Abstract
In this work, the interaction between agitation rate and oxidative etching on the growth mechanisms of silver nanowires during polyol process, the most conventional applied method for metallic nanoparticles synthesis, is evaluated. It was found that the main reason for the formation of multidiameter nanowires (MDNWs) is the local accumulation of silver ions in the system, i.e. even at a low concentration of silver ions (94 mM) under the mild agitation conditions, MDNWs were producible. Moreover, it was inferred that the more stress (stemming from the high agitation) applied on the initially formed decahedron Ag particles affects the ‘re-entrant grooves opening’ process which results in the formation and growth of irregular nanostructures. A polynomial equation for tuning aspect ratio of silver nanowires using different concentration of CuCl2 solution was also proposed. Finally, based on the obtained experimental data together with the thermodynamic considerations, a growth mechanism was proposed which can promisingly be employed for describing the one-dimensional growth of silver nanowires in both surfactant-modified and surfactant-free procedures.
1. Introduction
The versatile fields of applications for nanomaterials, such as optics, electronics and biology can be attributed to their size-dependent properties.[Citation1] Control of morphology and size of the nanocrystals, by the use of various chemical routes, for achieving favourable properties has become an attractive area of research during past decades.[Citation2–10] In chemical synthesis processes, generally, the controlling and monitoring of the shape and/or size of nanomaterials are successfully achievable by adjusting the reaction parameters.[Citation11]
Correct identification of the growth mechanisms during polyol process – a capable synthesis route for producing noble metal nanocrystals with different shapes, such as sphere, cube, rod and wire [Citation11–15] – is a prerequisite for the study of morphological evolutions. Moreover, an ideal archetype for investigating the growth mechanisms and twin crystals is silver due to its simple structure.[Citation16]
The growth mechanisms and the morphological features of silver nanocrystals have been studied by several researchers. It was reported that silver one-dimensional (1D) nanostructure consists of arranged subunits around a fivefold axis instead of a single crystal.[Citation17] The uniaxial growth of silver nanowires was illustrated based on Ag:O coordination of the absorbed polyvinylpyrrolidone (PVP) on Ag nanowires' surfaces.[Citation18] Furthermore, the growth mechanisms of Ag nanostructures via microwave-polyol method in the presence of Pt as the catalyst have been studied.[Citation19,Citation20] They showed that PVP tends to be absorbed on the {111} facets at short heating time. Visaveliya and Kohler [Citation21] realised the key role of ascorbic acid for tuning the aspect ratio during synthesis process of silver microrods. Additionally, it was reported that the synthesis parameters have important roles in the shape transformation of nanostructures.[Citation22,Citation23]
The shape evolution of a metallic nanocrystal is generally governed by thermodynamic and/or kinetic aspects of the synthesis process which can be regarded as a typical Ostwald ripening example. The thermodynamic aspect is mainly ascribed to the minimum interfacial energy for a definite volume, while the kinetic aspect is principally attributed to the surface diffusion of atoms. The latter can be enhanced by the application of heating, stirring and oxidative etching.[Citation11] Since multiply twinned particles (MTPs) with decahedral shape are thermodynamically stable structures, the correct and precise control of the kinetic parameters in the synthesis processes is essential for attaining favourable shapes. The desired vaterite crystals were successfully achieved by the control of kinetic and thermodynamic conditions using different precursor molar ratios.[Citation24] Furthermore, the driving force required for the shape evolution of NiS2 minicrystals has been estimated.Citation[42] However, there is limited information about the thermodynamic aspects of silver nanowires synthesised via polyol method.
Regarding the kinetic aspect of the process, Cobley et al. [Citation23] have employed a rapid injection of precursors to achieve desired silver nanostructures. Additionally, the use of appropriate oxidative/chemical etchant can be considered as an important strategy for governing the shape of nanocrystals. Distribution of single-crystal and multiple-twinned seeds can be controlled by the use of oxidative etching.[Citation25] Urchin-like WO3.H2O hollow spheres were successfully synthesised by employing appropriate chemical etchant.[Citation26] Moreover, gold nanocrystals with desired shape and size have been produced by applying proper oxidative etching of AuCl4− and Cl−anions.[Citation19,Citation20]
Another important factor for controlling the kinetics of a synthesis process is stirring of the reaction system. The crystal morphology of CaCO3 is simply controlled by variation of stirring conditions.[Citation27] Moreover, the effect of stirring conditions on the synthesis of TiO2 and TiO2/titanate hybrid nanostructures has been studied by Santara and Giri.[Citation28] They showed that nanorods/nanowires are achievable in highly alkaline water–ethanol mixed solvent. It has been reported that stirring has an important role in the morphology and optical properties of gold nanorods.[Citation29] A survey of previous literature on this case provides some clues about the effects of this parameter on the crystallisation field, colloids size distribution, crystallisation rate and crystallisation conditions that has been rarely discussed.[Citation30]
The difference in the values reported for the above-mentioned factors can be explained by the various materials synthesised in these works, and also the level of the other pertinent factors employed in these studies. Furthermore, the appropriate level of a factor might also be influenced by the level of the other factors in the synthesis process. In experimental parlance, there might be an interaction between factors in such a process. A survey of the published literature on Ag nanowire synthesis provides limited clues whether such interaction between agitation rate and oxidative etching exists or not. The aim of this work is to identify the interactive role of these two factors in the growth mechanisms of silver nanowires. Moreover, a supporting mechanism based on preliminary thermodynamic study for uniaxial growth of silver nanowires was also discussed.
2. Materials and methods
2.1. Reagents and solutions
Silver nitrate 99.9% (AgNO3) from Scharlau (Spain), copper chloride dihydrate99.9% (CuCl2.2H2O) and ethylene glycol (EG) from Merck, PVP 99.9% (K-30, MW ≈ 40,000 g/mol) from Sigma–Aldrich (USA) were used without further purification.
2.2. Synthesis procedure
A polyol synthesis consists of heating a polyol with a salt precursor and a polymeric capping agent to produce metal colloids.[Citation31] In the case of silver nanostructures, EG, AgNO3 and PVP serve as the polyol, salt precursor and polymeric capping agent, respectively. In a typical synthesis, 10 mL of EG was heated at 160 °C for one hour. Then, 80 μL of 4 mM CuCl2.2H2O/EG solution was added, and the solution was heated for 15 minutes. 3 mL of 114 mM PVP/EG was then added to the solution followed by 3 mL of 94 mM AgNO3/EG in a fixed rate (0.047 mmol/min). During the whole process, magnetic stirring rate was kept constant at three values of 0, 260 and 2000 rpm which are specified as A, B and C conditions, respectively. The reaction was stopped after one hour by dipping the vials in cold water. The products were washed three times with ethanol and three times with deionised water before further characterisation.
In order to study the effect of oxidative etching in a salt-mediated polyol process, CuCl2 solutions in various concentrations were used. CuCl2 concentration was varied from 0.1 to 2 mM by ∼1 mM steps to determine the role of oxidative etchant addition.
2.3. Characterisation
A Philips XL 30 scanning electron microscopy (SEM) at 25 keV was used for morphological studies of the synthesised nanostructures and the results are depicted in . Moreover, UV–visible absorption spectra of the nanostructures were obtained (0.003 gram of every sample was dispersed in deionised water and used for characterisation), . X-ray diffraction (XRD) patterns of nanostructures were obtained using a Philips PW 1140 XRD unit, . The XRD patterns indicate four diffraction peaks observed at 2θ = 38.3°, 44.4°, 64.5° and 77.4°, corresponding to (111, 200, 220) and (311) planes of the face-centred cubic (fcc) Ag nanostructures (JCPDS File No. 04-0783).
Figure 1. SEM images of synthesised silver nanostructures obtained (A) without stirring, (B) stirring at 260 rpm and (C) stirring at 2000 rpm. Bottom-right schematic of each image represents major nanostructures.
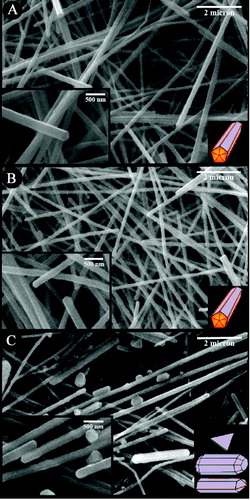
3. Results and discussion
3.1. Effect of agitation
The rate of physicochemical processes, such as crystallisation, can be effectively influenced by agitation conditions. In order to clarify the effect of agitation rate, magnetic stirring rate was set at three values of 0, 260 and 2000 rpm specified as A, B and C conditions, respectively. In the stagnant situation (case A), average diameter of the formed silver nanowires was ∼216 nm. However, in case B, where the stirring rate was 260 rpm, the average diameter of silver nanowires decreased to ∼139 nm which can be attributed to the homogenisation effect of stirring rate that hinders local increment of Ag+ concentration in the solution. Moreover, the presence of some multidiameter nanowires (MDNWs) under the stagnant conditions () indicates the inefficient stirring condition. The formation of such structures is ascribed to the high concentration of silver ions (≥250 mM) in the solution.[Citation32] However, our findings reveal that it is possible to obtain MDNWs at low concentration of silver ions (94 mM); thus, the low agitation level is the main reason for the formation of MDNWs which results in the local increment of Ag+ in the solution.
The higher stirring rate leads to more favourable conditions for nucleation and growth of nanowires. It is worthy of note that previous research reported a continuous decrease in diameter of nanowires by increasing stirring rate.[Citation33] However, according to the results obtained in the studied levels of agitation rate, various sizes and shapes of nanostructures are produced when the level of agitation is the highest (2000 rpm).
Moreover, UV–Vis spectroscopy analysis shows that the same morphology of nanostructures was obtained in cases A and B. Based on spectra showed in , obtained nanostructures in case C is mainly a mixture of null and 1D nanostructure.
Regarding the crystal growth aspect (the tip angle of a tetrahedron is 70.53°), assembling five tetrahedrons results in a 7.35° misfit gap in the final decahedron ((a)). This misfit increases the intrinsic energy in whole crystallite as it grows. For further growth of the decahedron crystallites, they must undergo a stress-relaxation process. Li et al. [Citation16] reported that stress-relaxation process proceeds via opening re-entrant grooves at the boundaries ((b)). The final irregular nanostructures is the consequence of the decahedrons growth comprising of some extra {100} and {111} facets. Based on the results obtained in this work, it is most likely that high agitation rate also influences the ‘re-entrant grooves opening’ by applying more stress on the initially formed decahedron particles. By increasing the agitation rate and keeping the CuCl2 concentration constant, the opening re-entrant grooves are accelerated due to the combination of misfit strain energy and stress. These findings are supported by the formation of irregular nanostructures containing additional {100} and {111} facets on their surfaces ((c)).
3.2. Thermodynamic of the growth mechanisms
From a thermodynamic standpoint, since the geometrical shape of an as-formed seed is unstable (due to surface atoms' fluctuations), the interfacial energy should be minimised during the shape evolution of a seed.[Citation11] The interfacial energy for a given system at constant temperature and pressure is defined as
(1) where G and A are the Gibbs free energy and the interfacial area, respectively. Also, K, ϵ and ρa stand for the number of broken bonds, bond strength and density of surface atoms, respectively. According to Xia et al.,[Citation11] the surface energy corresponding to the crystallographic facets of silver with fcc structure and lattice constant of ‘a’, can be presented as
(2)
(3)
(4)
Since interfacial energy of {111} facets is lower than those corresponding to {100} and {110} facets, the crystallographic transitions results in preferable formation of {111} facets.[Citation11,Citation34]
As a matter of knowledge, EG acquires its reducing power at around 160 °C. By introducing silver ions into the system, the reduction process starts and the nucleation process can occur. The phase diagram presented in reveals that by the growth of nuclei, the structure tends to adopt different morphologies to minimise the surface energy. These changes in the morphology bring forth certain defects in the nuclei. In the case of silver nuclei, the twin boundaries are the major defects known as singly and multiply twinned structures.[Citation35] According to , the thermodynamically favoured morphology at ∼440 K is decahedral MTP. Due to the presence of twinned defects on the surface of MTPs, Ag atoms favourably nucleate on the {111} facets. Despite the fact that in many studies the uniaxial growth of 1D silver nanostructure is attributed to the effect of capping agent, such as PVP and citrate,[Citation18–20,Citation36] other mechanisms are undoubtedly involved when no capping agent is used.[Citation37,Citation38]
Figure 6. Phase diagram for small particles. Modified with permission from [Citation35].
![Figure 6. Phase diagram for small particles. Modified with permission from [Citation35].](/cms/asset/ebe4d80b-49df-44bd-8eff-86056454c912/tjen_a_1014872_f0006_b.gif)
In a recent study, it was proposed that the addition of atoms in a decahedron, far from central axis, leads to an increase in strain energy, while the elongation of decahedron parallel to twin planes does not increase the strain energy.[Citation39] In other words, the atoms that tend to nucleate on Ag surfaces far from central axis could not overcome the strain energy barrier; thus, the 1D growth of silver decahedral is due to the fact that the addition of the atoms only occurs near the central axis. By the use of a capping agent that interacts more strongly with {100} side facets of a decahedral MTP, compared with {111} facets, uniaxial growth is more encouraged. The above proposed/discussed mechanism is summarised in .
3.3. Role of oxidative etching
Lofton and Sigmund [Citation40] stated that in the presence of Cl−/O2 pair in the medium, the etching of MTPs becomes the predominant process because of the reactive nature of such structures (the presence of the twin boundary defects on surfaces). Regarding the fact that decahedral MTPs are vital for the formation of nanowires, oxidative etching must be inhibited. When capping agents such as PVP are present in the system, there are only two possible ways for saving MTPs, removing the oxygen from the solution via an inert gas or diminishing the efficiency of oxidative etching by introducing some salts such as CuCl2 into the solution. In our previous work,[Citation22] we successfully developed a statistical model for controlling aspect ratio of silver nanowires in a polyol process by tuning AgNO3 addition rate and concentration of Cl− in the solution. For a constant addition rate of AgNO3 in the studied range (0.047–0.47 mmol/min) and an agitation rate of 260 rpm, the equation is as follows:
(5) where d and l are the diameter and length of the obtained nanostructures, respectively. By plotting the aspect ratio of silver nanowires versus CuCl2 concentration (), it can be observed that a minimum level of CuCl2 (≥1 mM) is essential for the formation of 1D nanostructures.
Figure 8. Plot of aspect ratio of silver nanostructures versus CuCl2 concentration in a constant stirring rate of 260 rpm.
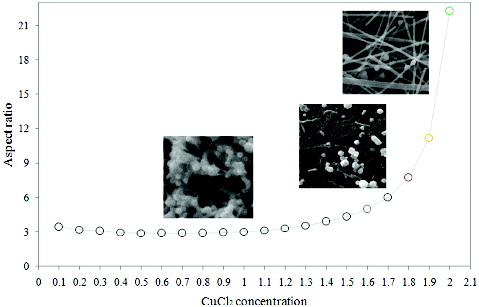
In order to verify the role of CuCl2 solution, three experiments with different concentrations of CuCl2 (0.1, 1 and 2 mM) were conducted. The aspect ratios of the obtained nanostructures were 2.9, 3 and 22, which is in agreement with the prediction of the model ().
Figure 9. SEM results for samples with different concentrations of CuCl2 solution: (a) 0.1mM, (b) 1mM and (c) 2mM.
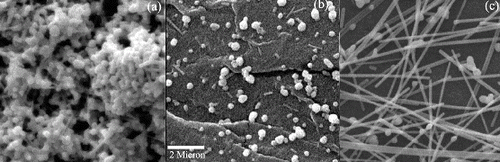
As CuCl2 is introduced into the EG medium, Cu++ ions are reduced to Cu+. The oxygen atoms present in the system are absorbed and dissociated over the Ag seeds, this results in blocking the sites for further Ag deposition. The Cu+ ions can effectively scavenge the adsorbed oxygen (Oa) through an oxidation reaction (Cu+/Cu++ pair). Again, EG reduces Cu++ to Cu+ which provides the conditions of continuous oxygen removal. Moreover, the oxygen scavenging role of Cu++/+ redox pairs allows the MTPs to grow.[Citation11,Citation14,Citation19,Citation20]
Another important point regarding introducing CuCl2 in polyol synthesis process of silver is the role of Cl− ions. The presence of Cl− reduces the concentration of free Ag+ ions in the solution via the formation of AgCl which improves the electrostatic stability of the initially formed Ag seeds.[Citation41] The lower level of Ag+ at initial Ag seed formation stage and the following slow release of Ag+ lead to a well-regulated reagent delivery system. Moreover, such conditions facilitate the high-yield formation of stable multiply twinned Ag seeds required for wire growth.
As it is shown in and , CuCl2 concentration plays an important role in final morphology of nanostructures. Final nanostructures are (1) nanoparticles, (2) nanorods/nanoparticles and (3) nanowires. Nanowires with aspect ratio of ∼25 were achieved in the presence of 2 mM CuCl2 ((c)).
Close observation of shows that at rigorous agitation conditions (case C), oxidative etching occurs significantly, despite the presence of Cu++/+ redox pairs in the solution. The obtained product in this case is a mixture of various nanostructures, as shown in . Thus, it seems that the agitation condition and Cu++/+ redox pair have an interaction with each other during the growth of Ag nanostructures. This interaction can be attributed to the effect of agitation on the transfer rate of solutes in the solution, i.e. the higher agitation level leads to an increase in the oxygen transfer rate.
To sum up, based on the above discussions, it can be stated that the rigorous agitation can increase the exposure of nanostructures to oxygen, which remarkably enhances the oxidative etching process. On the other hand, although the higher agitation rate provides more favourable conditions for chemisorption of PVP on {100} facets – which would expectedly result in mono-dispersed nanostructures in terms of shape and size – a set of nanostructures with different sizes and shapes are achieved (). Using optimal level of agitation rate (260 rpm) different silver nanostructures (particles, rods and wires) with aspect ratios of 1, ∼3 and ∼25 achieved successfully by varying the concentration of CuCl2 solution (0.1, 1 and 2 mM, respectively) and showed in . Moreover, it can be reasonably inferred that another mechanism (), other than the role of PVP, is involved in uniaxial growth of Ag nanowires.
4. Conclusions
In this study, the simultaneous influence of agitation rate and oxidative etching during polyol synthesis process of Ag nanowires was elucidated from a mechanistic viewpoint with the following results.
Variation in agitation rate (0, 260 and 2000 rpm), not only results in different aspect ratios of 1D nanostructure (nanorod to nanowire), but also it can significantly stimulate the formation of nanoparticles. Furthermore, this factor can lead to the production of a mixture of one-dimensional and zero-dimensional structures.
The level of CuCl2 concentration (in the range of 0.1–2mM) governs the structure of final products. The d/l ratio of the as synthesised nanostructures follows the following equation
Both the agitation rate and oxidative etching level have a significant contribution to the morphology of the Ag nanostructures. At high level of CuCl2 concentration, the structural features and dispersion quality of the formed nano-sized Ag are significantly affected by the level of agitation rate, while the level of CuCl2 in the solution has no remarkable effect on the morphology in a non-agitated system.
In the absence of PVP, the strain energy is responsible for uniaxial formation of Ag nanowires regarding the thermodynamic considerations.
Disclosure statement
No potential conflict of interest was reported by the authors.
References
- El-Sayed MA. Some interesting properties of metals confined in time and nanometer space of different shapes. Acc Chem Res. 2001;34:257–264.
- Gholivand MB, Malekzadeh G, Derakhshan AA. Boehmite nanoparticle modified carbon paste electrode for determination of piroxicam. Sensor Actuators B. 2014;201:378–386.
- Gholivand MB, Jalalvand AR, Paimard G, Goicoechea HC, Skov T, Farhadi R, Ghobadi S, Moradi N, Nasirian V. Fabrication of a novel naltrexone biosensor based on a computationally engineered nanobiocomposite. Int J Biol Macromol. 2014;70:596–605.
- Gholivand MB, Shamsipur M, Paimard G, Feyzi M, Jafari F. Synthesis of Fe–Cu/TiO2 nanostructure and its use in construction of a sensitive and selective sensor for metformin determination. Mater Sci Eng C. 2014;42:791–798.
- Ko H-H, Yang G, Cheng H-Z, Wang M-C, Zhao X. Growth and optical properties of cerium dioxide nanocrystallites prepared by coprecipitation routes. Ceram Int. 2014;40:4055–4064.
- Ko H-H, Yang G, Wang M-C, Zhao X. Isothermal crystallization kinetics and effect of crystallinity on the optical properties of nanosized CeO2 powder. Ceram Int. 2014;40:6663–6671.
- Lyubutin IS, Starchikov SS, Lin C-R, Lu S-Z, Shaikh MO, Funtov KO, Dmitrieva TV, Ovchinnikov SG, Edelman IS, Ivantsov R. Magnetic, structural, and electronic properties of iron sulfide Fe3S4 nanoparticles synthesized by the polyol mediated process. J Nanopart Res. 2013;15:1397–1410.
- Mendes PM. Cellular nanotechnology: making biological interfaces smarter. Chem Soc Rev. 2013;42:9207–9218.
- Ma X, Zhu X, You F, Feng J, Wang M-C, Zhao X. Preparation and optical polarization of Ag/epoxy composite films with aligned Ag nanowires. J Alloys Compd. 2014;592:57–62.
- Mao H, Feng J, Ma X, Wu C, Zhao X. One-dimensional silver nanowires synthesized by self-seeding polyol process. J Nanopart Res. 2012;14:887–892.
- Xia Y, Xiong Y, Lim B, Skrabalak SE. Shape-controlled synthesis of metal nanocrystals: simple chemistry meets complex physics? Angew Chem Int Ed. 2009;48: 60–103.
- Luo X, Chen Y, Yang D, Li Z, Han Y. Morphology control of silver nano-crystals through a polyol synthesis. Solid State Sci. 2011;13:1719–1723.
- Ungelenk J, Speldrich M, Dronskowski R, Feldmann C. Polyol-mediated low-temperature synthesis of crystalline tungstate nanoparticles MWO4 (M = Mn, Fe, Co, Ni, Cu, Zn). Solid State Sci. 2014;31:62–69.
- Wiley B, Sun Y, Xia Y. Synthesis of silver nanostructures with controlled shapes and properties. Acc Chem Res. 2007;40:1067–1076.
- Wu G, Zhou Y, Gao X, Shao Z. Facile low-temperature polyol process for LiFePO4 nanoplate and carbon nanotube composite. Solid State Sci. 2013;24:15–20.
- Li CR, Lu NP, Xu Q, Mei J, Dong WJ, Fu JL, Cao ZX. Decahedral and icosahedral twin crystals of silver: formation and morphology evolution. J Cryst Growth. 2011;319:88–95.
- Hofmeister H, Nepijko SA, Ievlev DN, Schulze W, Ertl G. Composition and lattice structure of fivefold twinned nanorods of silver. J Cryst Growth. 2002;234:773–781.
- Gao Y, Song L, Jiang P, Liu LF, Yan XQ, Zhou ZP, Liu DF, Wang JX, Yuan HJ, Zhang ZX, Zhao XW, Dou XY, Zhou WY, Wang G, Xie SS, Chen HY, Li JQ. Silver nanowires with five-fold symmetric cross-section. J Cryst Growth. 2005;276:606–612.
- Tsuji M, Miyamae N, Hashimoto M, Nishio M, Hikino S, Ishigami N, Tanaka I. Shape and size controlled synthesis of gold nanocrystals using oxidative etching by AuCl4− and Cl− anions in microwave-polyol process. Colloids Surf A. 2007;302:587–598.
- Tsuji M, Nishizawa Y, Matsumoto K, Miyamae N, Tsuji T, Zhang X. Rapid synthesis of silver nanostructures by using microwave-polyol method with the assistance of Pt seeds and polyvinylpyrrolidone. Colloids Surf A. 2007;293:185–194.
- Visaveliya N, Kohler JM. A self-seeding synthesis of Ag microrods of tuned aspect ratio: ascorbic acid plays a key role. Nanotechnology. 2013;24:345604–345615.
- Amirjani A, Marashi P, Fatmehsari DH. Effect of AgNO3 addition rate on aspect ratio of CuCl2-mediated synthesized silver nanowires using response surface methodology. Colloids Surf A. 2014;444:33–39.
- Cobley CM, Rycenga M, Zhou F, Li ZY, Xia Y. Etching and growth: an intertwined pathway to silver nanocrystals with exotic shapes. Angew Chem Int Ed. 2009;48:4824–4827.
- Jin D, Wang F, Yue L. Phase and morphology evolution of vaterite crystals in water/ethanol binary solvent. Cryst Res Technol. 2011;46:140–144.
- Wiley B, Herricks T, Sun Y, Xia Y. Polyol synthesis of silver nanoparticles: use of chloride and oxygen to promote the formation of single-crystal, truncated cubes and tetrahedrons. Nano Lett. 2004;4:1733–1739.
- Zeng Q, Zhao Y, Zhao J, Hao X, Lu Y, Guo J, Song Y, Gao F, Huang Z. Studies on fabrication of urchin-likeWO3·H2O hollow spheres and their photocatalytic properties. Cryst Res Technol. 2013;5:334–343.
- Yan FW, Zhang SF, Guo CY, Zhang XH, Chen GC, Yan F, Yuan GQ. Influence of stirring speed on the crystallization of calcium carbonate. Cryst Res Technol. 2009;44:725–728.
- Santara B, Giri PK. Impact of reaction temperature, stirring and cosolvent on the solvothermal synthesis of anatase TiO2 and TiO2/titanate hybrid nanostructures: elucidating the growth mechanism. Mater Chem Phys. 2013;137:928–936.
- García MA, Bouzas V, Carmona N. Influence of stirring in the synthesis of gold nanorods. Mater Chem Phys. 2011;127:446–450.
- Gavezzotti A. Crystal formation and stability: physical principles and molecular simulation. Cryst Res Technol. 2013;48:1–18.
- Fievet F, Lagier JP, Figlarz M. Preparing monodisperse metal powders in micrometer and submicrometer sizes by the polyol process. MRS Bull. 1989;14:29–34.
- Zhang W, Chen P, Gao Q, Zhang Y, Tang Y. High-concentration preparation of silver nanowires: restraining in situ nitric acidic etching by steel-assisted polyol method. Chem Mater. 2008;20:1699–1704.
- Coskun S, Aksoy B, Unalan HE. Polyol synthesis of silver nanowires: an extensive parametric study. Cryst Growth Des. 2011;11:4963–4969.
- Sun Y, Xia Y. Shape-controlled synthesis of gold and silver nanoparticles. Science. 2002;298:2176–2179.
- Ajayan PM, Marks LD. Quasimelting and phases of small particles. Phys Rev Lett. 1988;60:585–587.
- Zhao Y, Chen A, Liang S. Shape-controlled synthesis of silver nanocrystals via γ-irradiation in the presence of poly(vinylpyrrolidone). J Cryst Growth. 2013;372:116–120.
- Caswell KK, Bender CM, Murphy CJ. Seedless, surfactantless wet chemical synthesis of silver nanowires. Nano Lett. 2003;3:667–669.
- Tetsumoto T, Gotoh Y, Ishiwatari T. Mechanistic studies on the formation of silver nanowires by a hydrothermal method. J Colloid Interface Sci. 2011;362:267–273.
- Patala S, Marks LD, Cruz MO. Elastic strain energy effects in faceted decahedral nanoparticles. J Phys Chem C. 2013;117:1485–1494.
- Lofton C, Sigmund W. Mechanism controlling crystal habits of gold and silver colloids. Adv Funct Mater. 2005;15:1197–1208.
- Korte KE, Skrabalak SE, Xia Y. Rapid synthesis of silver nanowires through a CuCl- or CuCl2-mediated polyol process. J Mater Chem. 2008;18:437–441.
- Zhou X-F, Lin L, Wang W, Wang S-Y. Shape evolution of NiS2 minicrystals via thermodynamic controlled growth. J Cryst Growth. 2011;314:302–305.