ABSTRACT
The tribological properties of lubricating oil containing micro/nano-scale WS2 (90 nm, 2 µm) and ionic liquid [C7H11F3N2O3S] are evaluated using a four-ball friction tester. Results show that the addition of micro/nano-scale WS2 can improve the tribological properties of the base oil; moreover, adding the ionic liquid as a solvent may lead to a better mixing of the micro/nano-scale WS2 and base oil and promote the dispersion of WS2 in the sample oil. The base oil with 90 nm nano-WS2 and ionic liquid presents the best anti-friction and anti-wear properties at 1 wt.% content. The surface analysis of wear scars reveals that ploughing is the main cause of wear in the three bottom balls. Furthermore, the wear furrows of nano-WS2 as lubricating additive are uniform and symmetrical and can homogenously appear on the friction area. This work proves that the micro/nano-scale WS2 plays an important role in improving the performance of tribological properties of lubricating oil.
1. Introduction
Nanotechnology is considered to be the most revolutionary and respected technology in the twenty-first century; it has a profound impact on other disciplines, industries and the society [Citation1]. With the continuous progress of science and technology, nanotechnology has been developed rapidly. At present, the tribological properties of lubricating oil that contains nanoparticles have become the topic of interest in the research field. Several studies have indicated that the addition of nanoparticles in lubricating oil can significantly reduce friction and wear [Citation2–6]. Friction-reduction and anti-wear behaviours are related to various factors, such as the type, size, shape and concentration of nanoparticles [Citation7].
Micro/nano-scale WS2 is a new type of lubrication and wear-reduction material and has excellent lubricating performance; it is one of the best materials for friction and lubrication recognised by the scientific community. The micro/nano-scale WS2 material is not only suitable for the usual lubrication conditions but can also be used in harsh working environments, such as high temperature, high pressure, high vacuum, high load, radiation and corrosive medium. Thus, this material is extensively applied in aviation, aerospace and other high-technology fields. The microstructure of WS2 crystal is a hexagonal layered hollow sphere, which surface forms a six-square network layer through S–W–S molecules. The layers are connected by van der Waals force, and the weak binding force is easily sheared between layers. Moreover, the slip is prone to occur between layers; thus, the friction coefficient is low. Therefore, in the process of lubrication, the WS2 particle is a system with stable performance and high quality on the microscopic level. Furthermore, the nano-scale WS2 has the characteristics of high density, small size, large specific surface area and high specific surface energy. In recent years, numerous researchers have studied the lubrication properties of nano-WS2. As a type of highly efficient green solvent, ionic liquids have been widely used in the fields of electrochemistry, catalysis and organic synthesis. Ionic liquids are non-flammable and non-explosive, and have a low melting point, low volatility and high thermal stability. These properties are consistent with the desired performance of an ideal lubricant. Due to the internal forces of the ionic liquid, such as electrostatic, hydrogen bonding and van der Waals, the ionic liquid can be relatively firmly adsorbed on most solid surfaces, thereby forming a lubricating boundary layer. In addition, the C7H11F3N2O3S selected in this experiment is an ionic liquid with surface activity. Its cations are imidazole, and the organic salt formed by imidazole generally has a large π bond and has high stability. The anions are fluorocarbon sulfonates, these fluorocarbon non-polar groups with fluorine atoms partially or completely replace the hydrogen atoms on the hydrocarbon chain; moreover, the fluorocarbon chain structure is far more stable than the hydrocarbon structure. With high chemical inertness and stability, its compatibility is good, which means that it can be compatible with other types of active substances and solutions. Thus, the ionic liquid is considered to be a promising new lubricant.
In this experiment, as a lubricating oil additive, the micro/nano-scale WS2 was selected as the research object to test its friction-reduction and anti-wear effects, and the function of ionic liquid as a solvent was also discussed. Micro/nano-scale WS2 and ionic liquid were added to a base oil to prepare a sample oil with different mass ratios. A series of experiments were conducted on four-ball friction tester to study the relationship among the three variables (i.e. WS2 concentration, ionic liquid and pure base oil). After the friction tests, worn areas were characterised by surface analysis through scanning electron microscopy (SEM). Finally, based on the experimental data, the correlation among these variables was analysed, and the influence of these variables on the tribological properties of lubricating oil was studied.
2. Experimental section
2.1. Materials
The micro/nano-scale WS2 used in the experiment was purchased from Sigma-Aldrich Corporation, and two sizes of WS2, 90 nm and 2 µm, were selected as the main object in this study. Ionic liquid is a type of salt compound that is mainly composed of organic cation and anion. This liquid has a strong ability to dissolve when used as a solvent and can dissolve organic matter, inorganic compounds, metal organic compounds and polymers [Citation8]. In this study, the ionic liquid of 1-ethyl-3-methylimidazolium trifluoromethansulfonate [C7H11F3N2O3S] was selected as a solvent to study its effects on nanoparticle aggregation. The base oil used was the full synthetic oil of Volkswagen oil 5W-30. Certain auxiliary materials were also used, such as anhydrous ethanol and petroleum ether.
2.2. Characterisation of WS2 particles
The two sizes of WS2 were characterised through SEM and transmission electron microscopy (TEM) to obtain their physical and chemical properties, such as surface morphology, particle size and chemical composition [Citation9]. The TEM characterisation was performed after ultrasonic dispersion in ethanol. The TEM micrographs of the nano-WS2 are shown in . (a) shows that the nano-WS2 particles have an average diameter of 90 nm and are ellipsoidal in shape. (b) shows that the nanoparticles are mostly clustered together and exhibits evident aggregation phenomenon. (a,b) shows the SEM micrographs of the 2-µm micro-WS2 powder. The powder is composed of a large number of lamellar WS2. The thickness of the micro-WS2 is approximately 2 µm, whereas their lengths vary from 5 to 20 µm ((a)). The powder also exhibits evident agglomeration phenomenon, as shown in (b).
2.3. Tribological tests
2.3.1. Samples
Two sizes of WS2 and ionic liquid were selected as lubrication additives in the base oil. The constituents of WS2 and ionic liquid as lubricant additives are shown in . The mass ratio of WS2/base oil in samples include 0.5, 1.0, 1.5 and 2.0 wt.%. Each sample was ultrasonically dispersed for 10 min. The samples with ionic liquid were obtained by adding ionic liquid and WS2 to the base oil based on a 1:1 ratio. In addition, a set of base oils without any additives was also prepared. Therefore, a total of 17 groups of sample oil were prepared.
Table 1. Contents of WS2 and ionic liquid as lubricant additives.
2.3.2. Tribological tests
The MRS-10(G) four-ball friction tester was employed to study the friction-reduction and anti-wear capabilities of the oil that contains additives. Each group of sample oil was ultrasonically dispersed for another 5 min before conducting the friction experiment. The friction and wear tests were conducted at a constant load of 392 N and at a rotating speed of 1450 rpm for 40 min at room temperature. A prolonged friction time results in a large wear scar diameter (WSD) and large contact areas between the top ball and the three bottom balls; thus, the shearing and friction forces increased between the steel balls. The steel balls (diameter = 12.7 mm, surface roughness Ra = 0.020 µm) used in the tests were fabricated according to the national standard of China (G10, GB/T308-2002 of China) from a quenched-and-tempered GCr15 bearing steel with a hardness of 61 HRC [Citation9]. The friction coefficient could be collected and transmitted to the computer through the sensor, thereby obtaining the friction coefficient of the figure. Wear rate was obtained by the average WSD of the three bottom balls. The average WSD of the three bottom balls was measured using an optical microscope to an accuracy of ±0.01 mm, and the averaged value was used to evaluate the wear properties. The morphology of the wear scar was examined using SEM.
3. Results and discussion
3.1. Results of tribological tests
(a–d) shows the variations of friction coefficient with the increase of time at the rotating speed of 1450 rmp and a constant load of 392 N. The friction coefficients of the sample oil with different contents of micro/nano-scale WS2 (2 µm, 90 nm) are shown in (a,b). (c,d) shows the friction coefficient of the sample oil containing ionic liquid and different contents of micro/nano-scale WS2 (2 µm, 90 nm).
Figure 3. Friction coefficient of the sample oil with different WS2 morphology, diverse concentrations of WS2 and ionic liquid: (a) 2 µm; (b) 90 nm; (c) 2 µm + ionic liquid; (d) 90 nm + ionic liquid.
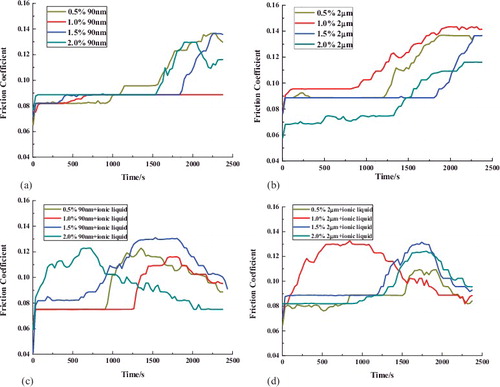
At the beginning of the test, the friction coefficient was approximately 0.0819 and could remain constant for approximately 1500 s ((a)). This result is significantly longer compared with that of pure base oil, as shown in . After more than 1500 s, the friction coefficient gradually increased, and the maximum friction coefficient could reach 0.1365. From the figure, the best lubrication state and the lowest friction coefficient occur when the added amount of nano-WS2 (90 nm) is 1%.
The friction coefficients of the sample oil that contains different contents of micro-WS2 (2 µm) and without ionic liquid are shown in (b), which presents a similar trend to that in (a). However, the two figures still slightly differ. First, the initial friction coefficient in (b) is slightly larger than that in (a). Second, the initial friction coefficient can maintain for 1200 s on average ((b)). Finally, the optimum amount of micro-WS2 in (b) is 2%.
(c) shows the friction coefficients of the sample oil that contains different contents of nano-WS2 (90nm) and ionic liquid. Moreover, the initial friction coefficient is approximately 0.0751 and can be kept for about 900 s. Furthermore, the friction coefficient begins to increase when the initial value reaches 0.1297; it begins to decrease gradually until it reaches the minimum friction coefficient. In addition, the lowest friction coefficient occurs in the sample with 1% nano-WS2.
(d) shows the friction coefficients of the sample oil with ionic liquid and different contents of micro-WS2 (2 µm). The initial friction coefficient can remain for 1300 s on average, which is longer than that in (b). The friction coefficient initially increases to the maximum value (0.1297) and then decreases and gradually reaches the minimum value, which is similar to the trend in (c). The optimum amount of micro-WS2 in (d) is 0.5%.
From the above analysis can be seen that the addition of 90nm WS2 of base oil, whether or not to add ionic liquid, the optimum amount is the nano-WS2 content of 1%. This indicates that the ionic liquid has little effect on the optimum content of nano-WS2. However, the optimum ratio of the addition of 2 µm WS2 and that of both adding ionic liquid and 2 µm WS2 are different, 0.5% and 2%, respectively. This indicates that the addition of ionic liquids affects the optimum amount of micron particles in the base oil. Thus, the nano-WS2 as an additive are affected by ionic liquids less than micro-WS2, and nano-WS2 are more stable in the oil.
The samples with the optimum amount of micro/nano-scale WS2 in (a–d) were selected and then were compared with the base oil without any additive. The results are shown in . All the friction coefficients increase with prolonged friction time; however, the friction coefficients of the samples that contain ionic liquid have a downward trend after reaching the maximum value. Moreover, the samples with lubricating additives (i.e. ionic liquid and WS2) can maintain a longer period of initial friction coefficient than that of the base oil. In addition, the samples with lubricating additives can evidently decrease the friction coefficient; thus, the WS2 as an additive plays an important role in friction-reduction and anti-wear effects.
A group of experiments with a duration of 5400 s was conducted to study whether the sample oil that contains ionic liquid and nano-WS2 additives can maintain excellent lubrication properties for a long time. The experimental results are shown in . Because it can be seen from , when the sample oils are added with WS2 and ionic liquid, their friction curves have a downward trend after a certain time. To demonstrate that the friction coefficient will not rebound after falling, it is possible to maintain a steady value for a long time, as shown in . The sample oils used in the experiments are base oil, and 1% 90 nm nano-WS2 and ionic liquid. When the lubrication state is stable, the friction coefficient of the sample oil that contains additives is lower than that of the base oil. In addition, the friction coefficient of the sample oil with ionic liquid does not rebound after the descent, and the friction coefficient almost decreases to the initial value.
3.2. Surface analysis of wear scars
shows the optical micrographs of the typical wear scars on the bottom ball. The steel balls were measured using the optical microscope ((a–e)); the measurements correspond to the steel balls of each curve in . shows the magnification of the optical micrographs of the wear scars.
Figure 6. Optical micrographs of the typical wear scars on the bottom balls lubricated by the sample oil: (a) base oil; (b) 1%, 90 nm WS2; (c) 1%, 90 nm WS2 and ionic liquid; (d) 0.5% 2 µm WS2 and ionic liquid; (e) 2% 2 µm WS2.
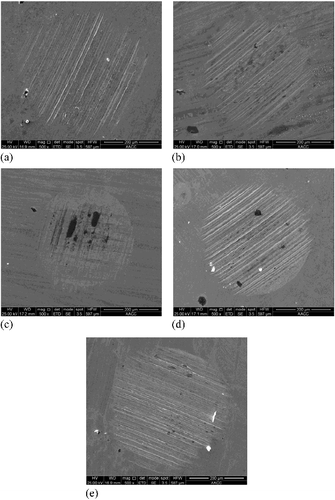
Figure 7. Magnification of the optical micrographs of the wear scars: (a) base oil; (b) 1%, 90 nm WS2; (c) 2% 2µm WS2.
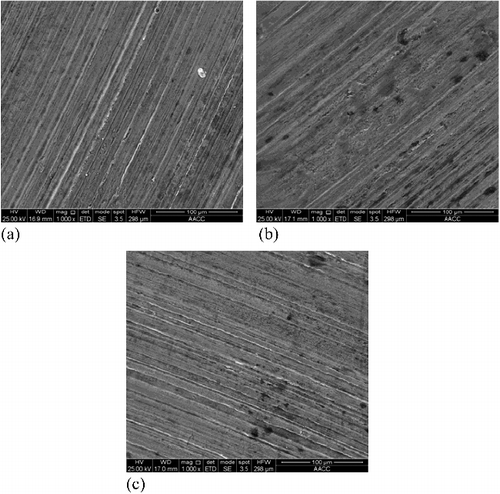
The ball surface exhibits evident furrows; thus, ploughing was the main manner of the wear on the three bottom balls. Compared with the base oil as lubricant, the wear furrows of micro/nano-scale WS2 as lubricating oil additive are more uniform and symmetrical and can homogenously appear on the entire friction area [Citation10]. lists the average values of WSD measured using the optical microscope. These values can be used to evaluate the wear properties. The WSD of the steel balls with pure base oil is larger than the diameter of the balls with WS2 and ionic liquid as additives. The WSD lessen ratio is also listed in . The WSD with additives decreases evidently, and the lessen ratios are approximately 5%–15%. This result indicates that the addition of nano-WS2 in the base oil affects the friction-reduction and anti-wear capabilities.
Table 2. Average WSD of steel balls with different lubricants.
3.3. Lubrication mechanism of WS2
The function of the lubricant is to form a lubricating film between the contact surfaces in a relative motion. This film can separate the surfaces from direct contact; thus, dry friction is changed into the internal friction between the lubricant molecules, which decreases friction and wear. In general, the lubricant can reduce the friction, wear and adhesion between the friction pairs in relative motion. Most of the lubricating oil is expelled from the gap between the contact surfaces under high load. Of course, there is also a part of the lubricating oil adsorbed on the solid surfaces, which isolate the direct contact of the moving surfaces and form a boundary lubrication layer between the surfaces. However, the adsorption of common oil is very weak, so it is difficult to maintain an effective boundary lubrication film for a long time. Therefore, it is necessary to use some auxiliary additives to enhance the adsorption of oil, thereby helping to form a solid boundary lubrication layer on the surface of the lubricant.
The friction coefficient curve in shows that the friction coefficient initially remains constant, increases gradually, and finally tends to stabilise. At the beginning of the experiments, the friction pairs were completely separated by a thick continuous lubricating film. The motion of the friction pairs drives the internal friction between the lubricating film molecules; therefore, the friction force and friction coefficient are considerably small, as in the beginning of most curves in . Shortly afterwards, as the oil cannot be firmly adsorbed on the solid surface under heavy load, the thickness of the lubricating film becomes thinner, and forming a complete oil layer is difficult. The thickness of the film is smaller than the height of the micro-convex body on the friction surface; thus, some of the micro-convex body contact directly. Meanwhile, the friction enters the running-in period, and the abrasive grains produced during the rubbing process are mixed in the lubricating oil, which result in the increase of friction coefficient. Finally, the friction coefficient tends to stabilise at the end of the running-in period, which may be due to the formation of a physical or chemical tribofilm on the surface.
The mechanisms of friction and wear for micro/nano-scale WS2 can be explained by several aspects. The dispersed WS2 in the lubricating oil has high surface energy. At the initial stage of friction, the WS2 and lubricating oil are adsorbed on the solid surfaces to form a layer of physical lubricating film. The film not only bears the load of the steel ball but also separates the friction surfaces and prevents them from direct contact [Citation9]. The addition of WS2 helps to enhance the adsorption of base oil, so that the physical adsorption film can be more reliable. In the friction process, especially under high load and high local temperature conditions, the nanoparticles with high dispersal and self-diffusion capability easily form a permeable layer on the surface of the metal friction pairs or react with the metal elements on the friction surface to produce a strong and wear-resistant chemical reaction film, thereby reducing the friction and wear between the friction pairs; moreover, the chemical reaction film is more stable than the adsorption film [Citation11]. The ellipsoidal nanoparticles in the lubricating oil enter and roll between the contact surfaces, which can be considered as nano-ball bearings. In addition, ultrafine WS2 powder can fill the micro-pits and damaged parts of the friction surface, and even into the matrix to play a role in repairing; thus, the friction table can remain in a relatively flat state.
As a solvent, the ionic liquid may lead to a better combination between WS2 and the base oil. First, adding ionic liquid to the sample oil progressively weakens the reunion between the nanoparticles as the attractive van der Waals forces are overcame by the adsorption of the ionic liquid [Citation12]. The ionic liquid promotes the dispersion of the WS2 in the sample oil, which makes the WS2 play a better role in friction-reduction and anti-wear effects. Second, C7H11F3N2O3S is an ionic liquid with surface activity, which can significantly reduce the surface tension of oil and promote the better integration of oil, ionic liquids and WS2. In the working process of the actual friction pair, the protective layer on the surface of the friction pair will be worn out and reform, which is a dynamic process. When the additive concentration is suitable, the wear rate is close to the film forming rate, which can reach the dynamic balance and the best lubricating effect.
4. Conclusion
(1) | The tribological properties of lubricating oil are influenced by the morphology and content of WS2 additives. The results indicate that the proper content of 90 nm WS2 in base oil is 1.0 wt.%, whereas that of 2 µm WS2 in the same medium is 2.0%; the proper content of 90 nm WS2 and ionic liquid in base oil is also 1.0%, whereas that of 2 µm WS2 and ionic liquid in the same medium is 0.5%. Moreover, the nano-WS2 as an additive are affected by ionic liquids less than micro-WS2, and nano-WS2 are more stable in the oil. Therefore, the tribological properties of lubricating oil can be affected by different WS2 particle sizes and concentration of additives. Generally, the lowest friction coefficient occurs in the sample with 1%, 90 nm WS2 and ionic liquid. | ||||
(2) | Compared with the base oil without adding any additives, the sample oil with micro/nano-scale WS2 can evidently reduce the friction coefficient. The initial friction coefficient of the oil with the addition of WS2 can generally be maintained for a longer time. In addition, the friction coefficients of the samples that contain ionic liquid have a downward trend after reaching their maximum values. Micro/nano-scale WS2 and ionic liquids play an outstanding role in base oil due to the excellent anti-wear and anti-friction properties of WS2 and the stability and surface activity of ionic liquids. Therefore, micro/nano-scale WS2 and ionic liquid indeed have a significant effect on friction-reduction and anti-wear capabilities. | ||||
(3) | The analysis of the optical micrographs of wear scars on the bottom ball reveals that ploughing is the main manner of wear in the three bottom balls, and the wear furrows of WS2 as lubricating oil additive are more uniform and symmetrical and can homogenously appear on the entire friction area. Moreover, WS2 and ionic liquid function as lubrication additives in base oil. The WSD of the steel balls with WS2 and ionic liquid as lubrication additives is smaller than that of the steel balls with base oil without adding any additive. |
Acknowledgments
This work is supported by the National Natural Science Foundation of China (No. 51575525), and a project funded by the Priority Academic Program Development of Jiangsu Higher Education Institutions (PAPD).
Disclosure statement
No potential conflict of interest was reported by the authors.
References
- Wu YY, Tsui WC, Liu TC. Experimental analysis of tribological properties of lubricating oils with nanoparticle additives. Wear. 2007;262:819–825.
- Rosentsveig R, Gorodnev A, Feuerstein N, et al. Fullerene-like MoS2 nanoparticles and their tribological behavior. Tribol Lett. 2009;36:175–182.
- Kalin M, Kogovsek J, Remskar M. Mechanisms and improvements in the friction and wear behavior using MoS2 nanotubes as potential oil additives. Wear. 2012;280–281:36–45.
- Watanabe S, Noshiro J, Miyake S. Tribological characteristics of WS2/MoS2 solid lubricating multilayer films. Surf Coat Technol. 2004;183:347–351.
- Zin V, Agresti F, Barison S, et al. Tribological properties of engine oil with carbon nano-horns as nano-additives. Tribol Lett. 2014;55:45–53.
- Tannous J, Dassenoy F, Vacher B, et al. Understanding the tribochemical mechanisms of IF-MoS2 nanoparticles under boundary lubrication. Tribol Lett. 2011;41:55–64.
- Tannous J, Dassenoy F, Tremel W, et al. Synthesis and tribological performance of novel MoxW1-xS2(0≤x≤1) inorganic fullerenes. Tribol Lett. 2010;37:83–92.
- Han JY, Huang X, Wang H, et al. Progress in characteristic and application of ionic liquid as green solvent. Chem Ind Eng. 2005;22(1):62–66.
- Huang HD, Tu JP, Zou TZ, et al. Friction and wear properties of IF-MoS2 as additive in paraffin oil. Tribol Lett. 2005;20(3):247–250.
- Hu KH, Hu XG, Xu YF, et al. The effect of morphology on the tribological properties of MoS2 in liquid paraffin. Tribol Lett. 2010;40:155–165.
- Wu J. Preparation, charactetization and application of MoS2/WS2 nanomaterials [master's thesis]. Shangdong: Qingdao University of Science & Technology; 2007.
- Cooper PK, Li H, Rutland MW, et al. Tribotronic control of friction in oil-based lubricants with ionic liquid additives. R Soc Chem. 2016;18:23657.