine A, Johánek V, Hoffmann J, Schauermann S, Frank MM, Libuda J, Rupprechter G, Freund H-J. Surface reactivity of Pd nanoparticles supported on polycrystalline substrates as compared to thin film model catalysts: infrared study of CO adsorption. J Phys Chem B. 2004;108:3603–3613.
- Shao M, Wang H, Zhang M, Ma DDD, Lee S-T. The mutual promotional effect of Au–Pd bimetallic nanoparticles on silicon nanowires: a study of preparation and catalytic activity. Appl Phys Lett. 2008;93:243110.
- Ding Y, Fan F, Tian Z, Wang ZL. Atomic structure of Au-Pd bimetallic alloyed nanoparticles. J Am Chem Soc. 2010;132:12480–12486.
- Nakagawa T, Nitani H, Tanabe S, Okitsu K, Seino S, Mizukoshi Y, Yamamoto TA. Structural analysis of sonochemically prepared Au/Pd nanoparticles dispersed in porous silica matrix. Ultrason Sonochem. 2005;12:249–254.