Abstract
In this paper, we studied the synergistic effect of sodium citrate dispersant and polyvinylpyrrolidone or polyvinylalcol capping polymer on controlling the size of copper nanoparticles (CuNPs) that were prepared by the chemical reduction method. The CuNPs were characterised by using ultraviolet–visible (UV–Vis) spectroscopy, transmission electron microscopy, and X-ray diffraction techniques. The size of the nanoparticles could be predicted via their UV–Vis absorbance. In the presence of capping polymers, the size of CuNPs was significantly changed, ranging from 2 ± 1 to 20 ± 7 nm with and without sodium citrate dispersant, respectively. Moreover, surface plasmon resonance of ultrafine CuNPs (2 nm in diameter) could be observed in the short wavelength region by using UV–Vis spectra. Our finding, could offer a new synthetic approach to the production of highly stable, small-sized CuNPs.
1. Introduction
Synthesis and characterization of metallic nanoparticles have recently been paid more attention in view of several applications.[Citation1,2] A large number of studies indicated that these nanosized metallic nanoparticles had many novel and different properties such as optical, catalytic, biomedical properties, etc., compared to their bulk metallic form.[Citation3–5] Among them, copper nanoparticles (CuNPs) exhibited interesting thermal and electrical conductivity, antifriction, and antibacterial properties. Therefore, they have more potential in the practical applications such as heat exchangers, conductive copper lines in inkjet printing, antifriction additive in lubricant, and microbial agents.[Citation6–13]
Up to now, many methods have been reported to prepare the CuNPs such as chemical method, polyol method, thermal decomposition, microwave heating, microemulsion techniques, etc.[Citation14–20] It is well known that at small sizes, CuNPs and other metallic nanoparticles exhibit special chemical, physical, and biological properties due to the increase in the surface area of the nanoparticles. Preparation of CuNPs by chemical reduction or polyol method seems to be easier but it requires a long time or high temperatures to complete the reaction.[Citation18,Citation21] Furthermore, finding a simple method to prepare small-sized, narrow-distribution, and high-stability CuNPs is a difficult task. Until now, only a small number of studies have been available.[Citation22,23] However, in those reports, copper salts were chemically reduced in organic solvents under strict conditions and using some particular agents that may be hard to exploit the obtained product for the practical applications. Therefore, studies on the preparation of CuNPs with small sizes based on simple, friendly methods and low cost are even more attractive.
In this study, we suggested a new method to prepare small-sized CuNPs by chemical reduction using basic chemical agents in which the synergistic effect of citrate dispersant and capping polymers has been expected to control the size growth of CuNPs. Ultraviolet–visible (UV–Vis) spectroscopy was used to predict nanosized formation of copper. To optimise the conditions for preparing small-sized CuNPs, several parameters were investigated such as reducing temperature, presence of the sodium citrate dispersant, and polyvinylpyrrolidone (PVP) or polyvinylalcol (PVA) capping polymer cofactor.
2. Materials and methods
2.1. Materials
Copper(II) chloride dihydrate (CuCl2·2H2O, 99.0%), glycerol (C3H5(OH)3, 99.0%), polyvinylpyrrolidone (Mw 58,000 g/mol), trisodium citrate dihydrate (99.0%), hydrazin dihydrate (N2H4·2H2O, 50%) were purchased from Acros Organics (Fair Lawn, NJ). Polyvinyl alcohol (Mw = 60,000 g/mol) was obtained from Merck. All other reagents were used without further purification.
2.2. Synthesis of CuNPs with dispersant and capping polymer cofactors
In the synthetic process, we conducted the reaction using a simple method in a short period of time that could scale up for practical production. Briefly, capping polymer (PVP) 0.2 g, CuCl2 (0.1M), and trisodium citrate (0.1M) were dissolved in 30 ml glycerol. The mixture was heated to a pre-determinated temperature by using a microwave oven (Sanyo EM-S2088W, 800 W). Once the set temperature (around 50–80 seconds) was reached, the oven was turned off and hydrazine hydrate solution (equal to molar number of copper salt for all reaction) was immediately added dropwise to the mixture under stirring. The reaction mixture was stopped to obtain a colloidal CuNP in 1 minute after the addition had completed. The colloidal CuNPs were characterised by UV–Vis, transmission electron microscopy (TEM), and X-ray diffraction (XRD). The effects of the reduced temperature (110–150 °C) in the presence of sodium citrate and PVP, with concentration of sodium citrate (sodium citrate/CuCl2 (mol/mol) = 0.0, 0.1, 0.25, 0.5, 0.75, 1.0, and 1.25) and copper salt (CuCl2/PVP = 1–7 wt%), on absorbance and CuNP size distribution were investigated. An additional experiment was done with PVA instead of PVP under the optimal condition.
2.3. Characterisation
UV–Vis absorption spectrum of the colloidal CuNPs solution was measured by Jasco V670 (Jasco Analytical Instrument), TEM images were measured by JEM-1400 version (JEM-1400, JEOL). The samples for TEM measurement were prepared by dropping CuNPs solution onto a carbon-coated copper grid. The histogram of the particle-size distribution and the average diameter were obtained by measuring particles. The XRD result was characterised using D8 advanced Bragg X-ray (D8 Advance, Brucker) with Cu Kα radiation. For sample handling, glass slide was used as a substrate for measurement. Leaned substrate was covered with the colloidal CuNPs solution and dried in air.
3. Results and discussion
Regarding to several reports in the previous section, there are many methods and techniques to prepare CuNPs. Every method includes parameters that can affect on the size of the nanoparticles. Parameters such as temperature, amount of metallic salt, dispersant, and capping polymers should be optimised in the reduction reaction of copper salt in glycerol solvent in order to obtain small-sized CuNPs.
3.1. Influence of temperature on CuNP size distribution in the presence of citrate
During the nanoparticle preparation, the reaction temperature had a strong influence on size distribution. However, the correlation between reaction temperature and nanoparticle morphologies has received less attention when evaluating each typical condition systematically. Our results indicated that CuNPs that were prepared at different temperature points exhibited different UV–Vis absorbance and nanosize distribution, as shown in and . shows that the UV-Vis absorbance band of the colloidal solution shifted to longer wavelengths when it was prepared at the higher reaction temperature. According to the spectra, it could be predicted that the increase in reaction temperature might contribute to produce larger sized CuNPs. The prediction was confirmed in TEM images. (a) and (b) show morphologies of reduced CuNPs at reaction temperature of 110 and 130 °C have diameter in range of 17 ± 4 and 33 ± 5 nm, respectively. These results indicated that the produced CuNPs at lower reaction temperature were smaller than those at higher reaction temperature. These could be explained that nuclei surface activity of the small-sized CuNPs increases when temperature is increased which makes the produced CuNPs easy to collide and reunite, resulting in increase of particle size.[Citation24] Xiong et al. indicated clearly that increment in the reaction temperature helps increase reaction rate resulting in enhancing particle size.[Citation25]
Figure 1. Correlation between UV–Vis absorbance wavelength and reaction temperature in preparation of CuNPs.
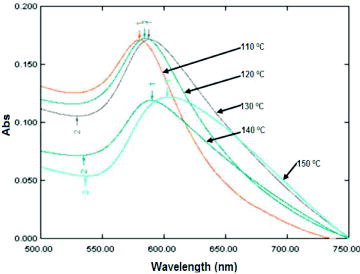
Purity and stability of the CuNPs produced at the reaction temperature (110 °C) were tested by XRD. In , XRD diffractogram of CuNPs does not show any peak of copper oxides and only peaks of metallic copper with high crystallinity at 43.2o, 50.4o, and 74.0o appear. These peaks correspond to the typical face-centred cubic of copper with miller indices at (111), (200), and (220), respectively.[Citation26]
3.2. Effect of ratio of citrate and copper salt on the size of nanoparticles
Preparation of CuNPs in the absence or presence of citrate dispersant exhibited quite different behaviours. As shown in (a), their colour shifted from brown to bordeaux. (b) shows UV–Vis absorbance of CuNP colloidal solution prepared without citrate dispersant, in which the absorbance band shifted to longer wavelength. When molar ratio of the dispersant to copper salt increased, it caused CuNP absorbance band to shift to shorter wavelength. This could indicate a change in nanoparticle size. However, absorbed wavelengths are insignificantly different when using molar ratios ranging from 0.5 to 1.25.
Figure 4. Colour of the CuNP colloid solutions without and with citrate dispersant (a) and their UV–Vis absorbance (b).
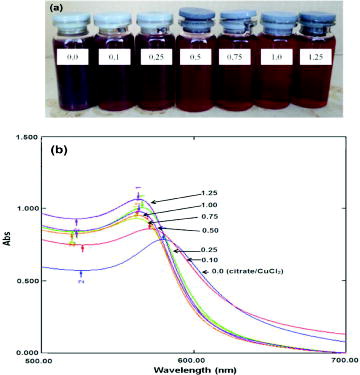
Their TEM images shown in (a) and (b) could contribute to confirm correlation between citrate concentration and the size of the produced CuNPs. In the experiment without citrate, average diameter of CuNPs ranges from 10 to 35 nm (20 ± 7 nm), whereas its diameter appears in a range of 3 ± 1 nm at molar ratio of citrate/CuCl2 = 0.5. Therefore, the ratio of citrate to copper salt is optimised at 0.5 to prepare CuNPs. Moreover, these results confirm that CuNPs with smaller size absorb shorter wavelength in UV–Vis spectrum. Addition of citrate plays a synergistic role to stabilise effectively the nanoparticles in the presence of capping polymer as illustrated in .
Figure 5. TEM images of CuNPs prepared without (a) and with citrate dispersant (b) (molar ratio of citrate/CuCl2 = 0.5).
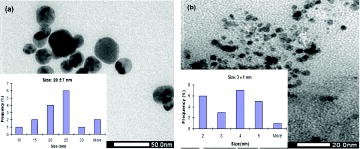
Figure 6. Synergistic effect of citrate dispersant and PVP capping polymer on controlling size growth of CuNPs.
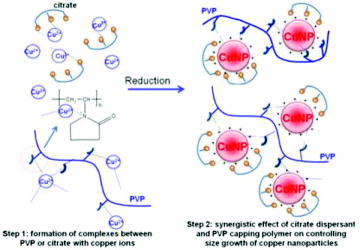
PVP is the most popular capping polymer used to protect colloidal solution containing metallic nanoparticles due to its capping effect. However, the bulky polymer is so hard to coat all surfaces of the metallic nanoparticles in order to protect them from their collision that results in outgrowth of their size. In preparation of some metallic nanoparticles including copper, trisodium citrate was also used as a dispersant and small molecular protecting agent.[Citation27,28] The molecule could be well adsorbed on the surface of metallic nanoparticles. Therefore, once the citrate/Cu− salt ratio increases, there are higher amount of citrate adsorbed on the surface of the produced CuNPs that reduces the nuclei surface activity of the small-sized CuNPs, resulting in decrease of CuNP size. However, it has been hard to prepare these small-sized metallic nanoparticles in the sole presence of capping polymers or citrate dispersant. Therefore, the synergistic effect of citrate dispersant and capping polymers has been expected to control size growth of CuNPs as demonstrated in . Alkaline metal salts of tartaric and gluconic acids could play a synergistic role like citrate to stabilise the nanoparticles because these molecules were used to stabilise several kinds of metallic nanoparticles. The highly, negatively charged tartrate and gluconate ions could cover on the surface of the CuNPs via electrostatic interaction.[Citation29,30]
3.3. Effect of the amount of feeded CuCl2 salt on the size of CuNPs (citrate/CuCl2 = 0.5)
In this experiment, increment in the copper salt concentration was combined to the increment of hydrazine concentration and kept constant in molar ratio of citrate to salt. The colloidal solutions at 1–3 wt% of the salt with PVP gave orange-like colour (as shown in (a)). Their UV–Vis absorbance peaks (in (b)) seemed to be reduced and partially replaced by surface plasmon effect of separated CuNPs with small size (less than 4 nm). The surface plasmon resonance of the CuNPs (with diameter less than 4 nm) was displayed as a broadened peak at short wavelength.[Citation24] These signals indicated that the colloidal solutions were prepared from 1 to 3 wt% of the salt with PVP containing CuNPs with very small size. The prediction was confirmed in (c), in which diameter of the CuNPs prepared from 5 wt% of the salt with PVP is around 4 nm.
3.4. Effect of PVA capping polymer on CuNP size (mole of citrate/CuCl2 = 0.5; CuCl2/PVA = 0.05 wt%)
The preparation of CuNPs (with PVP and citrate) under optimal conditions can result in CuNPs with small size (diameter is less than 4 nm). One additional experiment was conducted with PVA capping polymer instead of PVP at the same condition. In (a), besides UV–Vis, absorbance peak of CuNPs appear at wavelength 558 nm, a broadened region of the surface plasmon also appears from short wavelength region to 558 nm.[Citation22,23] This phenomenon indicates that CuNPs were prepared with diameter less than 4 nm in the presence of PVA. This result is clearer via TEM observation ((b)), in which the average diameter of CuNPs is around 2 nm and histogram shows a narrow-size distribution. The average diameter of CuNPs from TEM observation accorded with the results from UV–Vis measurement of the colloidal solutions. These results are also similar to those reported by Zhang et al., namely that the average diameter of CuNPs was prepared around 2 nm.[Citation22] In comparison with the experiment using PVP, CuNPs were obtained with smaller size in the presence of PVA at the same condition. The result could be explained by PVA and PVP possessing hydroxyl groups or amide groups that can form complexes with transition metals. However, PVA has higher concentration of hydroxyl groups present as compared to amide groups in PVP.[Citation31] These results show that aqueous solutions of PVA are more viscous than those of PVP at the same polymer concentration. This resulted in reducing CuNP size growth or aggregation in PVA.[Citation31]
Figure 8. Characteristics of the CuNPs with PVA capping polymer: UV–Vis absorbance of the CuNPs with a broadened region of the surface plasmon (a) and TEM image including size distribution (b).
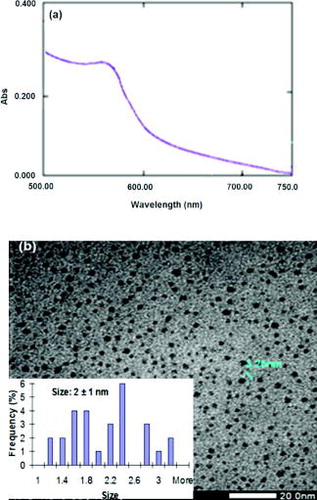
Besides several investigations presented in this paper, stability of the CuNP colloidal solutions was also assessed. The colloidal solution had not changed colour after 1 month without storing in any inner gas environment (data not shown here). This could be significant for several practical applications.
4. Conclusion
In summary, small-sized CuNPs were prepared (around 2 nm with PVA and 4–5 nm with PVP polymer in the presence of citrate) using chemical reduction and basic chemical agents and large-scale production. At this condition, the CuNPs exhibited narrow-size distribution and high stability. All investigations indicated that the nanosize of CuNPs in their colloidal solution could be predicted by using their UV–Vis absorbance and confirmed by TEM images. Especially, surface plasmon resonance of CuNP colloidal solution (CuNP diameter is around 2 nm) could be observed by using UV–Vis spectra. Our finding could offer a new synthetic approach to produce several kinds of metallic nanoparticles with small size, high stability, and in short, period of time in production.
Acknowledgements
This research is partly funded by Department of Science and Technology - Dongnai province.
References
- Sharon M, Choudhary AK, Kumar R. Nanotechnology in agricultural disease and food safety. J Phytopathol. 2010;2:83–92.
- Mrinmoy De, Partha SG, Vincent MR. Applications of nanoparticles in biology. Adv Mater. 2008;20:4225–4241.
- Lambert S, Cellier C, Gaigneaux EM, Pirard JP. Ag/SiO2, Cu/SiO2 and Pd/SiO2 cogelled xerogel catalysts for benzene combustion: relationships between operating synthesis variables and catalytic activity. Catal Commun. 2007;8:1244–1248.
- Huang HH, Yan FQ, Kek YM, Chew CH, Xu GQ, Ji W, Oh PS, Tang SH. Synthesis, characterization, and nonlinear optical properties of copper nanoparticles. Langmuir. 1997;13:172–175.
- Abhilash M. Potential applications of nanoparticles. Int J Pharma Bio Sci. 2010;1–12.
- Liu MS, Lin MCC, Sai CYT, Wang CC. Enhancement of thermal conductivity with Cu for nanofluids using chemical reduction method. Int J Heat Mass Transf. 2006;49:3028–3033.
- Nada EA, Masoud Z, Hijazi A. Natural convection heat transfer enhancement in horizontal concentric annuli using nanofluids. Int Commun Heat Mass Transf. 2008;35:657–665.
- Yu W, France DM, Routbort JL., Choi SUS. Review and comparison of nanofluid thermal conductivity and heat transfer enhancements. Heat Transf Eng. 2008;29:432–460.
- Li D, Sutton D, Burgess A, Graham D, Calvert P. Conductive copper and nickel lines via reactive inkjet printing. J Mater Chem. 2009;22: 3719–3724.
- Jeong SH, Song HC, Lee WW, Lee SS, Choi YM, Son W, Kim ED, Paik Hoon HC, Oh SH, Ryu HB. Stable aqueous based Cu nanoparticle ink for printing well-defined highly conductive features on a plastic substrate. Langmuir. 2011;27:3144–3149.
- Tarasov S, Kolubaev A, Belyaev S, Lerner M, Tepper F. Study of friction reduction by nanocopper additives to motor oil. Wear. 2002;252:63–69.
- Jayesh PR, Chatterjee AK, Siddhartha PD, Mukherji S. Strain specificity in antimicrobial activity of silver and copper nanoparticles. Acta Biomater. 2008;4:707–716.
- Kalyan Kamal SS, Sahoo PK, Vimala J, Manivel Raja M, Durai L, Ram S. Sacrificial seed mediated synthesis of copper nanopots by a modified polyol process. J Exp Nanosci. 2011;6:422–431.
- Mustafa B, Şişman I. Controlled synthesis of copper nano/microstructures using ascorbic acid in aqueous CTAB solution. Powder Technol. 2010;198:279–284.
- Park BK, Jeong S, Kim D, Moon J, Lim S, Kim JS. Synthesis and size control of monodisperse copper nanoparticles by polyol method. J Colloid Interf Sci. 2007;311:417–424.
- Nasibulin AG, Ahonen PP, Richard O, Kauppinen EI, Altman IS. Copper and copper oxide nanoparticle formation by chemical vapor nucleation from copper (II) acetylacetonate. J Nanopart Res. 2001;3:385–400.
- Zhu H, Zhang C, Yin Y. Novel synthesis of copper nanoparticles: influence of the synthesis conditions on the particle size. Nanotechnology. 2005;16:3079–3083.
- Blosi M, Albonetti S, Dondi M, Martelli C, Baldi G. Microwave-assisted polyol synthesis of Cu nanoparticles. J Nanopart Res. 2011;13:127–138.
- Pham QL, Sohn HJ, Park HJ, Hyun KS, Lee CB. Comparative study on the preparation of conductive copper pastes with copper nanoparticles prepared by electron beam irradiation and chemical reduction. Radiat Phys Chem. 2011;80:638–642.
- Al-Mamun MA, Kusumoto Y, Manickavachagam M. Simple new synthesis of copper nanoparticles in water/acetonitrile mixed solvent and their characterization. Mater Lett. 2009;63:2007–2009.
- Senthil Kumaran CK, Agilan S, Velauthapillai D, Muthukumarasamy N, Thambidurai M, Senthil TS. Preparation of copper cactus like structure by chemical method. J Nanosci Nanotechnol. 2012;1:14–18.
- Zhang XH, Siegert U, Liu R, Cai WB. Facile fabrication of ultrafine copper nanoparticles in organic solvent. Nanoscale Res Lett. 2009;4:705–708.
- Derrick M, Jeffrey G, Wang LY, Luo Jin J, Zhong CJ. Synthesis of size-controlled and shaped copper nanoparticles. Langmuir. 2007;23:5740–5745.
- Zhang QL, Yang ZM, Ding BJ, Lan XZ, Guo YJ. Preparation of copper nanoparticles by chemical reduction method using potassium borohydride. Trans Nonferrous Met Soc China. 2010;20:240–244.
- Xiong J, Wang Y, Xue QJ, Wu XD. Synthesis of highly stable dispersions of nanosized copper particles using L-ascorbic acid. Green Chem. 2001;13:900–904.
- Masoud SN, Fatemeh D, Noshin M. Synthesis and characterization of metallic copper nanoparticles via thermal decomposition. Polyhedron. 2008;27:3514–3518.
- Majzik A, Fülöp L, Csapó E, Bogár F, Martinek T, Penke B, Bíró G, Dékány I. Functionalization of gold nanoparticles with amino acid, beta-amyloid peptides and fragment. Colloids Surf B Biointerfaces 2010;81:235–241.
- Samim M, Kaushik NK, Maitra A. Effect of size of copper nanoparticles on its catalytic behaviour in Ullman reaction. Bull Mater Sci. 2007;30:535–540.
- Xu Y, Dong Y, Jiang X, Zhu N. Colorimetric detection of trivalent chromium in aqueous solution using tartrate-capped silver nanoparticles as probe. J Nanosci Nanotechnol. 2013;13:6820–6825.
- Panigrahi S, Kundu S, Ghosh SK, Nath S, Praharaj S, Basu S, Pal T. Selective one-pot synthesis of copper nanorods under surfactantless condition. Polyhedron. 2006;25:1263–1269.
- Garces LJ, Hincapie B, Makwana VD, Laubernds K, Sacco A, Suib SL. Effect of using polyvinyl alcohol and polyvinyl pyrrolidone in the synthesis of octahedral molecular sieves. Micropor Mesopor Mater. 2003;63:11–20.