Abstract
Interest in the photocatalytic oxidation of formaldehyde from contaminated wastewater is growing rapidly. The photocatalytic activity of the nanocrystalline Fe3+/F− co-doped TiO2–SiO2 composite film for the degradation of formaldehyde solution under visible light was discussed in this study. The films were characterised by field emission scanning electron microscopy (FE-SEM) equipped with energy-dispersive spectroscopy, X-ray diffraction (XRD), BET surface area, UV–Vis absorption spectroscopy, and photoluminescence spectroscopy. The FE-SEM results revealed that the Fe3+/F− co-doped TiO2–SiO2 film was composed of uniform round-like nanoparticles or aggregates with the size range of 5–10 nm. The XRD results indicated that only the anatase phase was observed in the film. Compared with a pure TiO2 film and a singly modified TiO2 film, the Fe3+/F− co-doped TiO2–SiO2 composite film showed the best photocatalytic properties due to its strong visible light adsorption and diminished electrons-holes recombination.
1. Introduction
Environmental pollution caused by volatile organic compounds, such as formaldehyde, is becoming a serious problem all over the world. However, it is difficult to remove them by traditional biological and physical treatment methods. Heterogeneous photocatalyst has attracted a great interest in the field of the degradation of toxic pollutants.[Citation1,Citation2] TiO2 is the most suitable material for heterogeneous photocatalysts owing to its long-term stability, high photocatalytic activity, non-toxicity, chemical and biological inertness, controllable structure and its low cost.[Citation3,Citation4] Recently, many studies have pointed towards easily recycled TiO2 films because conventional TiO2 powders are difficult to recover in wastewater treatment processes.[Citation5,Citation6]
TiO2 is a UV-absorbing material with a gap energy in the 3.0–3.4 eV range.[Citation7] Usually the band gap is equal to or greater than 3.2 eV for anatase, which means only under the irradiation of ultraviolet light can the reaction occur to create electrons and holes. Another problem is the high recombination rate of photogenerated electrons and holes. Many methods have been proposed to reduce the recombination of photogenerated electron-hole pairs and widen the light response region of TiO2. Doping TiO2 with foreign ions is one of the most promising strategies. Easy availability and low cost have given iron broad applications in the modification of TiO2. Wu et al. reported that Fe doping could enhance the intensity of absorption in the visible light region and make a red shift in the band gap transition of Fe-doped TiO2 samples.[Citation8] Transition metal doping can enhance the visible light response, but the problems of TiO2 with metal ion doping are only a small red shift, poor thermal stability, and surface aggregation rather than substitution. Doping with non-metal ions into TiO2 can provide a favourable visible light response and facilitate efficient charge carrier transfer processes. Yu et al. reported that F-doped TiO2 powders exhibited stronger absorption in the UV–visible range with a red shift in the band gap transition.[Citation9] Metal dopant energy levels are usually below the conduction band edge, while non-metal dopant energy levels are just above the top of the valence band of TiO2.[Citation10] Therefore, co-doping with metal and non-metal ions can effectively narrow the band gap of TiO2 and thus enhancing the photocatalytic activity of TiO2.[Citation11] Composite semiconductors are also a good method to improve the photocatalytic activity of TiO2. Kim et al.[Citation12] reported that the high photocatalytic activity of a Zr/TiO2/SiO2 photocatalyst could be attributed to the large specific surface area and strong absorption in the visible light region. With a high specific surface area, SiO2 can provide more effective adsorption sites in the SiO2–TiO2 composite system.
Considering the green environmental protection, we prepared the nanocrystalline Fe3+/F− co-doped TiO2–SiO2 composite film using the sol–gel method in this work. It is expected that the film may exhibit better photocatalytic properties for the photocatalytic degradation of formaldehyde in an aqueous solution. The improvement mechanism by Fe3+/F− co-doping and SiO2 composite was also discussed.
2. Experimental
TiO2 or SiO2 sol was prepared by a sol--gel method using tetrabutyl titanate [TiO(C4H9O)4] or tetraethylorthosilicate (TEOS) as the precursor, as previously reported.[Citation13,Citation14] One mL Ti(OC4H9)4 was added to 20 mL absolute C2H5OH. Then, 20 mL HNO3 (0.2M) was added to the above solution with vigorous stirring. One mL TEOS was added to 30 mL absolute ethanol. Then, 0.5 mL ammonium hydroxide was added to the above solution with vigorous stirring. TiO2–SiO2 composite sol was prepared by mixing TiO2 and SiO2 colloids in a 2/1 volume ratio with vigorous stirring. A controllable dip-coating device was used to prepare pure TiO2 or TiO2–SiO2 composite films on the surface of a glass substrate open to the atmosphere. Fe(NO3)3 or NH4F aqueous solutions were chosen as the iron or fluorine sources. The Fe3+, F−, or Fe3+/F− ions were doped onto the surface layer by coating a thin Fe3+, F−, or Fe3+/F− doped TiO2 sol onto a dried TiO2–SiO2 composite film. Finally, the films were calcined at 450 °C in air for 1 h in a horizontal furnace. The thickness of the film was 0.3–0.6 μm in our preparations.
The surface morphology of the sample was studied by a high-resolution field emission scanning electron microscopy (FE-SEM, Nova NanoSEM 450) equipped with an energy-dispersive spectroscopy analysis (EDS, X-Max 50) to determine the composition of the samples. The X-ray diffraction (XRD) profiles of the samples were recorded by XRD with a diffractometer (Bruker D8 FOCUS) employing Cu Kα radiation at a scan rate of 0.05° s−1 to characterise the phases and crystal size of the samples. The specific surface area of the sample was analysed using a nitrogen adsorption/desorption apparatus (ASAP2020). The UV–Vis diffuse reflectance spectra of the sample was recorded on a UV–Vis spectrophotometer (TU-1901) with an integrating sphere accessory (IS 19-1) using a blank glass plate as reference. The recombination rate of photogenerated electrons and holes in the sample was studied by photoluminescence (PL) emission spectra, recorded at room temperature using a spectrometer (FLS 920).
The photodegradation of formaldehyde (20 g L−1) in aqueous solutions was used to evaluate the photocatalytic activities of the samples. In each photocatalytic experiment a catalyst sample was placed into 5 mL aqueous solution of a target pollutant in a quartz beaker. A tungsten halogen lamp equipped with a UV cut-off filter (λ > 400 nm) was used as a visible light source whose average light intensity was 40 mW·cm−2. The distance between the beaker and the light source was kept at about 15 cm. The formaldehyde concentration was determined by an acetylacetone spectrophotometry. shows the adsorption curve of the samples in formaldehyde solutions. It is clear that the adsorption–desorption equilibrium has been reached after stirring in the dark for 10 min. in our case.
3. Results and discussion
3.1. Photocatalytic activity
Volatile organic compounds, such as formaldehyde, may cause a serious threat to groundwater, indoor environments, and even human health. Liquid effluents with high formaldehyde concentrations are difficult to treat using conventional processes.[Citation15] Interest in the photocatalytic oxidation of formaldehyde from contaminated wastewater is growing rapidly. shows the decomposition kinetics of formaldehyde solutions using pure TiO2, TiO2–SiO2, and Fe3+/F− co-doped TiO2–SiO2 films on glass substrates under visible light irradiation. From , it can be seen that the photocatalytic activity of the Fe3+/F− co-doped TiO2–SiO2 film is highest in three samples. The degradation rate of the formaldehyde solution using the Fe3+/F− co-doped TiO2–SiO2 film is about 57% under visible light irradiation for 200 min compared to pure TiO2 film 8% and TiO2–SiO2 film 18%. The photocatalytic activity of the Fe3+/F− co-doped TiO2–SiO2 film may be attributed to enhance visible light absorption efficiency and reduce the recombination rate of the photogenerated charges.
3.2. Photocatalyst characterisation
The UV–Vis absorption spectra (a) and plots of (αhν)1/2 versus energy (hν) (b) for pure TiO2, TiO2–SiO2, and Fe3+/F− co-doped TiO2–SiO2 films are presented in . From (a), the spectrum of the Fe3+/F− co-doped TiO2–SiO2 film shows a significant enhancement of light absorption at wavelengths of 400–700 nm compared to the pure TiO2 film and the TiO2–SiO2 film. Fe3+/F− co-doping can result from the formation of the dopant energy level within the band gap of TiO2. The levels introduced by F merge with the valence band of TiO2 whereas the states introduced by Fe are placed near the middle of the band gap of TiO2. Thus, a low concentration co-doping of cations and anions can effectively narrow the band gap thus enhancing the visible light absorption efficiency.[Citation11] The optical band gap can be deduced from the equation for a crystalline semiconductor, αhν = A(hν - Eg)n/2, where α, ν, Eg, and A are the absorption coefficient, light frequency, band gap, and a constant, respectively. The value of n is determined by the type of optical transition of a semiconductor. Therefore, the band gap energy of TiO2 can be determined from a plot of (αhν)1/2 versus energy (hν),[Citation16] as is shown in (b). The band gap of pure TiO2, TiO2–SiO, and Fe3+/F− co-doped TiO2–SiO film is 3.25, 3.15, and 2.85 eV, respectively. These data suggest that Fe3+/F− co-doping can result in an obviously narrowing of the band gap of TiO2. The lower band gap has a positive effect on the photocatalytic activity of TiO2.
Figure 3. (a) UV–Vis absorption spectra and (b) plots of (αhν)1/2 versus energy (hν) for pure TiO2, TiO2–SiO2, and Fe3+/F− co-doped TiO2–SiO2 films.
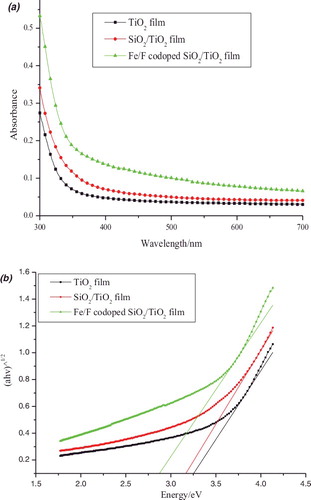
PL emission is related to the formation of photo-induced charge carriers and their recombination kinetics. shows the PL emission spectra of pure TiO2, TiO2–SiO2, and Fe3+/F− co-doped TiO2–SiO2 films. The PL intensity of the Fe3+/F− co-doped TiO2–SiO2 film is the lowest among the three samples indicating that the recombination of electrons and holes was effectively prohibited. In the Fe3+/F− co-doped TiO2–SiO2 composite system, Fe3+ can trap electrons to separate e−/h+ pairs whereas F− can adsorb holes to separate e−/h+ pairs. Especially, SiO2 can provide more effective adsorption sites in the SiO2–TiO2 composite system, so the photogenerated holes can reach them before recombination with electrons thus enhancing the photocatalytic activity of TiO2.[Citation17] This indicates that the synergistic effects of Fe3+, F−, and SiO2 can greatly reduce the recombination of photo-induced electrons and holes in TiO2.
Surface area is one of the important factors in the photocatalytic activity of TiO2 because the reaction takes places initially by the adsorption of O2 and H2O on the surface of the photocatalyst to generate oxidising agents by electrons and holes, respectively. shows the nitrogen adsorption/desorption isotherms of TiO2, TiO2–SiO2, and Fe3+/F− co-doped TiO2–SiO2 powders calcined at 450 °C in the air for 1 h. The specific surface areas of the samples are calculated according to the BET method. In our case, the specific surface area of pure TiO2, TiO2–SiO2, and Fe3+/F− co-doped TiO2–SiO2 are 95.9, 294.3, and 397.0 m2 g−1, respectively. The incorporation of SiO2 and Fe3+/F− into the TiO2 catalysts can reduce the particle size thus increasing the specific surface area, while the large surface area can effectively adsorb more H2O, O2, and pollutants onto the reaction sites of the catalysts.
Figure 5. N2 adsorption/ desorption isotherms of pure TiO2, TiO2–SiO2, and Fe3+/F − co-doped TiO2–SiO2 powders.
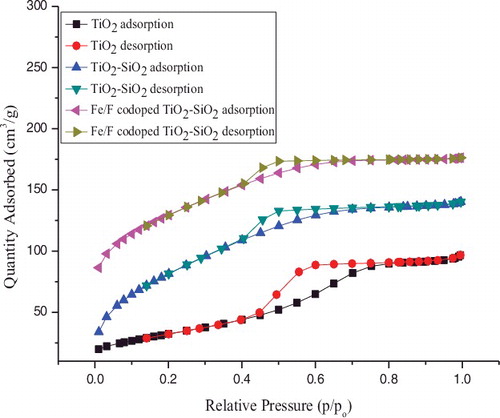
shows the XRD spectra of pure TiO2, Fe3+/F− co-doped TiO2–SiO2 samples calcined at 450 °C in air for 1 h. Pure TiO2 exhibit a mixed crystallinity composed of anatase (25.43°, 37.94°, and 48.08°), rutile (27.47° and 36.26°), and brookite (30.77°). However, only the anatase phase is observed in the Fe3+/F− co-doped TiO2–SiO2 sample. The introduction of SiO2 and the addition of Fe3+/F− can effectively suppress the anatase-to-rutile phase transformation and prevent the formation of brookite phases. This is because SiO2 is well dispersed in TiO2,[Citation12,Citation18] or atoms are well-inserted into the crystal lattice of TiO2 as interstitial atoms.[Citation19] However, the overall peak intensities of the anatase TiO2 decrease with the insertion of SiO2 and Fe3+/F−, indicating that the addition of SiO2 and Fe3+/F− can also effectively suppress the growth of TiO2 crystals. No SiO2 diffraction peaks are observed because the SiO2 is an amorphous phase at this temperature.[Citation20] No obvious Fe- and F-related compound peaks are observed indicating that the small amounts of Fe and F dopants are uniformly distributed on TiO2. It is well documented that the anatase phase has a higher photoactivity compared to rutile or brookite phases.[Citation21]
It is accepted that the photocatalytic activity of TiO2 is related to its surface morphology. The surface morphology of the Fe3+/F− co-doped TiO2–SiO2 film is very different from that of pure TiO2 film calcined at 450 °C in air for 1 h, as shown in . The surface of the Fe3+/F− co-doped TiO2–SiO2 film is smoother without cracks compared to that of a pure TiO2 film. Moreover, the multi-modified TiO2 film is composed of smaller spherical nanoparticles with a lower level of agglomeration compared to a pure TiO2 film. The amorphous silica framework segregates at the anatase TiO2 grain boundary and retards TiO2 species from aggregating together.[Citation22] EDS indicates that the weight per cents of Ti, O, Si, Fe, and F in the Fe3+/F− co-doped TiO2–SiO2 film are 23.24%, 55.38%, 17.31%, 1.02%, and 3.05%, respectively, as shown in .
Figure 7. FE-SEM images of (a) pure TiO2 and (b) Fe3+/F− co-doped TiO2–SiO2 composite films calcined at 450 °C in air for 1 h.
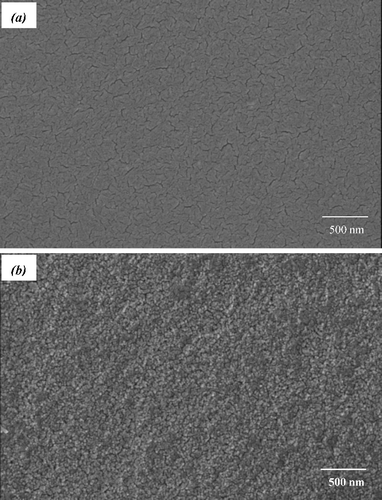
Figure 8. EDS images of the Fe3+/F− co-doped TiO2–SiO2 composite film calcined at 450 °C in air for 1 h.
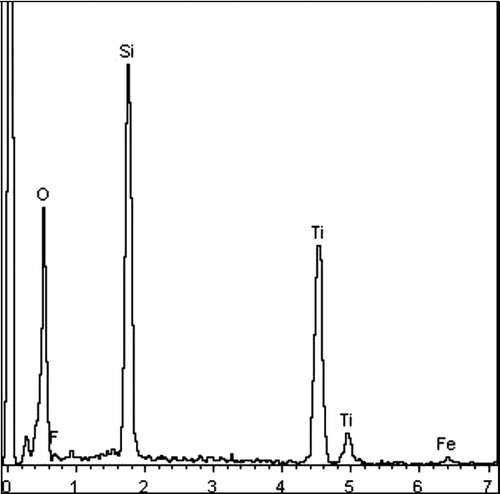
In a word, the Fe3+/F− co-doped TiO2–SiO2 film exhibit excellent photocatalytic activity in the degradation of formaldehyde under visible light irradiation. The enhanced visible-light activity may originate from the synergistic effects of Fe3+, F−, and SiO2. First, Fe3+/F− co-doping can decrease the band gap of the TiO2 from 3.25 to 2.85 eV, which may facilitate its absorption of visible light. Second, the synergistic effects of Fe3+, F−, and SiO2 can greatly reduce the recombination of photo-induced electrons and holes in TiO2 thus enhancing the photocatalytic activity of TiO2. Third, the large specific surface areas caused by Fe3+/F− co-doping and SiO2 compositing can improve the adsorption of the pollutants. These factors can result in excellent visible-light photocatalytic activity. The major steps in the mechanism may be summarised by Equations (Equation1(1) )–(Equation4
(4) ).[Citation1,Citation23–Citation25]
(1)
(2)
(3)
(4)
4. Conclusions
A highly photoactive nanocrystalline Fe3+/F− co-doped TiO2–SiO2 film has been successfully synthesised using the sol–gel method. The multi-modified TiO2 film shows strong visible light adsorption compared to a pure TiO2 film and a singly modified film. Experimental results show that the Fe3+/F− co-doped TiO2–SiO2 film is effective in the degradation of formaldehyde under visible light irradiation. The enhanced photocatalytic activity of the multi-modified TiO2 film can be understood on the basis of increasing photo generated species and reducing the recombination rate of electrons and holes.
Disclosure statement
No potential conflict of interest was reported by the authors.
Additional information
Funding
References
- Pelaez M, Nolan NT, Pillai SC, Seery MK, Falaras P, Kontos AG, Dunlop PSM, Hamilton JWJ, Byrne JA, O'Shea K, Entezari MH, Dionysiou DD. A review on the visible light active titanium dioxide photocatalysts for environmental applications. Appl Catal B. 2012;125:331–349.
- Mani AD, Muthusamy S, Anandan, S, Subrahmanyam Ch. C and N doped nano-sized TiO2 for visible light photocatalytic degradation of aqueous pollutants. J Exp Nanosci. 2015;10:115–125.
- Shen C, Wang YJ, Xu JH, Luo GS. Facile synthesis and photocatalytic properties of TiO2 nanoparticles supported on porous glass beads. Chem Eng J. 2012;209:478–485.
- Liu R, Yang W-D, Chung Z-J, Chueng H-J. Synthesis and application of TiO2 nanotubes in environmental pollutant removal. Int J Mater Res. 2014;105:397–403.
- Eshaghi A, Aghaei AA, Eshaghi A. Photocatalytic and self-cleaning properties of SiO2/TiO2/SiO2 nanostructured thin film. Int J Mater Res. 2013;104:1263–1266.
- Wang D, Li X, Chen J, Tao X. Enhanced photoelectrocatalytic activity of reduced graphene oxide/TiO2 composite films for dye degradation. Chem Eng J. 2012;198–199:547–554.
- Kubacka A, Fernandez-Garcia M, Colon G. Advanced nanoarchitectures for solar photocatalytic applications. Chem Rev. 2012;112:1555–1614.
- Wu YM, Zhang JL, Xiao L, Chen F. Preparation and characterization of TiO2 photocatalysts by Fe3+ doping together with Au deposition for the degradation of organic pollutants. Appl Catal B. 2009;88:525–532.
- Yu JC, Yu JG, Ho WK, Jiang ZT, Zhang LZ. Effects of F− doping on the photocatalytic activity and microstructures of nanocrystalline TiO2 powders. Chem Mater. 2002;14:3808–3816.
- Jaiswal R, Patel N, Kothari DC, Miotello A. Improved visible light photocatalytic activity of TiO2 co-doped with Vanadium and Nitrogen. Appl Catal B. 2012;126:47–54.
- Charanpaharia A, Umarea SS, Gokhaleb SP, Sudarsanc V, Sreedhard B, Sasikalac R. Enhanced photocatalytic activity of multi-doped TiO2 for the degradation of methyl orange. Appl Catal A. 2012;443–444:96–102.
- Kim CS, Shin JW, An SH, Jang HD, Kim TO. Photodegradation of volatile organic compounds using zirconium-doped TiO2/SiO2 visible light photocatalysts. Chem Eng J. 2012;204–206:40–47.
- Zhao N, Yao Y, Feng JJ, Yao MM, Li F. Enhanced photocatalytic activity of Co surface doped nanocrystalline TiO2-SiO2 composite films. Water Air Soil Pollut. 2012;223:5855–5864.
- Yao Y, Zhao N, Feng JJ, Yao MM, Li F. Photocatalytic activities of Ce or Codoped nanocrystalline TiO2–SiO2 composite films. Ceram Int. 2013;39:4735–4738.
- Guimarães JR, Farah CRT, Maniero MG, Fadini PS. Degradation of formaldehyde by advanced oxidation processes. J Environ Manage. 2012;107:96–101.
- Yan XD, Zou CW, Gao XD, Gao W. ZnO/TiO2 core–brush nanostructure: processing, microstructure and enhanced photocatalytic activity. J Mater Chem. 2012;22:5629–5640.
- Gao XT, Wachs IE. Titania–silica as catalysts: molecular structural characteristics and physico-chemical properties. Catal Today. 1999;51:233–254.
- Cani D, Pescarmona PP. Macroscopic TiO2–SiO2 porous beads: efficient photocatalysts with enhanced reusability for the degradation of pollutants. J Catal. 2014;311:404–411.
- Zhong JB, Li JZ, Feng FM, Lu Y, Zeng J, Hu W, Tang Z. Improved photocatalytic performance of SiO2–TiO2 prepared with the assistance of SDBS. J Mole Catal A 2012;357:101–105.
- Li F, Li H, Guan LX, Yao MM. Nanocrystalline Co2+/F− codoped TiO2-SiO2 composite films for environmental applications. Chem Eng J. 2014;252:1–10.
- Bettinelli M, Dallacasa V, Falcomer D, Fornasiero P, Gombac V, Montini T, Romano L, Speghini A. Photocatalytic activity of TiO2 doped with boron and vanadium. J Hazard Mater. 2007;146:529–534.
- Ren CJ, Qiu W, Chen YQ. Physicochemical properties and photocatalytic activity of the TiO2/SiO2 prepared by precipitation method. Separation Purif Technol. 2013;107:264–272.
- Zhou MH, Yu JG, Cheng B, Yu HG. Preparation and photocatalytic activity of Fe-doped mesoporous titanium dioxide nanocrystalline photocatalysts. Mater Chem Phys. 2005;93:159–163.
- Yin XL, Yao MM, Li F, Wu XF, Zhang SX. Microstructures and photo-catalytic properties of B3+ and F− co-doped TiO2 films. J Exp Nanosci. 2012;7:310–326.
- Li FT, Wang XJ, Zhao Y, Liu JX, Hao YJ, Liu RH, Zhao DS. Ionic-liquid-assisted synthesis of high-visible-light-activated N–B–F-tri-doped mesoporous TiO2 via a microwave route. Appl Catal B. 2014;144:442–453.