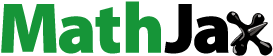
Abstract
The objective of the current research was to study the effects of polyvinyl alcohol (PVA) electrospun nanofibers loaded with different concentrations of leaf extract of Moringa oleifera (MO) on butyrylcholinesterase (BuChE), monoamine oxidase A (MAO A) and monoamine oxidase B (MAO B) enzymes. The MO-loaded PVA nanofibers were investigated for their inhibitory effect on BuChE and MAO A & B enzymes. The characterization of the fabricated nanofibers was performed by using SEM, FTIR and XRD. The results showed that increasing the concentration of MO, the enzyme inhibition capability of electrospun nanofibers was greatly (p < 0.05) influenced. This study involved the fabrication of electrospun nanofibers of PVA containing four different concentrations of MO (0%, 0.1%, 0.2% and 0.4%). The SEM micrographs revealed that all the nanofibers were porous, beadless and smooth. At a high concentration of M. oleifera, uniform fibers were obtained. The FTIR results of the fabricated nanofibers revealed that chemical interaction did not take place between M. oleifera and polyvinyl alcohol. The results reveal that with an increase in the concentration of M. oleifera (MO), the enzymes inhibition capability of electrospun nanofibers is greatly influenced. Different concentrations of MO extract were successfully loaded to the MMT-reinforced, glutaraldehyde-cross-linked nanofibers.
1. Introduction
During the past few years, the fabrication of polymeric nanofibers for drug delivery has extensively increased due to their variety of applications, such as to achieve the controlled and targeted delivery of drugs, minimize their undesired effects, and reduce the dose size [Citation1]. Similarly, herbal extracts could be loaded into polymeric nanofibers to improve their therapeutic efficacy [Citation2]. The herbal extract can be blended with polymer to produce a composite. This green synthesis of polymer matrix composite or fibers has been explored because such materials are environmentally friendly. Medicinal plants possess various bioactive components that act as inhibitory agents. For instance, turmeric and cinnamon are extensively used for medicinal treatment. Herbal therapies show a dominant role in the healthcare system. In developing countries, the use of herbal medicines has a long history [Citation3]. World Health Organization (WHO) promotes the addition of herbal drugs in national health care programs due to their accessibility at low prices. In addition, the use of herbal drugs is safer as compared to synthetic drugs [Citation3].
Moringa oleifera (MO), commonly known as Drumstick tree or Sohanjana, is a rapidly growing, transitive plant that is 10–12 m high [Citation4]. M. oleifera belongs to the Moringaceae family. It is cultivated all over the tropical areas and is found in Bangladesh, India, Afghanistan and Pakistan [Citation4]. The leaves of the plant have widely been used because they are rich in bioactive components such as vitamins, amino acids carotenoids, polyphenols, phenolic acids, flavonoids, alkaloids, isothiocyanates and tannins [Citation4].
Enzymes are biologically active substances or proteins that catalyze various metabolic reactions in the human body. They possess active sites on which substrate can attach. Many metabolic imbalances are observed due to the activity of enzymes, so enzymes inhibition is required. Inhibitors are chemical compounds that can bind on active sites and stop the binding of the substrate with enzyme/s active site and cause hindrance for enzymes to catalyze the chemical reaction. High or low levels of butyrylcholinesterase (BuChE), monoamine oxidase A (MAO A) and monoamine oxidase B (MAO B) enzymes have been involved in many neuropsychiatric disorders, such as Parkinson’s disease, Alzheimer’s disease and depression [Citation2]. These enzymes are important binding proteases or flavin (FAD) present in the outer mitochondrial membrane. MAO are responsible for the oxidation of monoamines such as dopamine, adrenaline, norepinephrine, serotonin. BuChE cause loss of cholinergic neurons by degradation of acetylcholine into acetate and thiocholine [Citation3]. This degradation of neurotransmitters leads to neurological disorders like depression and Alzheimer. Therefore, there is a need to inhibit these enzymes by using alternative inhibitors to reduce neuropsychiatric disorders. Medicinal plants are an important source for the treatment of neurological disorders. Bioactive components like flavonoids and alkaloids present in plants have good potential to inhibit these enzymes. Alam et al. investigated the natural enzymes inhibators with antidiabetic property. They studied the enzymes responsible for diabetic disease and find the natural source to inhibit these enzymes as antidiabetic drug [Citation5]. Fais et al. examined the enzyme inhibitory potential of Euphorbia characias extract towards α-amylase and α-glucosidase responsible for metabolic disorders [Citation6]. A lot of work has been performed on the enzyme inhibitory effect of various plant extracts for pharmaceutical applications. However, no study has yet described the enzyme inhibition behavior of M. oleifera nanofibers.
Polymeric nanofibrous mats are an important class of nanomaterials and have wide applications in the nanotechnology field. Nanofibers have a diameter in the range of nanometer (less than 100 nm). They possess various physical properties and a wide range of applications [Citation7]. Many natural and synthetic polymers like cellulose, chitosan, alginate, keratin and polycaprolactone, polyurethane and polylactic acid respectively are used to fabricate nanofibers [Citation8]. The diameter can be varied by changing the method of production and type of polymers. Fabricated nanofibers have unique properties like high surface to volume ratio, high porosity and good mechanical properties [Citation9, Citation10].
In contrast to other fibers fabrication techniques, the electrospinning technique is the most applicable, easy processing, cost-effective and extensively used technique for the fabrication of fibers [Citation11–13] or membranes with fiber diameter in the range of micro to nanometer scale. A high voltage is used to fabricate nanofibers with a high surface to volume ratio and reduced pore size in the electrospinning technique. Through electric sources, negative and positive polarity is created [Citation14]. Fibers can be fabricated by using polymer solution and melt of polymer in electrospinning technique.
The fabrication of electrospun scaffolds is becoming important in the biomedical field as in tissue engineering, wound dressings, and delivery of the drug. The fabrication of PVA fibers has attracted widespread interest due to its nontoxic nature, water-solubility, biodegradability, chemical resistance, biocompatibility, bio-adhesive, elastic and non-carcinogenic nature [Citation15–17]. To improve water stability of electrospun PVA fibers, crosslinking is done by using various crosslinkers like glutaraldehyde, citric acid, malic anhydride, glyoxal, formaldehyde and bis(β-hydroxyethyl) sulfone [Citation18].
The aim of this research work was to study the enzymes inhibition potential of the PVA-MO leaf extract-based electrospun nanofibers.
2. Experimental section
2.1. Materials
Polyvinyl alcohol (PVA, MW: 72,000 g/mol, degree of hydrolysis 85.89%) and glutaraldehyde (as 50% PS solution), and MMT (K-catalyst, pH 3-4, corporation with surface area of 250 m2/g) were obtained from Sigma-Aldrich. Deionized water was obtained from commercial sources.
2.2. Preparing the leaf extract
M. oleifera (MO) plant leaves were acquired from Benessere Health Company, Multan, Pakistan, and authenticated by an expert botanist Dr. Altaf Hussain Dasti from the Department of Botany, Bahauddin Zakariya University Multan, Pakistan. The voucher specimen was submitted to the university herbarium (Voucher No. 78X/20) for further studies.
The fresh plant leaves (500 g) were washed to remove dust particles, dried for about a month in a shaded area at room temperature. The dried leaves were ground into fine powder by using an electrical grinder. Extract of MO leaves was obtained by using the maceration process. The powdered leaves were macerated or soaked into methanol for about 48 days. Stirring with a glass rod and shaking was done at least 4–5 times daily. After filtration, the obtained filtrate was evaporated, dried and stored in a dry place. The filtrate or crude extract was dried and the solvent was evaporated under reduced pressure at 40 °C in a rotary evaporator. Finally, the semi-solid crude extract was obtained.
2.3. Phytochemical screening of extract
Various qualitative (for instance, Mayer’s test, Hager’s tests and Shinodha test) and quantitative tests were performed for the screening of MO extract. Quantitative characterization was performed by using high-performance liquid chromatography (HPLC, Shimadzu, Japan), connected with a UV detector set at 280 nm. The mobile phase comprised water:acetic acid (94:6) having pH 2.27. After running this mobile phase, 15% acetonitrile solution was run for 15 min, then 45% acetonitrile solution was run for 30 min, and 100% acetonitrile solution was run for 45 min. C18 column (25 cm × 4.6 mm; 5 µ) (Shim-Pack, USA) was used for ingredient isolation. Finally, peaks in chromatograms were compared with standards.
2.4. Solution preparation for electrospinning
The aqueous solution of PVA (16% w/w) was prepared in deionized water and put on a hot plate for 4–5 h at 80 °C. Stirring was done with the help of a magnetic stirrer at a rotation speed of 250–300 rpm to get a homogenous solution. Then 1–2 drops of glutaraldehyde were added with continuous stirring to complete the crosslinking reaction between PVA and glutaraldehyde. A 1% MMT was added while maintaining the temperature at 40 °C. The prepared nanocomposite solution was cooled down to room temperature and leaf extract was added into the polymeric mixture. The resultant solution was ready to electrospun. Four different formulations were prepared by changing the concentrations of plant extract. Each product was labeled as PNF 1-4, where PNF stands for polymeric nanofibers and 1-4 are nanofibers with 0, 0.1, 0.2 and 0.4 g concentrations of MO extract (). The quantities of PVA, glutaraldehyde and MMT nanoclay were kept constant.
Table 1. Composition of various formulations containing M. oleifera extract.
2.5. Electrospinning of polymeric solution
The electrospinning machine of model FLUIDNA TEK LE-10 (BIOINICIA, Spain) was used for the fabrication of nanofibers. The main components of the electrospinning machine are syringe and needle, syringe pump, high voltage power supply and the collector plate. The parameters are set at optimum conditions as a voltage of about 15 kV was applied and the metallic collector plate was set at a distance of 18 cm. The electrospinning process was done at a 0.5 ml/h feed rate.
The completely dissolved homogenous solution was subjected to the electrospinning process by using an electrospinning machine. A syringe of 5 ml with a needle having a diameter of 11.99 mm was filled with polymeric solution. The process parameters to fabricate nanofibers were adjusted at optimum value and kept constant. When a high voltage of about 15 kV was applied, charged solution jet was ejected from the spinneret in the form of a Tylor cone. The charged solution jet was moved towards the collector and deposited on it. The metallic collector plate was set at a distance of 18 cm from the needle tip. The electrospinning process was done at a 0.5 ml/h feed rate at room temperature. The collector plate was covered with aluminum foil from which solid fibers were collected as a membrane () and stored.
2.6. Enzyme inhibition studies
2.6.1. MOA A and B inhibition studies
The leaf extract of MO was analyzed on monoamine oxidase A and B isozymes (MAO A and MAO B) through the Fluorescence method. Fresh enzymes were prepared 15–20 min before, at cool room temperature. The activity of MAO A and MAO B was blocked irreversibly by using Clorgyline (60 nM) or Deprenyl (300 nM). A 96-well plate was used to perform this assay. The assay volume was 200 μL having 140 μL buffer, 10 μL test sample (1 mg/mL, 100% DMSO) followed by adding enzyme 10 μL (26 μg of protein for MAO A and 5.0 μg for MAO B). The mixture was incubated for 15 min for MAO B and 20 min for MAO A, then 20 μL of freshly prepared Amplex red and 20 μL of the substrate was added to this mixture. A 0.1 mM concentration of Clorgyline and Deprenyl was used to determine the activity of non-MAO A and MAO B. Fluorescence plate reader (BMG Labtech GmbH, orten berg Germany) was used to determine the change in the fluorescence. All experiments were repeated in triplicate, and percentage inhibition was calculated.
where, Df is the After read minus pre read, NC is the difference in Negative control (DMSO) and MAO is the Monoamine oxidase.
2.6.2. Cholinesterase inhibition studies
Ellman's test method was employed for the evaluation of the potency of samples (extracts) for the inhibition of BuChE (Butyrylcholine esterase). The basis of the spectrophotometric method is the reaction of released thiocholine to provide a colored product with chromogenic reagent 5,5-dithio-bis (2-nitrobenzoic) acid (DTNB) [Citation19]. A 2.5 units/mL of concentrated enzyme solutions were prepared. The volume of the assay was 100 μL containing 10 μL of test compound (10% DMSO, 1 mg/mL concentrated) and 60 μL buffer followed with the addition of 10 μL (0.04 U/well) enzyme. The incubation of mixture was done for about 10 min. Then 10 μL of the substrate (0.5 mM) and 10 μL of DTNB (0.5 mM) was inserted into this mixture. The yellow anion production was noted at 405 nm after 20 min. The following equation was employed to calculate the rate of percentage inhibition:
2.7. Characterizations of nanofibers
2.7.1. Scanning electron microscopy (SEM)
Jeol, JSM 6400 F was used to identify the surface morphology of nanofibers. In SEM microscopy a focused beam of electrons having a high level of energy is used and generates different signals at the surface of the solid sample. These signals give information about the sample, topology, external morphology and diameter of nanofibers. It works at a high range of temperature and pressure and has a resolution of less than 1 nm.
2.7.2. Fourier transformed infrared spectroscopy (FTIR)
The FTIR spectroscopy analysis was made by using ‘FTIR-6600 type A’ Fourier Transform Spectrophotometer. The spectrum was scanned from 4700 cm−1 to 500 cm−1. It is studied to deal with both qualitative and quantitative analysis of organic as well as inorganic materials. This spectroscopic method uses infrared light of electromagnetic radiations for scanning materials and produced IR spectrum reveals their chemical properties. This technique can be used for the identification of unknown compounds, additives within polymer and more.
2.7.3. Thermogravimetric analysis (TGA)
Q600 SDT thermogravimetric analyzer was used to study the thermal stability of fabricated nanofibers. The thermal decomposition of samples was carried out in the range of 20–700 °C with a heating rate of 10 °C per minute. In TGA analysis sample mass loss is observed as a function of time or temperature when the controlled temperature is supplied to the sample in an inert atmosphere.
2.7.4. X-Ray powdered diffraction (XRD)
XRD is an analytical technique used to identify the physical form, amorphous or crystallinity of materials. The crystal structure and atomic spacing can be studied by using this technique. X-rays are waves of electromagnetic radiation. When incident X-rays come into interaction with atoms electrons, the crystal atoms scattered these rays. These rays undergo destructive and constructive interference and this process is known as the diffraction process. Philips XPERT PRO 3040/60 was used to identify the physical nature of nanofibers. The scanning scope of 2θ was 5–80°.
2.7.5. Contact angle analyzer
The wettability of fabricated nanofibers was examined through a contact angle analyzer (Water Contact angle Goniometer). In this analysis drop images are captured and the drop shape was analyzed as a function of time automatically. Surface tension and interfacial tension can be directly measured through a contact angle meter.
2.7.6. Fluorescence plate reader
A fluorescence plate reader (BMG Labtech GmbH, Germany) was employed to quantify the absorbance of light emitted by a fluorescent sample. This method was employed for the detection of many chemical and biological processes.
2.8. Statistical analysis
Various findings were compared using a t-test at a level of significance of 0.05 with the help of SPSS version 17.0.
3. Results and discussion
3.1. Enzymes inhibition studies
The enzyme inhibitory potential of leaf extract of MO and fabricated nanofibers with different concentrations of leaf extract were evaluated against MAO A, MAO B and BuChE as shown in . The results show that the inhibition potential of PNF against all enzymes significantly (p < 0.05) increases with an increase in leaf extract concentration. The results show that the inhibition potential of PNF against all enzymes significantly (p < 0.05) increases with increase in leaf extract concentration as shown in . The bioactive components present in leaf extract of MO are responsible for the inhibition of these enzymes [Citation20, Citation21]. PNF-4 fraction has shown the highest enzyme inhibition among other fractions (p < 0.05). M. oleifera is a natural plant that contains phenolic compounds, alkaloids and flavonoids which are responsible for the inhibition of enzymes. Flavonoid compounds have antidepressant effects due to their neuroprotective property. Flavonoids such as apigenin, quercetin, luteolin, kaempferol, naringenin and galangin can inhibit MAO enzymes [Citation22]. Flavonoids like quercetin have the potential to inhibit monoamine oxidases by intermolecular hydrogen bonding and π–π stacking interactions. The double bond at 2 and 3 positions of C ring, substituent in the B ring, the methoxyl group and hydroxyl group in B ring at the para position in flavonoids are responsible for Monoamine oxidase inhibition [Citation23].
3.2. Phytochemical screening of extract
The qualitative tests confirmed the presence of alkaloids, saponins, glycosides, fixed oils, phytosterols and flavonoids. In addition, quantitative analysis confirmed the presence of quercetin, gallic acid, caffeic acid, vanillic acid, benzoic acid and coumaric acid ().
3.3. Scanning electron micrographs
Surface Morphology of nanofibers loaded with leaf extract of MO and without extract are studied to investigate the changes in surface properties of fabricated nanofibers. SEM micrographs of nanofibers with MO leaf extract are shown in . and e illustrate the SEM images of crosslinked PVA containing 0.2 g and 0.4 g of leaf extract of MO. The SEM micrographs reveal that the fabricated nanofibers have a uniform, smooth and beadless surfaces as shown in . The fibers are randomly oriented and possess a high degree of porosity. The average diameter of nanofibers without extract is approximately 300–330 nm. The results observed from micrographs show that the morphology of fabricated nanofibers is slightly changed by increasing the concentration of leaf extract. The nanofibers with a 0.4 g concentration of extract have a uniform, randomly oriented, beadless and smooth surface. But the diameter of nanofibers is slightly increased with an increase in extract concentration due to an increase in viscosity of solution [Citation20]. The increase in viscosity reduces the mobilization of extract and leads to thicker nanofibers. Beaded nanofibers are obtained at a high concentration of leaf extract. The PVA nanofibers with 0.2 g and 0.4 g of leaf extract show the diameter in the range of 350–370 nm and 390–410 nm.
3.4. Fourier transform infrared spectroscopy
illustrates the FT-IR results of MMT reinforced crosslinked PVA nanofibers. Black peaks represent the sample PNF-1 in which the concentration of leaf extract is 0%. In , FT-IR spectra show that PVA have various absorbance peaks at different frequencies and similar peaks are observed for nanofibers containing leaf extract. The peaks for sample PNF-4 shows some degree of broadness due to the presence of extract. The spectra show the O-H stretching at 3313 cm−1, C = O at 1733 cm−1, O–H bending at 1432 cm−1, 1365 cm−1 and 1320 cm−1, the stretching C-H at 2930 cm−1, and the bending C–H at 941 cm−1, 835 cm−1, and 610 cm−1 and C-O stretching at 1254 cm−1 and 1088 cm−1. There is no appearance of a new peak in the spectra of PNF containing leaf extract which shows the interaction takes place between PVA and extract [Citation21]. In FT-IR spectra of nanofibers loaded with root extract, the broadening of peaks was observed due to the hydrogen bond between PVA and compounds present in Moring oleifera, indicating that hydroxyl and carboxylic acid groups are present in the extract.
3.5. Thermogravimetric analysis
TGA analysis was done to determine the thermal stability and weight loss pattern of fabricated nanofibers as a function of temperature. illustrates the thermogram representation of MMT reinforced PVA nanofibers in the absence of leaf extract and nanofibers with 0.2 g and 0.4 g of leaf extract. showed that three typical weight loss regions can be observed in the TGA thermogram. The initial weight loss in the range of 40–70 °C is appeared due to the loss of moisture present in nanofibers. The mass loss from 280 to 390 °C is due to the degradation of the carbon chain present in PVA and the loss of hydroxyl groups. The weight loss from 400 to 470 °C is due to the decomposition of -OH groups present in MMT and some residue [Citation24]. Some mass loss between 120 and 268 °C is due to the decomposition of volatile components present in the leaf extract. PNF-4 shows the thermal behavior of nanofibers containing a high concentration of leaf extract. The mass loss between 110 and 260 °C is greater as compared with the PNF-3 sample which is due to the presence of a large number of volatile components. The results show that the thermal stability of fabricated nanofibers decreases to some extent due to the incorporation of volatile components present in leaf extract of MO.
3.6. X-Ray diffraction spectroscopy
explains the X-ray diffraction spectra of nanofibers without leaf extract, 0.2 g MO/PVA, and 0.4 G MO/PVA electrospun nanofibers. illustrates that the fabricated nanofibers consist of four different peaks. The pure PVA nanofibers showed two characteristic peaks at 20.4° and 40° [Citation25–26]. The peak of PVA is slightly shifted to 2θ = 19.54° in electrospun nanofibers containing leaf extract of MO. The X-ray diffractogram of fabricated nanofibers containing different concentrations shows that relatively broad peaks are appeared as compared to PNF-1 which demonstrates an increase in the amorphous region. In PNF-4 the peak at 2θ = 20.50° is slightly disappeared, showing less crystalline behavior of fabricated nanofibers. The interaction between PVA and leaf extract of M. oleifera results in the disappearance of some peaks [Citation27]. The diffractogram shows a peak for MMT at 2θ = 12.85°. However, the loading of leaf extract did not cause a significant effect on the amorphous behavior of fabricated nanofibers [Citation28].
3.7. Contact angle measurements
The wettability of MMT reinforced crosslinked PVA nanofibers is investigated through a contact angle analyzer. The wetting ability of samples PNF-1 with 0% of leaf extract and PNF-4 containing 12% of extract is examined by using the drop method. shows contact angle images of fabricated nanofibers at different time intervals. The materials with less value of angle θ (<90 °C) have a high degree of hydrophilicity [Citation29]. shows less contact angle (40 °C) with a high degree of hydrophilicity. shows that the value of the angle is increased to 50 °C due to interaction between hydroxyl group present in PVA chain and extract [Citation9]. According to the literature, hydrophilicity is increased by increasing the concentration of plant extract [Citation10]. But in our research work, it is not justified. This may be due to the presence of some hydrophobic tails in bioactive components or the interaction of free hydroxyl groups present in PVA with extract compounds [Citation29].
5. Conclusion
In this study, MMT reinforced crosslinked PVA nanofibers have been fabricated with successful loading of different concentrations of leaf extract of M. oleifera by using an electrospinning machine. SEM images indicated the porous, smooth and uniform morphology with no presence of beads. According to findings, the bioactive components present in M. oleifera tend to inhibit enzymes involved in neurological disorders. The extract loaded nanofibers showed a promising enzyme inhibition potential. These nanofibers could be further tested in preclinical studies to test their therapeutic and protective potential against Alzheimer’s and Parkinson’s disease.
Notes on contributors
•••
Disclosure statement
No potential conflict of interest was reported by the authors.
References
- Pourabadeh A, Mirjalili M, Shahvazian M. Modification of silk fibroin nanofibers scaffold by PAMAM dendrimer for cell culture. J Exp Nanosci. 2020;15(1):297–306.
- Huang Y, Zhu C, Xie R, et al. Green synthesis of nickel nanoparticles using Fumaria officinalis as a novel chemotherapeutic drug for the treatment of ovarian cancer. J Exp Nanosci. 2021;16(1):369–382.
- Abbas S, Chang D, Riaz N, et al. In-vitro stress stability, digestibility and bioaccessibility of curcumin-loaded polymeric nanocapsules. J Exp Nanosci. 2021;16(1):230–246.
- Vergara-Jimenez M, Almatrafi MM, Fernandez ML. Bioactive components in Moringa oleifera leaves protect against chronic disease. Antioxidants. 2017;6(4):91.
- Alam F, Shafique Z, Amjad ST, et al. Enzymes inhibitors from natural sources with antidiabetic activity: a review. Phytother Res. 2019;33(1):41–54.
- Fais A, Era B, Di Petrillo A, et al. 2018). Selected enzyme inhibitory effects of euphorbia characias extracts. Biomed Res Int. 2018;2018:1219367.
- Sofi HS, Rashid R, Amna T, et al. Recent advances in formulating electrospun nanofiber membranes: Delivering active phytoconstituents. J Drug Delivery Sci Technol. 2020;60:102038. Volume
- Amna R, Ali K, Malik MI, et al. A brief review of electrospinning of polymer nanofibers: history and main applications: a brief review of electrospinning of polymer nanofibers: history and main applications. 2020;23(3):151–163.
- Sagadevan S, Vennila S, Muthukrishnan L, et al. Exploring the therapeutic potentials of phyto-mediated silver nanoparticles formed via Calotropis procera (ait.) R. Br. root extract. J Exp Nanosci. 2020;15(1):217–231.
- Gugulothu D, Barhoum A, Nerella R, et al. Fabrication of nanofibers: electrospinning and non-electrospinning techniques. Barhoum A, Bechelany M, Makhlouf ASH, editors, Handbook of nanofibers. 2019. p. 45–77. Switzerland: Springer Nature.
- Zhang C, Li Y, Wang P, et al. Electrospinning of nanofibers: Potentials and perspectives for active food packaging. Compr Rev Food Sci Food Saf . 2020;19(2):479–502.
- Begum HA, Khan K. Study on the various types of needle based and needleless electrospinning system for nanofiber production. Int J Text Sci. 2017;6:110–117.
- Santhoshkumar R, Hima Parvathy A, Soniya EV. Phytosynthesis of silver nanoparticles from aqueous leaf extracts of Piper colubrinum: characterisation and catalytic activity. J Exp Nanosci. 2021;16(1):295–309.
- Chen Y-P, Liu H-Y, Liu Y-W, et al. Determination of electrospinning parameters’ strength in poly (D, L)-lactide-co-glycolide micro/nanofiber diameter tailoring. J Nanomater . 2019;2019:1–8.
- Nisar B, Sultan A, Rubab S. Comparison of medicinally important natural products versus synthetic drugs-a short commentary. Nat Prod Chem Res. 2018;6:308.
- Dar RA, Shahnawaz M, Qazi PH, General overview of medicinal plants: a review. J Phytopharmacol. 2017;6(6):349–351.
- Fonmboh DJ, Abah ER, Fokunang TE, et al. An overview of methods of extraction, isolation and characterization of natural medicinal plant products in improved traditional medicine research. AJRIMPS. 2020;9(2):31–57.
- Ardekani NT, Khorram M, Zomorodian K, et al. Evaluation of electrospun poly (vinyl alcohol)-based nanofiber mats incorporated with Zataria multiflora essential oil as potential wound dressing. Int J Biol Macromol. 2019;125:743–750.
- Mumtaz A, Majeed A, Zaib S, et al. Investigation of potent inhibitors of cholinesterase based on thiourea and pyrazoline derivatives: synthesis, inhibition assay and molecular modeling studies. Bioorg Chem. 2019;90:103036.
- Fayemi OE, Ekennia AC, Katata-Seru L, et al. Antimicrobial and wound healing properties of polyacrylonitrile-moringa extract nanofibers. ACS Omega. 2018;3(5):4791–4797.
- Hikmawati D, Rohmadanik A, Putra A. In the effect of aloe vera extract variation in electrospun polyvinyl alcohol (PVA)-aloe vera-based nanofiber membrane. J Phys Conf Series IOP Publ. 2018;012096.
- Larit F, Elokely KM, Chaurasiya ND, et al. Inhibition of human monoamine oxidase a and B by flavonoids isolated from two Algerian medicinal plants. Phytomedicine. 2018;40:27–36.
- Mathew B, Suresh J, Mathew E, et al. Plant secondary metabolites-potent inhibitors of monoamine oxidase isoforms. CNSAMC. 2014;14(1):28–33.
- Thamer BM, Esmail GA, Al-Dhabi NA, et al. Fabrication of biohybrid electrospun nanofibers for the eradication of wound infection and drug-resistant pathogens. Colloids Surf, A. 2021;609:125691.
- Abdel Bary E, Soliman YA, Fekri A, et al. Aging of novel membranes made of PVA and cellulose nanocrystals extracted from Egyptian rice husk manufactured by compression moulding process. Int J Environ Stud . 2018;75(5):750–762.
- Hong X, Zou L, Zhao J, et al. In dry-wet spinning of PVA fiber with high strength and high young’s modulus. IOP Conf Ser Mater Sci Eng. 2018;439:042011.
- Kebede TG, Dube S, Nindi MM. Fabrication and characterization of electrospun nanofibers from Moringa stenopetala seed protein. Mater Res Express. 2018;5(12):125015.
- Kim SJ, Young Kim T, Kang BH, et al. Fabrication of graphene oxide/montmorillonite nanocomposite flexible thin films with improved gas-barrier properties. RSC Adv. 2018;8(68):39083–39089.
- Marmur A, Della Volpe C, Siboni S, et al. Contact angles and wettability: towards common and accurate terminology. Surf Innovations . 2017;5(1):3–8.